NCBI Bookshelf. A service of the National Library of Medicine, National Institutes of Health.
Institute of Medicine (US) Committee to Review Dietary Reference Intakes for Vitamin D and Calcium; Ross AC, Taylor CL, Yaktine AL, et al., editors. Dietary Reference Intakes for Calcium and Vitamin D. Washington (DC): National Academies Press (US); 2011.
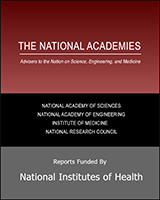
Dietary Reference Intakes for Calcium and Vitamin D.
Show detailsThe last step in the risk assessment process is the step of so-called risk characterization. Its intent is to highlight the nature of the “risks” or public health problems that are relevant to the use of Dietary Reference Intakes (DRIs) and to alert users of the DRI reference values to implications of the assessors' work and to related special issues. This chapter reflects the risk characterization step of the risk assessment approach and is organized to provide: a brief summary of the assessment; discussions about the implications of the committee's work for stakeholders; and discussions to highlight population segments and conditions of interest relative to calcium and vitamin D nutriture.
SUMMARY OF ASSESSMENT
The new DRIs establish, for the first time, an Estimated Average Requirement (EAR) and a Recommended Dietary Allowance (RDA) for calcium and vitamin D. Previously, the DRIs for these nutrients reflected Adequate Intakes (AIs). The ability to set EARs and RDAs rather than AIs enhances the utility of the reference values for national planning and assessment activities. It is important to recognize that these values are intended for the North American population, and also that the requirement for each nutrient is based on the assumption that the requirement for the other nutrient is being met.
Considerable effort was made to ensure that an array of indicators was examined as a possible basis for setting requirements, as well as upper levels of intake. The intent was to fully and objectively examine the scientific basis for the suggested benefit before drawing conclusions. Despite the many claims of benefit surrounding vitamin D in particular, the evidence did not support a basis for a causal relationship between vitamin D and many of the numerous health outcomes purported to be affected by vitamin D intake. Although the current interest in vitamin D as a nutrient with broad and expanded benefits is understandable, it is not supported by the available evidence. The established function of vitamin D remains that of ensuring bone health, for which causal evidence across the life stages exists and has grown since the 1997 DRIs were established (IOM, 1997). The conclusion that there is not sufficient evidence to establish a relationship between vitamin D and health outcomes other than bone health does not mean that future research will not reveal a compelling relationship between vitamin D and another health outcome. The question is open as to whether other relationships may be revealed in the future.
Of great concern recently have been the reports of widespread vitamin D deficiency in the North American population. Based on this committee's work and as discussed below, the concern is not well founded. In fact, the cut-point values used to define deficiency, or as some have suggested, “insufficiency,” have not been established systematically using data from studies of good quality. Nor have values to be used for such determinations been agreed upon by consensus within the scientific community. When higher cut-point values are used compared with those used in the past, they necessarily result in a larger proportion of the population falling below the cut-point value and thereby defined as deficient. This, in turn, leads to higher estimations of the prevalence of deficiency among the population and possibly to unnecessary intervention incorporating high-dose supplementation in the health care of individuals. National survey data suggest that the serum 25-hydroxyvitamin D (25OHD) levels in the North American population generally exceed the levels identified in this report as sufficient for bone health, underscoring the inability to conclude that there are significant levels of deficiency in the population.
Specifically in terms of the new DRIs and challenges for calcium and vitamin D nutriture, several points can be highlighted, within the context of the limitations of estimates of dietary intake, which tend to be under-estimates of actual consumption. First, for calcium, adolescent girls continue to be a group at risk for low intakes from food sources. Older women use calcium supplements in greater proportion, and some may be at risk for excess intake as a result of the use of high-dose supplements. If supplements are needed to ensure adequate calcium intake, it would appear that lower dose supplements should be considered. Many older women have baseline calcium intakes that are close to or just below requirements, and therefore the practice of calcium supplementation at high levels may be unnecessary. This is a special concern for calcium supplement use given the possibility that total intakes (diet plus supplements) above 2,000 mg/ day may increase the risk for kidney stones, and demonstrate no increase in benefits relative to bone health. There is also some limited evidence that the long-term use of calcium supplements may increase the risk for cardiovascular disease. Although no attempt was made to compare systematically the data used for the North American population that is the subject of this report with data from other countries focused on persons who are genetically and environmentally different from those in the United States and Canada, it should be recognized that calcium requirements may be subject to a variety of factors that have not yet been fully elucidated and so therefore cannot yet be integrated into DRI reviews.
For vitamin D, the challenges introduced by issues of sun exposure cannot be ignored. This nutrient is unique in that it functions as a prohormone, and the body has the capacity to synthesize the nutrient if sun exposure is adequate. However, concerns about skin cancer risk preclude making recommendations about sun exposure; in any case, there are a number of unknowns surrounding the effects of sun exposure on vitamin D synthesis. At this time, the only solution when DRIs are to be set for vitamin D is to proceed on the basis of an assumption of minimal sun exposure and set a reference value assuming that all of the vitamin D must come from the diet. Moreover, the possibility of risk for persons typically of concern because of reduced synthesis of vitamin D, such as persons with dark skin or older persons in institutions, is minimized given the assumption of minimal sun exposure for the DRIs.
One unknown in the process of DRI development for vitamin D is the degree to which waning kidney function with aging may be relevant. It appears that increasing serum 25OHD levels do not typically increase calcitriol levels in aging persons with mild renal insufficiency, and a dietary strategy to address the concern is not evident.
Although ensuring adequacy is important, there is now an emerging issue of excess vitamin D intakes. A congruence of diverse data on health outcomes ranging from all-cause mortality to cardiovascular risk suggests that adverse health outcomes may be associated with vitamin D intakes that are much lower than those classically associated with hypervitaminosis D and that appear to occur at serum 25OHD levels achievable through current levels of supplement use.
IMPLICATIONS
The extensive review of the data required to conduct this study and to determine DRIs for calcium and vitamin D that are consistent with existing scientific understandings has answered many questions. But, the process has also identified or left unanswered other questions due to the limitations of the available evidence. Because uncertainties exist in the knowledge base related to the role of vitamin D and calcium in health outcomes, it is important to acknowledge that there are uncertainties surrounding these reference values for calcium and vitamin D. The development of any reference value should be viewed as a work in progress, which may be subject to change if there are significant changes in the science base.
Further, an important aspect of DRI development is its grounding in public health applications and the concept of distributions of risk. This approach may appear strange to some and may be disconcerting to those with a clinical orientation who are familiar with the medical model in which the goal is to treat the patient in the most efficacious manner to enhance a positive outcome. The interpretation and use of data in the case of DRI development are within the context of the relevant probability distributions of risk; the DRI task focuses on median requirements and the description of risk, whereas the medical model is based on maximizing effects that ensure beneficial outcomes for all persons. This report, therefore in contrast to a medical model approach, determines dose–response relationships by assessing the level at which 50 percent of the population's needs are met (the EAR) and the level at which approximately 97.5 percent of the population are likely to have their needs met (the RDA). The distribution of dose–response effects is highly relevant to DRI development, compared with information about a maximizing effect for benefit. A difficulty the committee too often faced was studies that included only a placebo or baseline low dose coupled with a relatively large, single supplemental dose, as these are relatively uninformative for DRI development.
Discussions below call attention to the uncertainties surrounding the DRI values for calcium and vitamin D and also highlight important conclusions that stem from the process of developing these DRIs. In addition, given that this report is the first effort to develop DRIs since the 2007 IOM workshop that explored lessons learned and new challenges and outlined the risk assessment approach for DRI development (IOM, 2008; Taylor, 2008), comments are offered about the process. Specific research recommendations for the future development of DRIs related to calcium and vitamin D are presented in Chapter 9.
Assumption of Minimal Sun Exposure
The committee's assumption of minimal sun exposure is a markedly cautious approach given that the vast majority of North Americans appear to obtain at least some vitamin D from inadvertent or deliberate sun exposure. Currently, there is a lack of information about whether certain levels of sun exposure may be experienced without increased risk of cancer and whether such exposure would be consistent with a contribution of vitamin D useful to the body. Therefore, at this time, recommendations concerning sun exposure relative to vitamin D requirements cannot and should not be offered; there are no options other than to base dietary recommendations on the assumption of minimal sun exposure. The evidence to indicate that the synthesis of vitamin D from sun exposure is subject to a feedback loop that precludes toxicity from sun exposure is reassuring and, when coupled with the checks and balances introduced into the DRI development process, makes it very unlikely that consumption of the DRI levels of vitamin D, even if combined with high levels of sun exposure, will be problematic to the general population.
However, given that many North Americans appear to obtain at least some vitamin D from inadvertent or deliberate sun exposure, there are implications for the interpretation of intake levels of the vitamin. In short, the intake data for vitamin D cannot stand alone as a basis for public health action on a national population level. Such considerations are consistent with the 2000 IOM report on applications of DRIs in dietary assessment (IOM, 2000), which states: “Whenever possible, the assessment of apparent dietary adequacy should consider biological parameters such as anthropometry, … biochemical indices, … diagnoses, … clinical status, and other factors as well as diet. Dietary adequacy should be assessed and diet plans formulated based on the totality of the evidence, not on dietary intake data alone.” In short, for policy making and decisions about the adequacy of the food supply for the general population at the national level, vitamin D must be considered in the context of measures of serum 25OHD, an established biomarker of exposure from endogenous synthesis as well as diet, including supplements. Although the reported estimates of vitamin D intake appear to be less than needed to meet requirements, the serum 25OHD data available—when coupled with the committee's assessment of serum 25OHD levels consistent with EAR and RDA values—suggest that average requirements are being met for the DRI age groups nationally in both countries. That is, although mean total intakes of vitamin D generally are lower than the estimated median requirement (the EAR), the available clinical measures do not suggest widespread deficiency states. This underscores the possibility that sun exposure is contributing generally to the maintenance of adequate serum 25OHD concentrations.
Uncertainties
As discussed in the preceding chapters, there are limited data for many topics of interest in setting DRI values for calcium and vitamin D. Overall, the uncertainties surrounding the DRI values for calcium are less than those for vitamin D, because the evidence base is considerably larger for calcium, and the physiology and metabolism of calcium are better understood. The following key issues were identified by the committee as introducing uncertainty into the DRI values for calcium and vitamin D, as based on bone health outcomes:
- The tendency for study protocols to administer a combination of calcium and vitamin D, reducing the opportunity to ascertain the effects of each nutrient independently;
- The lack of data examining the responses and health outcomes due to graded doses of calcium or vitamin D intake so as to elucidate dose–response relationships;
- The interaction between calcium and vitamin D to the extent that it would appear that adequate calcium intake greatly diminishes the need for vitamin D relative to bone health outcomes;
- The unique situation in which a nutrient (vitamin D) physiologically serves as a prohormone introduced a myriad of variables and feedback loops related to its health effects;
- The paucity of data and resulting uncertainty concerning sun exposure that confound interpretation of the dose–response relationship between intakes of vitamin D and various health outcomes. This, coupled with the apparent contribution of sun exposure to overall vitamin D nutriture in North American populations, leads to an inability to characterize and integrate sun exposure with intake recommendations as much as may be appropriate, given the concern for skin cancer risk reduction, which must be paramount. Thus, for individuals who do not follow recommendations to avoid sun exposure, the uncertainty of the DRI values is greater than for those who do;
- The lack of clarity concerning the validity of the serum 25OHD measure as a biomarker of effect;
- The variability surrounding measures of serum 25OHD concentrations as a result of different methodologies used;
- A number of findings suggesting a strong role for metabolic adaptations and controls in the case of vitamin D, which complicates estimations of nutrient requirements. These include: the non-linear response of serum 25OHD level to vitamin D intake, which, in turn, suggests that it requires proportionately more vitamin D to continue to increase serum 25OHD levels after a certain serum 25OHD level is reached; the observation that seasonal declines in serum 25OHD level are greater if a person begins the winter season with a higher compared with a lower serum 25OHD level; and the lack of effect of age and body size (other than adiposity) on serum 25OHD levels;
- The limited number of long-term clinical trials related to calcium and vitamin D intakes and health outcomes; and
- The need to set ULs based on limited data in order to ensure public health protection.
An important question that will undoubtedly be asked given this committee's report, is: Why is it that so much information about the positive effects of vitamin D on outcomes such as cancer, diabetes, and immunity is said to exist and is reported almost daily in the press, but this committee found no basis to support these causal relationships? The short answer is that a systematic examination of the evidence, using established guidelines for measuring the strength and quality of studies, revealed that the claimed benefits based on the associations of low or high intakes of vitamin D on non-skeletal health outcomes could not be supported by the studies— the evidence was inconsistent and/or conflicting or did not demonstrate causality. In addition, some effects were not related to setting nutritional requirements for vitamin D. This conclusion, however, does not preclude pursuing investigation of causal relationships.
Moreover, a related question that will be asked is: With the advent of newer studies, why is there still so much uncertainty? At least one reason is that most studies were not designed to seek data maximally useful for DRI development, which is well described by others (Yetley et al., 2009). DRI development fundamentally requires elucidation of dose–response relationships and benefits from data of high quality obtained in randomized controlled trials. In making its conclusions about potential indicators other than bone health, the committee noted the findings previously specified by an IOM committee tasked with examining the evolution of evidence for nutrient and disease relationships (IOM, 2002). That committee concluded that evidence about relationships between specific nutrients and a disease or health outcome typically remains elusive for a number of reasons (IOM, 2002). These include the following:
- Although preliminary evidence, usually from mechanistic studies, experimental animal studies, and observational studies in humans, can generate exciting new hypotheses about nutrient–health relationships, evidence from these studies has limitations. For instance, even in well-designed, large-scale observational studies, it is difficult to isolate the effects of a single nutrient under investigation from the confounding effects of other nutrients and from non-nutrient factors.
- Scientific advances in understanding relationships between specific nutrients and health outcomes do not necessarily emerge within a short time, and progress is often erratic. Some gaps are filled, while others are created.
- The etiology of disease–health relationships, especially in the case of chronic disease, is commonly multi-factorial. Even if diet has a prominent role, it is extremely unlikely that a single nutrient is directly responsible for a chronic disease or, conversely, that addition of a single nutrient will eliminate disease risk. It is possible that a focus on specific nutrients as risk factors for diseases in relatively homogeneous or diseased populations can lead to a number of spurious associations.
- Clinical trials, which are generally considered to provide the strongest evidence about the effects of nutrient intake on subsequent disease and health, are complex, expensive, and time-consuming, especially for chronic diseases that develop over decades and are influenced by a host of genetic, physiological, and environmental factors that may also affect risk.
The committee found all of the above findings to be the case for non-skeletal health outcomes for vitamin D, as the discussions of the strength, consistency, and causality of the evidence demonstrate in Chapter 4.
Finally, an important uncertainty focuses on the issue of excess intake. This is particularly true for vitamin D, which has been hypothesized to confer health benefits at relatively high levels of intake. Although the committee's decisions for the ULs made use of emerging data concerning a U-shaped (or perhaps reverse-J-shaped) curve for risk, which suggested adverse effects at levels much lower than those associated with hypervitaminosis D, the lack of data on the safety of higher intakes of vitamin D when used chronically is very concerning. Byers (2010), in a recent editorial commenting on the outcomes of a pooling study focused on vitamin D and six types of cancer in which the only association observed was a doubling of the risk for pancreatic cancer for those in the highest quintile of circulating serum 25OHD levels, offered the following observation: “We have learned some hard lessons…. and we now know that taking vitamins in supernutritional doses can cause serious harm.”
Conclusions About Vitamin D Deficiency in the United States and Canada
Serum 25OHD levels have been used as a “measure of adequacy” for vitamin D, as they reflect intake from the diet coupled with the amount contributed by cutaneous synthesis. The cut-point levels of serum 25OHD intended to specify deficiency and sufficiency for the purposes of interpreting laboratory analyses and for use in clinical practice are not specifically within the charge to this committee. However, the committee notes with some concern that serum 25OHD cut-points defined as indicative of deficiency (or as reported by some, “insufficient”) for vitamin D have been subject to a wide variation in specification without a systematic, evidence-based consensus development process. In order to ensure clarity, the discussion in this section expresses serum 25OHD levels in both nmol/L and ng/mL measures.
From this committee's perspective, a considerable over-estimation of the levels of vitamin D deficiency in the North American population now exists due to the use by some of cut-points for serum 25OHD levels that greatly exceed the levels identified in this report as consistent with the available data. The 1997 IOM report (IOM, 1997) specified a serum 25OHD concentration of 27.5 nmol/L (11 ng/mL) and above as an indicator of vitamin D adequacy from birth through 18 years of age, and a concentration of 30 nmol/L (12 ng/mL) and above as an indicator of vitamin D adequacy for adults. This level (27.5 nmol/L for children, and 30.0 nmol/L for adults) remains a level below which frank deficiency including rickets and osteomalacia may be expected to occur. In recent years, others have suggested different cut-point values as determinants of deficiency (or “insufficiency”). These include values ranging from less than 50 nmol/L (20 ng/ mL) to values above 125 nmol/L (50 ng/mL). Based on this committee's deliberations, the vitamin D–related bone health needs of approximately one-half of the population may be expected to be met at serum 25OHD concentrations between 30 and 40 nmol/L (12 and 16 ng/mL); most of the remaining members of the population are likely to have vitamin D needs met when serum concentrations between 40 and 50 nmol/L (16 and 20 ng/mL) are achieved. Failure to achieve such serum concentrations place persons at greater risk for less than desirable bone health as manifested by, depending upon age, increased rates of bone accretion, bone mineral density, and fractures.
Use of higher than appropriate cut-points for serum 25OHD levels would be expected to artificially increase the estimates of the prevalence of vitamin D deficiency. The specification of cut-point values for serum 25OHD levels has serious ramifications not only for the conclusions about vitamin D nutriture and nutrition public policy, but also for clinical practice. At this time, there is no central body that is responsible for establishing such values for clinical use. This committee's review of data suggests that persons are at risk of deficiency at serum 25OHD levels of below 30 nmol/L (12 ng/mL). Some, but not all, persons are potentially at risk for inadequacy at serum 25OHD levels from 30 up to 50 nmol/L (12 to < 20 ng/mL). Practically all persons are sufficient at levels of 50 nmol/L (20 ng/mL) and above. Serum concentrations of 25OHD above 75 nmol/L (30 ng/mL) are not associated with increased benefit. There may be reason for concern at serum 25OHD levels above 125 nmol/L (50 ng/mL). Given the concern about high serum 25OHD levels as well as the desirability of avoiding mis-classification of vitamin D deficiency, there is a critical public health and clinical practice need for consensus cut-points for serum 25OHD. The current lack of evidence-based consensus guidelines is problematic and of concern because individuals with levels of 25OHD serum above 50 nmol/L (20 ng/mL) may at times be diagnosed as deficient and treated with high-dose supplements of vitamin D containing many times the levels of intake outlined in this report.
Decisions Regarding Levels of Calcium and Vitamin D to Be Administered in Controlled Clinical Trials
Although this report identifies upper levels of intake below which adverse effects are not expected to arise, ULs are intended to serve as a lifetime public health measure for a free-living, unmonitored population. Those responsible for determining the appropriate dosages of nutrients to be studied in carefully controlled experimental trials conducted with appropriate adverse event and safety monitoring have the opportunity to bring other considerations into play when deciding on the levels of nutrients that are acceptable and appropriate for subjects taking part and being monitored in such studies. Research using intakes higher than those specified in the ULs can be justified under a number of circumstances after careful review of the literature and through the use of appropriate study protocols. Indeed, such studies are likely to be informative to the understanding of dose–response relationships and the health benefits or risks associated with calcium and vitamin D intakes.
The DRI Development Process
As described in Chapter 1, the DRI development process has recently been subjected to a review as well as targeted discussions about the process and ways to enhance it (IOM, 2008). As an overall result of these discussions, DRI development is now placed more clearly in the context of the risk assessment approach—that is, an organizing framework for conducting evaluations with public health implications often made with evidentiary uncertainties. There is also a series of existing “gap issues”—specifically, needed methodologies and guidelines—that have been identified as important to improving and enhancing the process for developing DRIs and would benefit from targeted efforts to resolve the gaps (Taylor, 2008).
The report of this committee is the first DRI report to be completed subsequent to the 2004 to 2008 evaluation of the DRI development process. It has been structured to be consistent with the risk assessment process with the intent of enhancing its transparency, especially in the face of uncertainties. Although this committee was mindful of the identified methodological gaps for enhancing the DRI process, it was not tasked with addressing them; in any case, virtually all of the relevant issues are complex and suggest a need to convene groups of individuals with specific expertise germane to the question at hand. Because this DRI report is an initial effort to set DRI development on the path of a risk assessment approach, its experience points to the importance of addressing several gap issues.
Specifically:
- The identification of dose–response relationships for calcium and vitamin D relative to health outcomes was a major challenge. The gap issue1 (number 5-5 in Taylor, 2008) that is focused on methodologies for approximating dose–response relationships warrants attention, as it is likely that DRI efforts in the future will face the same challenges.
- With the exception of the inclusion of osteoporosis within the bone health measures, the existing data precluded the use of a chronic disease such as cancer or heart disease as an indicator for DRI development. However, had it been possible, this DRI process would have benefited from guidelines specifying what, if any, differences may apply to using chronic disease endpoints versus other types of endpoints for DRI development (gap issue number 4-42 in Taylor, 2008).
- In the committee's judgment, sufficient new data were available to allow the development of EARs and RDAs, and it was no longer necessary to make use of AI estimates for calcium and vitamin D, except for infants. The AI is useful in that it allows the specification of some type of a reference value for use in public health settings—which is better than the absence of any value. However, it presents challenges in public health applications (gap issue number 4-23 in Taylor, 2008), because it is not entirely consistent with the statistical approach based on distributions of requirements that underpin the DRIs. Guidelines for the use of AIs would be helpful, both those that exist for other nutrients at this time as well as those that might be specified in the future.
POPULATION SEGMENTS AND CONDITIONS OF INTEREST
Adiposity
As highlighted in Chapter 3, excess adiposity or obesity—defined as a body mass index (BMI) measure of 30 mg/m2 or higher—is associated with lower serum 25OHD concentrations (and higher parathyroid hormone levels) than found in non-obese counterparts. This would appear to be due to sequestration of 25OHD by adipose tissue, given that supplementation of obese and lean persons with vitamin D appears to result in no significant difference in response between the two groups (Jones, 2008). Moreover, a few studies of modest weight loss have found circulating 25OHD levels to increase despite no increased intake of vitamin D from diet or sun exposure (Riedt et al., 2005; Reinehr et al., 2007; Zittermann et al., 2009; Tzotzas et al., 2010), suggesting release from adipose stores with adipose depletion. Further, neither season nor ethnicity influences these biochemical parameters (Alemzadeh et al., 2008).
An important concern is whether the lower serum 25OHD levels associated with obesity have meaningful consequences for the DRI indicator of bone health. Evidence for effects of obesity on bone density is mixed. The combined influence of increased weight-bearing activity and endogenous synthesis of estrogen due to outcomes of increased adiposity has long been associated with higher bone density (Reid, 2008). In a population-based study in Finland of perimenopausal and early postmenopausal women, Pesonen et al. (2005) found that increased body weight was a strong predictor of high bone density. Likewise, Morin and Leslie (2009), in a retrospective cohort study, found a strong correlation between higher BMI category and high bone density in postmenopausal women.
Although these and other studies have suggested that total body mass contributes to bone density and would appear to support the role of increased weight-bearing activity as a factor positively influencing bone density (Prentice et al., 1991; Khosla et al., 1996; Wortsman et al., 2000; Finkelstein et al., 2002, 2008), more recent studies lead to further questions. The distribution of body fat may influence bone mass, such that excess intra-abdominal fat could adversely affect bone remodeling and even contribute to greater fracture risk (Premaor et al., 2010; Sukumar et al., 2011). One possibility is that intra-abdominal adipose tissue is more biologically active than subcutaneous fat, secreting cytokines and adipokines that negatively affect osteoblast and osteoclast activity (Kawai and Rosen, 2010). Moreover, both lean and fat mass contribute to weight-bearing effects. Because obesity is accompanied by increases in both lean mass and fat mass, at least in younger individuals, it is difficult to attribute the effect on bone density to fat mass as opposed to lean mass. Further, body composition changes with age, even in the obese; in turn, there may be less lean body mass in older individuals.
This complicates the ability to clarify how adiposity may affect bone health. As noted, some studies have suggested that adiposity or increased fat mass itself may be a factor in the development rather than the prevention of osteoporosis, particularly in the elderly. Zhao et al. (2007) observed that when the effect of mechanical loading from high body weight on bone density was statistically controlled, fat mass was inversely correlated with bone mineral content. Further investigation by Zhao et al. (2008) suggested that molecular signaling pathways involved in osteoblast differentiation may contribute to the previously identified effect of increased adiposity on decreased bone mineral content, although a mechanism has not been elucidated. However, this science is just emerging and it is premature to speculate on its significance or relevance to bone health and bone density.
At this time, there is the possibility that obesity, at least in older persons, may not be beneficial for bone health and may be demonstrated to be a risk factor, not an advantage, for decreased bone density and, in turn, reduced bone health. There is no evidence that increases in calcium or vitamin D nutriture beyond the requirements specified for non-obese persons can affect this purported outcome.
Persons Living at Upper Latitudes in North America
The question of the impact of latitude on vitamin D nutriture is often a topic of concern or, at least, interest. The issue, however, is set in the context of the inability to specify a safe dose of sunlight that could contribute to vitamin D synthesis while also avoiding the risk of skin cancer. There are also the recognized challenges associated with quantifying the contributions from sun exposure coupled with the limited information on the role of stored vitamin D during seasonal changes. The prevailing assumption about the effect of latitude is that ultraviolet B (UVB) penetration decreases with increasing latitude (i.e., distance from the equator) and this, in turn, causes persons living at higher latitudes in North America to experience little or no UVB exposure, making them at risk for vitamin D deficiency. This assumption may not be entirely accurate. However, the question of latitude may work in tandem with other factors, discussed below, such as limited sun exposure overall or cultural and dietary practices. This section focuses only on the issue of latitude per se.
The relationship between UVB penetration and latitude is complex and not merely a function of distance from the equator. Other factors that come into play include the reduced atmosphere at the poles (about 50 percent less than at the equator), more cloud cover at the equator than at the poles, differences in ozone cover, and the duration of sunlight in summer versus winter. Geophysical surveys have indicated that UVB penetration over 24 hours during the summer months at Canadian north latitudes equals or exceeds UVB penetration at the equator (Lubin et al., 1998), suggesting that persons living in the northern latitudes are not necessarily receiving notably less total sunlight during the year. Rather, it suggests that there may be considerable opportunity during the spring, summer, and fall months in the far north for humans to form vitamin D and store it in liver and fat. Likewise, animals living in the same region that are consumed as part of the traditional diet are also rich sources of vitamin D (Keiver et al., 1988; Kenny et al., 2004; Brunborg et al., 2006; Kuhnlein et al., 2006).
These factors help to explain why latitude alone does not appear to predict serum 25OHD concentrations in humans. In a Finnish study, healthy subjects living above the Arctic Circle (latitude 66°N) did not have lower serum 25OHD levels than subjects living in southern Finland; in fact, the group living above the Arctic Circle had higher levels. Both groups achieved mean serum 25OHD levels above 90 nmol/L during the summer, whereas the mean serum 25OHD level at the winter nadir was 56 nmol/L in the south and 68 nmol/L in those living above the Arctic Circle (Lamberg-Allardt et al., 1983).
Persons Experiencing Reduced Vitamin D Synthesis from Sun Exposure
The DRIs for vitamin D established in this report are based on the assumption of minimal sun exposure. Therefore, they are regarded as adequate for persons who may be experiencing a reduced synthesis of vitamin D from sun exposure. Assuming that some population groups may be consuming less than the current DRI values for vitamin D, the question is to what extent are these persons at risk for vitamin D deficiency, or, conversely, to what extent can inadvertent sun exposure be expected to compensate for lower intakes for these persons?
Dark Skin
As described in Chapter 3, skin pigmentation—due to melanin in the epidermal layer—can reduce the amount of vitamin D synthesized by the human body. The amount of UVB required for changes in serum 25OHD levels is partly related to the degree of skin pigmentation. Further, a number of reports through the years have indicated consistently lower serum 25OHD levels in persons identified as black compared with those identified as white (Specker et al., 1985; Harkness and Cromer, 2005; Stein et al., 2006; Armas et al., 2007; Basile et al., 2007; Bodnar et al., 2007). Looker et al. (2008), using the National Health and Nutrition Examination Surveys (NHANES) 2000 to 2004, reported lower serum 25OHD levels for non-Hispanic blacks compared with Mexican Americans and whites. Mexican Americans had serum 25OHD concentrations that were intermediate between those of non-Hispanic blacks and whites.
The question is whether the consistently lower levels of serum 25OHD for persons with dark skin pigmentation have significant health consequences. Based on the data of Looker et al. (2008), non-Hispanic blacks in the NHANES had an average serum 25OHD concentration of 40.14 nmol/L (± 0.88 nmol/L [standard error of the mean]). Given that 40 nmol/L may be reflective of an acceptable median level for serum 25OHD in serum based on this committee's work, it is difficult to suggest that this average serum 25OHD level is indicative of widespread deficiency, although such conclusions cannot be based solely on mean values. However, at least for those of African American ancestry, there are corollary data to suggest that rates of osteoporosis and bone disease are not higher among African Americans; in fact, African Americans have reduced rates of fracture and osteoporosis compared with whites (see Chapter 4). There are no data in this regard for other ethnic groups with dark skin, such as South Asians, so firm conclusions about their risk related to bone health cannot be drawn. Furthermore, it is possible that risk may be introduced or modulated by an array of variables, including cultural and ethnic practices.
Given the unknowns, dark-skinned immigrant groups who now reside in North America may present a concern, as described below. There is also a concern for dark-skinned infants and children whose overall diet may be low in calcium and who may have low serum 25OHD levels, especially if exclusively breast-fed and not otherwise supplemented (see below). The vitamin D and calcium issues related specifically to African Americans have been described earlier in Chapter 4.
South Asian and Middle Eastern immigrant groups South Asians (e.g., Indians, Pakistanis, Sri Lankans) are now residing in greater numbers in North America, and are reported to be at increased risk for vitamin D–deficiency. This group has a significant presence in Canada and is growing in number (Statistics Canada, 2010). A recent study by Wu et al. (2009) measured vitamin D intakes and serum 25OHD levels in three different ethnic groups in southern Ontario and found that levels were significantly lower in South Asians than in Eastern Asian or European groups. Over the past few decades, there have been sporadic reports of vitamin D–deficiency rickets in Canadians, almost always in breast-fed, dark-skinned Canadians of African or Asian descent, but the total number of cases, even in a major metropolitan area like Toronto, is small (17 over a 5-year period from 1988 to 1993) (Binet and Kooh, 1996). Similar to the situation in African Americans (see Chapter 3), the lower serum 25OHD levels observed are not associated with significant rises in the rates of bone disease (osteomalacia or rickets) in the Canadian South Asian cohort. In other South Asian communities living at relatively high latitudes (> 50°N) in Europe (e.g., Scotland), there have been reports of rickets and osteomalacia dating back to the early 1970s (Ford et al., 1976; Goel et al., 1976) and suggestions that vitamin D deficiency might also be associated with higher rates of tuberculosis (Yesudian et al., 2008). Although ensuring that the DRIs are met for these groups should reduce the risk for deficiency states to the extent that their conditions mimic those from minimal sun exposure, it is considered advisable to exercise vigilance for this growing group.
Some immigrant populations or religious groups adhere to cultural practices regarding clothing that can greatly reduce exposure to sun light and exacerbate the effects of low intake of vitamin D. There is the suggestion that at least 20 percent of the body's surface must be exposed to UVB for serum 25OHD levels to increase (Specker et al., 1985; Hollis, 2005). Whether such sun exposure is a wise public health practice for any group is not the issue, only that there is a need for awareness when such cultural practices limit sun exposure.
Dark-skinned, exclusively breast-fed infants In 2000, a report was published concerning rickets among nine children from various areas of the United States (Shah et al., 2000). Eight children were described as African American, and one child was described as Hispanic. All patients were primarily breast-fed for more than 11 months, with minimal intake of dairy products and without vitamin D supplementation. Breast milk, is of course, not a source of vitamin D for infants. This report had been preceded by a 1979 report from Bachrach et al. (1979), who noted 24 cases of vitamin D–deficiency rickets in black, breast-fed infants who were otherwise healthy and had no underlying malabsorptive or renal diseases, but whose parents belonged to groups that subscribed to dietary restrictions and clothing habits that minimized their exposure to sunlight. Later, a 2001 report described a black infant who was breast-fed until 10 months of age and then weaned to a soy food beverage that was not fortified with vitamin D or calcium (Carvalho et al., 2001). The infant developed normally until about 9 months of age when the child's height and weight became severely arrested. In 2003, DeLucia et al. (2003) commented on 43 children with nutritional rickets reported from 1986 through 2002 and located in the New Haven, Connecticut area. Approximately 86 percent were of African American, Hispanic, or Middle Eastern descent. More than 93 percent of the children had been breast-fed. In this case, the authors implicated both low calcium intake as well as marginal vitamin D nutriture in rickets.
A recent 2-year survey of Canadian pediatricians found the incidence of rickets in their patients to be 2.9 per 100,000; the mean age at diagnosis was 1.4 years (range of 2 weeks to 6.3 years). Ninety-four percent of the children with rickets had been breast-fed. Additional risk factors included dark skin, living in the far north, born of mother who took no vitamin supplements, limited sun exposure, emigrated from a region where vitamin D deficiency is endemic, and delayed initiation of solid foods (Ward et al., 2007).
Vitamin D supplementation of partially or fully breast-fed infants should begin in the first week of life and provide approximately 400 IU/day, as breast milk is not a source of this nutrient for infants, and sun exposure to compensate for this cannot be adequately described but neither can it be recommended given the concerns for skin cancer. It is important to be especially vigilant regarding supplementation in the case of exclusively breast-fed, dark-skinned infants, as they appear to be at higher risk than lighter-skinned infants.
Use of Sunscreen
Sunscreen absorbs ultraviolet light and prevents it from reaching the skin. It has been reported that sunscreen with a sun protection factor (SPF) of 8 based on the UVB spectrum can decrease vitamin D synthetic capacity by 95 percent, whereas sunscreen with an SPF of 15 can reduce synthetic capacity by 98 percent (Matsuoka et al., 1987). The extent and frequency of use of sunscreen are unknown, and therefore the significance of the role that sunscreen may play in reducing the opportunity to synthesize vitamin D is unclear. Increases in serum 25OHD levels seen in summer months in national surveys conducted in both the United States and Canada would suggest either that sunscreen is not used consistently by the population as a whole or that the actual decrease in serum 25OHD level due to appropriate use of sunscreen has been overstated. Although inconsistent with advice provided by the American Academy of Dermatology4 and the National Council on Skin Cancer Prevention5 for skin cancer protection, given the carcinogenic potential of UVB light, one report indicated that there is adequate vitamin D production when exposure of hands, face, arms and legs to sunlight is for an amount of time equal to about 25 percent of what it would take to develop a “mild sunburn”; after this extent of exposure, a sunscreen should be applied to prevent damage (Holick, 2003). However, this is in contrast to a recent report on mathematical models of observational data regarding the impact of seasonal sun exposure (Diffey, 2010). The effect of the use of sunscreen, as with other factors that may limit exposure, warrants vigilance. However, its use should not constitute a concern, given that the DRI values assume minimal sun exposure.
Indoor Environments and Institutionalized Older Persons
Increased urbanization and the normative condition among North Americans to work and recreate indoors cannot be quantified or addressed in terms of increased risk for vitamin D deficiency. The newly established DRI values assume minimal sun exposure, and therefore vitamin D intake need not be increased above this level for normal persons living in urban settings and spending time primarily indoors.
However, data for institutionalized, frail older persons suggest a propensity for lower serum 25OHD levels generally. Causation, however, is uncertain. It is likely that many factors contribute, such as their restriction to primarily indoor environments often coupled with inadequate total intake overall. Further, aging skin is known to be less effective in synthesizing vitamin D in part because of a decrease in skin provitamin D (7-dehydrocholesterol) levels and in part because of alterations in skin morphology (MacLaughlin and Holick, 1985). The EAR and RDA values have taken this group into consideration to the extent possible and allowed by the data. Given the unknowns, however, monitoring institutionalized elderly people for vitamin D (and calcium) nutriture is appropriate. Supplementation, however, should not be random and without cause, because excess intakes of these nutrients may have adverse consequences for this frail sub-population.
Alternative Diets or Changes in Dietary Patterns
Dairy and Animal Product Exclusion: Lactose Intolerance, Cow's Milk Food Allergy, Ovo-Vegetarianism, and Veganism
Exclusion of dairy products occurs therapeutically in those with lactose intolerance or cow's milk food allergy, and voluntarily in those who are vegans or non-lacto vegetarians. As noted in the recent National Institutes of Health (NIH) Consensus Statement on Lactose Intolerance (Brannon et al., 2010; Suchy et al., 2010), exclusion of dairy products, all of which are rich sources of calcium and some of which are fortified with vitamin D (e.g., fluid milks, some yogurts, and limited other dairy products [Yetley, 2008]), can be a risk factor for inadequate intakes of calcium and vitamin D. This is also true for vegans (Craig, 2009) and likely others who systematically exclude dairy foods as well as other animal products from their diets. However, as pointed out by the American Dietetic Association (American Dietetic Association and Dieticians of Canada, 2003; Craig and Mangels, 2009) as well as the Dietitians of Canada (American Dietetic Association and Dietitians of Canada, 2003), appropriately planned vegetarian diets, including total vegetarian or vegan diets, are healthful and nutritionally adequate.
The North American prevalence of lactose intolerance, a clinical syndrome characterized by diarrhea, bloating and/or flatulence following consumption of lactose, is challenging to determine because the parameters surrounding lactose intolerance, lactose malabsorption, and lactase non-persistence are not well defined, and frequent self-diagnosis occurs (Brannon et al., 2010; Suchy et al., 2010). The prevalence of cow's milk allergy reported in a systematic evidence review (Rona et al., 2007) was 0.6 to 0.9 percent by skin test, specific immunoglobulin E measurement, or food challenge test; this is lower than the self-reported prevalence of 3 percent. Similarly to lactose intolerance, individuals may perceive that they have cow's milk food allergy when they do not. With respect to vegetarians, in 2006, approximately 1.4 percent of U.S. adults and nearly 1 percent of children and adolescents 8 to 18 years of age self-reported that they were vegans, and 2.3 to 3 percent reported themselves to be vegetarians.6 In a 2002 survey, about 4 percent of Canadian adults reported being vegetarians (American Dietetic Association and Dietitians of Canada, 2003). Although there are few data to document the consequences of poorly planned diets that exclude dairy or animal products—it is noted that Craig (2009) reported a 30 percent increased risk of fracture for vegans—it is best to assume that persons who have chosen or must follow such diets should make special efforts to ensure nutritional adequacy.
Strategies for ensuring adequate intakes of calcium and vitamin D vary depending on the reason for dietary exclusion. Using an Agency for Healthcare Research and Quality systematic evidence review as a basis (Shaukat et al., 2010; Wilt et al., 2010), an NIH Consensus Panel found that individuals with lactose intolerance or lactose malabsorption are able to tolerate up to 12 g of lactose, the equivalent of one cup of milk, in a single dose and may be able to tolerate larger amounts if consumed in smaller doses spread over the day and with other foods. Larger amounts of reduced-lactose dairy products such as certain yogurts and fluid milks as well as virtually unrestricted amounts of reduced-fat hard cheeses with very low amounts of lactose may be ingested to ensure adequate intakes of calcium. For those who avoid all dairy because of allergies or personal choice, consumption of non-dairy sources of calcium, such as low-oxalate vegetables (e.g., kale, bok choy, Chinese cabbage, broccoli, and collards), calcium-containing tofu, or fortified plant-based foods, such as cereals or fruit juice are feasible strategies to ensure adequate intakes of highly bioavailable calcium (Weaver et al., 1999). Finally, supplements of calcium are also a strategy, although care should be taken not to over-supplement.
Meeting vitamin D needs is more challenging in the absence of sun exposure. Plant foods are not natural sources of vitamin D,7 but the marketplace in the United States is increasingly offering plant-based fortified alternatives such as cereals and juices. In addition, the Canadian food supply includes margarines fortified with vitamin D and plant-based beverages that are fortified with vitamin D and calcium. Such fortified foods can be helpful in meeting the DRIs across age groups. As with calcium, a dietary supplement of vitamin D is also an option, but total intake (foods plus supplements) should not exceed the Tolerable Upper Intake Level (UL).
Changes in Dietary Patterns of Indigenous Canadian Populations
Among the indigenous Canadian populations, switching from a traditional diet that contains vitamin D–rich foods to a westernized diet may increase the likelihood of vitamin D deficiency, especially if UVB exposure is limited or avoided. This has been underscored by a survey of Inuit living in Greenland, which reported that those consuming a westernized diet had lower serum 25OHD levels than those consuming a traditional diet (32 vs. 53 nmol/L in summer, 29 vs. 41 nmol/L in winter) (Rejnmark et al., 2004). As noted above, there is ample opportunity during the spring, summer, and fall months in the far north for animals that commonly comprise the traditional diet of indigenous groups to form vitamin D and store it in liver and fat. In turn, the blubber and liver of various arctic marine mammals (e.g., seal, narwhal, beluga, walrus) and fish (e.g., char, cisco, lake trout, loche, sculpin, whitefish) are sources of vitamin D for those who consume a traditional diet (Keiver et al., 1988; Kenny et al., 2004; Brunborg et al., 2006; Kuhnlein et al., 2006).
The Canadian Health Measures Survey does not collect data on these indigenous populations living at upper northern latitudes, and overall dietary and health data for them are limited. One recent survey (Kuhnlein et al., 2008) in northern Canada found that the intakes of vitamin D differed by ethnic group. The median vitamin D intake was 200 IU/day in both Yukon First Nations8 and Dene/Métis. However, much higher median intakes were found in older (over age 40) Inuit who consumed a traditional diet (1,000 IU/day and 680 IU/day in men and women, respectively), whereas younger Inuit had much lower intakes (328 IU/day and 372 IU/day in men and women, respectively). This research group also surveyed indigenous women of reproductive age from various communities in the Canadian Arctic and found the mean daily intakes of vitamin D to be 456 IU/day in Inuit from Qikiqtarjuaq, 364 IU/day in Inuit from 18 other communities, and 228 IU/day in a combined data set of Dene, Métis, and Yukon First Nations. Pregnant and lactating women had higher vitamin D intakes, with the highest mean intake being 816 IU/day in lactating Inuit from Qikiqtarjuaq (Berti et al., 2008). Neither of these surveys measured serum 25OHD levels.
A 1999 survey (Smith, 1999) estimated vitamin D intakes and measured serum 25OHD levels in 121 pregnant women living in the Inuvik region of the Northwest Territories. The sample included 33 whites, 51 Inuit, and 37 First Nations people. The investigator did not report whether the First Nations and Inuit mothers were consuming a traditional or a western diet; moreover, the accuracy for the measures of the vitamin D content of traditional foods is unclear. The estimated daily mean vitamin D intake of Inuit and First Nations people was 324 IU/day with supplements (136 IU/ day without) compared with 532 IU with supplements (232 IU without) to whites. At the point of delivery, the plasma levels of 25OHD were lower in the First Nations and Inuit mothers and their babies than in their white counterparts. Not quite as far north, a survey of 104 pregnant women from three First Nations communities in northern Manitoba found that their serum 25OHD levels ranged from < 15 nmol/L (undetectable) to 63 nmol/L, with mean values of 18, 21, and 24 nmol/L in each of the three communities (Smith, 1999). No information was provided in that report as to whether the women were consuming a traditional or western diet. A chart review was done of all babies born in 1993 and 1994 to determine how many had been diagnosed with rickets, and a high prevalence was found. Despite similar serum 25OHD levels in all three communities, there was a marked difference in the prevalence of rickets: 85/1,000 and 55/1,000 in two communities, but none in the third. No clear explanation for the differing prevalence was obtained by the investigator (Smith, 1999).
Taken as a whole, the limited data surrounding indigenous Canadian populations suggest a basis for concern regarding vitamin D nutriture, most notably in the likelihood that typical diets are changing from traditional foods to more westernized foods. Although the assumption of minimal sun exposure underpinning the DRI values may not entirely align with this group of people who may experience considerable sun exposure in the summer, ensuring that the diet meets the DRI values should provide assurances that risk of vitamin D deficiency has been greatly reduced.
Use of Calcium Supplements
The forms and nature of calcium supplements have been discussed in Chapter 2, and their possible role in kidney stone formation as well as the emerging data regarding possible adverse cardiovascular effects have been outlined in Chapter 6. The mechanisms for differential effects of food sources and supplement forms of calcium on kidney stone formation are complex and may relate to the timing of calcium administration. Approximately 80 percent of kidney stones contain calcium combined with oxalate or, less often, phosphate (Park and Pearle, 2007). Calcium in food or in supplements taken with food is believed to bind to dietary oxalate in the digestive tract, reducing the absorption and subsequent urinary excretion of oxalate and thus risk for kidney stones (urinary oxalate may be more critical than urinary calcium with respect to calcium oxalate crystallization) (Curhan et al., 1997). When calcium supplements are not taken with food, dietary oxalate is absorbed unopposed and thus is more available for stone formation. Although dairy foods, which are the major source of calcium in much of North America, have been suggested to contain an unidentified protective compound not found in supplements (Curhan et al., 1997), this possibility has not been well studied. Obtaining sufficient calcium via dietary sources is the preferred strategy—and it remains uncertain as to whether taking calcium supplements with food may reduce the likelihood of stone formation associated with supplement use. Head-to-head comparisons of different calcium supplement formulations with respect to risk for kidney stone formation are also lacking. In any case, given the desirability of not surpassing the UL for calcium intake and given that even those not meeting their requirement for calcium are nonetheless consuming some calcium from dietary sources that range from breads to dairy products, care must be taken in selecting a calcium supplement that when combined with dietary intake does not result in a total intake above the UL. The UL for a sizable proportion of the population, including groups that commonly consume calcium supplements, is 2,000 mg/day, which is relatively close to the EAR and RDA values. For these more vulnerable groups, supplements containing amounts less than the RDA may be appropriate given that their diet is likely to contain at least some calcium. Further, until better information is available to clarify the possible link between supplement use and kidney stone formation, taking calcium supplements with foods is advisable.
Moreover, in the case of persons prone to developing kidney stones who cannot get adequate calcium from diet (e.g., due to lactose intolerance), there is limited evidence from small, short-term trials suggesting that supplemental calcium in moderate doses may not increase risk for stone recurrence (Levine et al., 1994; Williams et al., 2001; Lewandowski and Rodgers, 2004). Again, taking supplements with food is desirable.
The ULs are defined for the healthy, general population. Nonetheless, gray areas are acknowledged to exist between healthy people and those with medical conditions; for some persons in these gray areas a calcium intake as high as the UL may no longer be considered without any risk. The effect of calcium intake in situations of hypercalciuria is not fully understood, but conditions leading to hypercalciuria (which may be exacerbated by adding extra vitamin D to an already high calcium intake) may warrant a more cautious approach to ULs for calcium in the future. In older adults experiencing illness or decline, hypercalciuria may develop. For pregnant women experiencing absorptive hypercalciuria and therefore at higher risk of renal stone formation, keeping calcium intake below the UL may also be most appropriate. Similarly, as lactation drives bone resorption, urinary calcium excretion decreases, the ionized serum calcium concentration rises slightly, intravascular volume is contracted and occasionally women become hypercalcemic. Under these and similar conditions, ensuring a calcium intake below the UL may be most appropriate. Greater surveillance of urinary calcium excretion in future studies may shed more light on the relationship between higher levels of total calcium intake and risk of hypercalciuria or hypercalcemia under special conditions.
Oral Contraceptive Use
The use of ethinyl estradiol oral contraceptives (OCs) has been hypothesized to reduce bone resorption and preserve bone density in premenopausal and postmenopausal women. This concept was based on clinical and observational evidence that ethinyl estrogen–based hormone replacement therapy reduced risk for osteoporosis in postmenopausal women (Zittermann, 2000). A non-systematic review of clinical trials carried out before 1994 indicated that the evidence at that time largely supported positive effects of OCs on bone density in postmenopausal women, although a number of trials in the review showed no effects (DeCherney, 1996). Among clinical trials and observational evidence examining the effects of OCs on bone density from the past two decades, results have been mixed and, when considered in total, are inconclusive. A systematic review of 75 studies of varied design, including 11 randomized controlled trials, examined outcomes of OC use and bone density in healthy premenopausal, amenorrheic premenopausal, anorexic premenopausal, and perimenopausal women (Liu and Lebrun, 2006). A meta-analysis was not done; however, the review found good evidence for a positive effect of OCs on bone density in perimenopausal women, fair evidence for an effect in amenorrheic premenopausal women, and limited evidence for an effect in anorexic and healthy premenopausal women.
Observational studies published since Liu and Lebrun (2006) also suggest mixed results from studies on OC use and bone density that may be related to the population group studied. A small study on OC use and bone density and bone size in a young white female cohort found that OC use had a significant negative effect on bone density at the spine and heel and resulted in a non-significant decrease in hip bone density (Ruffing et al., 2007). Similarly, Hartard et al. (2007), in a cross–sectional analysis of young white women taking OCs, also suggested a negative effect of OCs on bone density. Women who had ever used OCs had significantly lower bone densities at the tibial shaft and femoral neck compared with those who had never used OCs. In premenopausal and postmenopausal women no significant difference was found between OCs users and never users in another cross–sectional study of the effects of OCs on bone density and bone markers (Allali et al., 2009).
Randomized trials of estrogen treatment with and without vitamin D and calcium supplementation suggest a positive effect on bone density in postmenopausal women. Recker et al. (1999) tested vitamin D and calcium supplementation with and without low-dose hormone replacement therapy for effectiveness in maintaining bone density in postmenopausal women more than 65 years of age. Although this study did not differentiate between hormone replacement therapy alone and therapy combined with vitamin D and calcium supplementation, it did suggest an effect of increasing bone density and bone markers in older women who received the combination therapy compared with those who received vitamin D and calcium supplementation alone. A randomized, double-blind, placebo-controlled trial of OC therapy either alone or combined with calcitriol therapy found a significant increase in bone density and reduction in bone resorption at the hip compared with OC therapy alone in postmenopausal women (ages 65 to 77 years) who had normal bone density for their age (Gallagher et al., 2001). Another prospective randomized trial in postmenopausal women (ages 53 to 79 years) treated with hormone replacement therapy alone or with calcitriol also found a significant increase in bone density, at multiple sites and total body, for the combined therapy compared with hormone replacement alone (Gutteridge et al., 2003).
Given the variability in all the study outcomes reviewed by the committee and the unresolved question of the effect of age and endogenous estrogen status on the ability of OCs to preserve bone density or prevent bone resorption, specific recommendations to address the impact of OCs with or without vitamin D and calcium supplementation for both premenopausal and postmenopausal women cannot be offered at this time.
Premature Infants
Premature infants are a clinical population and thus outside the scope of this committee's task, which is focused on the normal, healthy population. However, because premature infants are a highly vulnerable group and do raise special concerns relative to calcium and vitamin D nutriture, this group is discussed here briefly.
The minerals in human milk, especially calcium and phosphorus, do not fully meet the needs of rapidly growing premature infants who rely primarily on passive intestinal absorption of calcium, therefore “this and other factors place premature infants at high risk for nutritional rickets” (Abrams, 2005). “The recent addition of various forms of mineral salts and/or mineral fortifiers to human milk and the use of specialized preterm infant formulas with high calcium content have been reported to enhance the amount of calcium and other minerals retained from the diet, to increase the bone mineral content of the infants and to decrease the incidence of osteopenia and frank rickets in preterm infants (Schanler et al., 1988; Schanler and Abrams, 1995; Schanler, 1998)... The bioavailability of the calcium in these fortifiers may be a key aspect of their adequacy. Using a commercially available human milk fortifier, Schanler and Abrams (1995) reported that net calcium retention was 104 ± 36 mg/kg body weight per day in premature infants, a value approximating the in utero accretion rate during the third trimester. These retention values are well above those achieved using earlier human milk fortifiers (Schanler et al., 1988)” (Abrams, 2005).
“Of interest is that calcium absorption from both fortified human milk and specialized preterm formula averages 50 to 65 percent in many studies (Abrams et al., 1991; Bronner et al., 1992). This constancy of absorptive fraction in premature infants suggests that much of the calcium absorption by premature infants and newborn full-term infants is not vitamin D dependent…” (Abrams, 2005), which is the conclusion of a review of more than 100 balance studies by Bronner et al. (1992).
How much vitamin D is needed by premature infants is more difficult to determine. Unfortunately, there are no studies using modern isotope techniques of the effects of vitamin D on calcium absorption in premature infants, nor could such studies be possible practically or ethically. One study with oral vitamin D intakes as low as 160 IU/day (Koo et al., 1995) and multiple studies with intakes of 200 to 400 IU/day (Cooke et al., 1990; Pittard et al., 1991; Backstrom et al., 1999a) “demonstrated adequate serum 25OHD concentrations and clinical outcomes with oral vitamin D intakes as low as 160 IU/day (Koo et al., 1995). In addition, studies have generally failed to show any clinical benefit to increasing vitamin D intake above 400 IU/day in preterm infants (Backstrom et al., 1999b)” (Abrams, 2005).
Routine measurement of serum 25OHD levels in premature infants is not supported by currently available clinical research. No studies have related serum 25OHD level in these infants to specific clinical outcomes, and extremely few data suggest a dose–response relationship between serum 25OHD levels and other outcomes. A normal level at different gestational ages or postnatal ages is not available for 25OHD in serum based on end-points such as calcium absorption or bone mineral content. However, in the presence of a likely impairment of 25-hydroxylation, such as might be present in an infant with cholestasis, measurement of serum 25OHD level might be considered, especially to ensure a level at or above 50 nmol/L (20 ng/mL). “The effects of other formula components on mineral absorption have also been considered. A study using a triple lumen perfusion technique demonstrated that calcium absorption was greater using a solution that included a glucose polymer rather than lactose (Stathos et al., 1996). As glucose polymers are widely used in preterm formulas, this effect may be clinically important. Altering the fat blend of infant formula to more closely resemble that of human milk may also enhance mineral absorption in premature infants (Carnielli et al., 1995; Lucas et al., 1997)” (Abrams, 2005).
Interactions Between Vitamin D and Prescription Drugs
Although clinical practice and related guidelines are outside this committee's purview, it is useful to acknowledge that measures of the various forms of vitamin D can be affected by prescription drugs and related medications. A brief listing of key interactions can be found in Table 8-1.
TABLE 8-1
Drugs and Their Effect on Vitamin D Metabolism.
REFERENCES
- Abrams SA, Esteban NV, Vieira NE, Yergey AL. Dual tracer stable isotopic assessment of calcium absorption and endogenous fecal excretion in low birth weight infants. Pediatric Research. 1991;29(6):615β8. [PubMed: 1866219]
- Abrams SA. Chapter 49: Vitamin D deficiency and calcium absorption during infancy and childhood, Vitamin D. 2. Feldman D, Pike JW, Glorieux F, editors. London: Elsevier Academic Press; 2005.
- Adams JS, Wahl TO, Lukert BP. Effects of hydrochlorothiazide and dietary sodium restriction on calcium metabolism in corticosteroid treated patients. Metabolism. 1981;30(3):217β21. [PubMed: 7207196]
- Alemzadeh R, Kichler J, Babar G, Calhoun M. Hypovitaminosis D in obese children and adolescents: relationship with adiposity, insulin sensitivity, ethnicity, and season. Metabolism. 2008;57(2):183β91. [PubMed: 18191047]
- Alfrey AC, Hegg A, Craswell P. Metabolism and toxicity of aluminum in renal failure. American Journal of Clinical Nutrition. 1980;33(7):1509β16. [PubMed: 7395774]
- Allali F, El Mansouri L, Abourazzak F, Ichchou L, Khazzani H, Bennani L, Abouqal R, Hajjaj-Hassouni N. The effect of past use of oral contraceptive on bone mineral density, bone biochemical markers and muscle strength in healthy pre and post menopausal women. BMC Women's Health. 2009;9:31. [PMC free article: PMC2776575] [PubMed: 19887010]
- American Dietetic Association and Dietitians of Canada. Position of the American Dietetic Association and Dietitians of Canada: vegetarian diets. Canadian Journal of Dietetic Practice & Research. 2003;64(2):62β81. [PubMed: 12826028]
- Armas LA, Dowell S, Akhter M, Duthuluru S, Huerter C, Hollis BW, Lund R, Heaney RP. Ultraviolet-B radiation increases serum 25-hydroxyvitamin D levels: the effect of UVB dose and skin color. Journal of the American Academy of Dermatology. 2007;57(4):588β93. [PubMed: 17637484]
- Avioli LV. Heparin-induced osteopenia: an appraisal. Advances in Experimental Medicine and Biology. 1975;52:375β87. [PubMed: 123694]
- Bachrach S, Fisher J, Parks JS. An outbreak of vitamin D deficiency rickets in a susceptible population. Pediatrics. 1979;64(6):871β7. [PubMed: 574626]
- Backstrom MC, Maki R, Kuusela AL, Sievanen H, Koivisto AM, Ikonen RS, Kouri T, Maki M. Randomised controlled trial of vitamin D supplementation on bone density and biochemical indices in preterm infants. Archives of Disease in Childhood. Fetal and Neonatal Edition. 1999;80(3):F161β6. [PMC free article: PMC1720926] [PubMed: 10212074]
- Backstrom MC, Maki R, Kuusela AL, Sievanen H, Koivisto AM, Koskinen M, Ikonen RS, Maki M. The long-term effect of early mineral, vitamin D, and breast milk intake on bone mineral status in 9- to 11-year-old children born prematurely. Journal of Pediatric Gastroenterology and Nutrition. 1999;29(5):575β82. [PubMed: 10554126]
- Basile LA, Taylor SN, Wagner CL, Quinones L, Hollis BW. Neonatal vitamin D status at birth at latitude 32 degrees 72': evidence of deficiency. Journal of Perinatology. 2007;27(9):568β71. [PubMed: 17625571]
- Bell RD, Pak CY, Zerwekh J, Barilla DE, Vasko M. Effect of phenytoin on bone and vitamin D metabolism. Annals of Neurology. 1979;5(4):374β8. [PubMed: 220903]
- Berti PR, Soueida R, Kuhnlein HV. Dietary assessment of Indigenous Canadian Arctic women with a focus on pregnancy and lactation. International Journal of Circumpolar Health. 2008;67(4):349β62. [PubMed: 19024804]
- Binet A, Kooh SW. Persistence of vitamin D-deficiency rickets in Toronto in the 1990s. Canadian Journal of Public Health. [Revue Canadienne de Sante Publique]. 1996;87(4):227β30. [PubMed: 8870299]
- Bodnar LM, Simhan HN, Powers RW, Frank MP, Cooperstein E, Roberts JM. High prevalence of vitamin D insufficiency in black and white pregnant women residing in the northern United States and their neonates. Journal of Nutrition. 2007;137(2):447β52. [PMC free article: PMC4288960] [PubMed: 17237325]
- Bolland MJ, Grey A, Horne AM, Thomas MG. Osteomalacia in an HIV-infected man receiving rifabutin, a cytochrome P450 enzyme inducer: a case report. Annals of Clinical Microbiology and Antimicrobials. 2008:7β3. [PMC free article: PMC2253556] [PubMed: 18226256]
- Brannon PM, Carpenter TO, Fernandez JR, Gilsanz V, Gould JB, Hall KE, Hui SL, Lupton JR, Mennella J, Miller NJ, Osganian SK, Sellmeyer DE, Suchy FJ, Wolf MA. NIH Consensus Development Conference Statement: Lactose Intolerance and Health. NIH Consensus State of the Science Statements. 2010;27(2) [PubMed: 20186234]
- Bronner F, Salle BL, Putet G, Rigo J, Senterre J. Net calcium absorption in premature infants: results of 103 metabolic balance studies. American Journal of Clinical Nutrition. 1992;56(6):1037β44. [PubMed: 1307704]
- Brunborg LA, Julshamn K, Nortvedt R, Frøyland L. Nutritional composition of blubber and meat of hooded seal (Cystophora cristata) and harp seal (Phagophilus groenlandicus) from Greenland. Food Chemistry. 2006;96(4):524β31.
- Byers T. Anticancer vitamins du jour—the ABCED's so far. American Journal of Epidemiology. 2010;172(1):1β3. [PMC free article: PMC2892535] [PubMed: 20562190]
- Carnielli VP, Luijendijk IH, van Goudoever JB, Sulkers EJ, Boerlage AA, Degenhart HJ, Sauer PJ. Feeding premature newborn infants palmitic acid in amounts and stereoisomeric position similar to that of human milk: effects on fat and mineral balance. American Journal of Clinical Nutrition. 1995;61(5):1037β42. [PubMed: 7733025]
- Carvalho NF, Kenney RD, Carrington PH, Hall DE. Severe nutritional deficiencies in toddlers resulting from health food milk alternatives. Pediatrics. 2001;107(4):E46. [PubMed: 11335767]
- Compston JE, Thompson RP. Intestinal absorption of 25-hydroxyvitamin D and osteomalacia in primary biliary cirrhosis. Lancet. 1977;1(8014):721β4. [PubMed: 66519]
- Compston JE, Horton LW. Oral 25-hydroxyvitain D3 in treatment of osteomalacia associated with ileal resection and cholestyramine therapy. Gastroenterology. 1978;74(5 Pt 1):900β2. [PubMed: 640344]
- Cooke R, Hollis B, Conner C, Watson D, Werkman S, Chesney R. Vitamin D and mineral metabolism in the very low birth weight infant receiving 400 IU of vitamin D. Journal of Pediatrics. 1990;116(3):423β8. [PubMed: 2308035]
- Craig WJ. Health effects of vegan diets. American Journal of Clinical Nutrition. 2009;89(5):1627Sβ33S. [PubMed: 19279075]
- Craig WJ, Mangels AR. Position of the American Dietetic Association: vegetarian diets. Journal of the American Dietetic Association. 2009;109(7):1266β82. [PubMed: 19562864]
- Curhan GC, Willett WC, Speizer FE, Speigelman D, Stampfer MJ. Comparison of dietary calcium with supplemental calcium and other nutrients as factors affecting the risk for kidney stones in women. Annals of Internal Medicine. 1997;126(7):497β504. [PubMed: 9092314]
- DeCherney A. Bone-sparing properties of oral contraceptives. American Journal of Obstetrics and Gynecology. 1996;174(1 Pt 1):15β20. [PubMed: 8571999]
- DeLucia MC, Mitnick ME, Carpenter TO. Nutritional rickets with normal circulating 25-hydroxyvitamin D: a call for reexamining the role of dietary calcium intake in North American infants. Journal of Clinical Endocrinology and Metabolism. 2003;88(8):3539β45. [PubMed: 12915633]
- Diffey B. Modelling the seasonal variation of vitamin D due to sun exposure. British Journal of Dermatology. 2010;162:1342β8. [PubMed: 20163416]
- Dobs AS, Levine MA, Margolis S. Effects of pravastatin, a new HMG-CoA reductase inhibitor, on vitamin D synthesis in man. Metabolism. 1991;40(5):524β8. [PubMed: 1902546]
- Favus MJ, Kimberg DV, Millar GN, Gershon E. Effects of cortisone administration on the metabolism and localization of 25-hydroxycholecalciferol in the rat. Journal of Clinical Investigation. 1973;52(6):1328β35. [PMC free article: PMC302395] [PubMed: 4703222]
- Feldman D. Ketoconazole and other imidazole derivatives as inhibitors of steroidogenesis. Endocrine Reviews. 1986;7(4):409β20. [PubMed: 3536461]
- Finkelstein JS, Lee ML, Sowers M, Ettinger B, Neer RM, Kelsey JL, Cauley JA, Huang MH, Greendale GA. Ethnic variation in bone density in premenopausal and early perimenopausal women: effects of anthropometric and lifestyle factors. Journal of Clinical Endocrinology and Metabolism. 2002;87(7):3057β67. [PubMed: 12107201]
- Finkelstein JS, Brockwell SE, Mehta V, Greendale GA, Sowers MR, Ettinger B, Lo JC, Johnston JM, Cauley JA, Danielson ME, Neer RM. Bone mineral density changes during the menopause transition in a multiethnic cohort of women. Journal of Clinical Endocrinology and Metabolism. 2008;93(3):861β8. [PMC free article: PMC2266953] [PubMed: 18160467]
- Ford JA, McIntosh WV, Butterfield R, Preece MA, Pietrek J, Arrowsmith WA, Arthurton MW, Turner W, O'Riordan JL, Dunnigan MG. Clinical and subclinical vitamin D deficiency in Bradford children. Archives of Disease in Childhood. 1976;51(12):939β43. [PMC free article: PMC1546158] [PubMed: 1015846]
- Gallagher JC, Fowler SE, Detter JR, Sherman SS. Combination treatment with estrogen and calcitriol in the prevention of age-related bone loss. Journal of Clinical Endocrinology and Metabolism. 2001;86(8):3618β28. [PubMed: 11502787]
- Goel KM, Sweet EM, Logan RW, Warren JM, Arneil GC, Shanks RA. Florid and subclinical rickets among immigrant children in Glasgow. Lancet. 1976;1(7970):1141β5. [PubMed: 58196]
- Gutteridge DH, Holzherr ML, Retallack RW, Price RI, Will RK, Dhaliwal SS, Faulkner DL, Stewart GO, Stuckey BG, Prince RL, Criddle RA, Drury PJ, Tran L, Bhagat CI, Kent GN, Jamrozik K. A randomized trial comparing hormone replacement therapy (HRT) and HRT plus calcitriol in the treatment of postmenopausal osteoporosis with vertebral fractures: benefit of the combination on total body and hip density. Calcified Tissue International. 2003;73(1):33β43. [PubMed: 14506952]
- Hahn TJ, Birge SJ, Scharp CR, Avioli LV. Phenobarbital-induced alterations in vitamin D metabolism. Journal of Clinical Investigation. 1972;51(4):741β8. [PMC free article: PMC302186] [PubMed: 4335442]
- Harkness L, Cromer B. Low levels of 25-hydroxy vitamin D are associated with elevated parathyroid hormone in healthy adolescent females. Osteoporosis International. 2005;16(1):109β13. [PubMed: 15175848]
- Hartard M, Kleinmond C, Wiseman M, Weissenbacher ER, Felsenberg D, Erben RG. Detrimental effect of oral contraceptives on parameters of bone mass and geometry in a cohort of 248 young women. Bone. 2007;40(2):444β50. [PubMed: 16965947]
- Holick MF. Vitamin D: a millenium perspective. Journal of Cellular Biochemistry. 2003;88(2):296β307. [PubMed: 12520530]
- Hollis BW. Circulating 25-hydroxyvitamin D levels indicative of vitamin D sufficiency: implications for establishing a new effective dietary intake recommendation for vitamin D. Journal of Nutrition. 2005;135(2):317β22. [PubMed: 15671234]
- IOM (Institute of Medicine). Dietary Reference Intakes for Calcium, Phosphorus, Magnesium, Vitamin D, and Fluoride. Washington, DC: National Academy Press; 1997. [PubMed: 23115811]
- IOM. Dietary Reference Intakes: Applications in Dietary Assessment. Washington, DC: National Academy Press; 2000.
- IOM. Evolution of Evidence for Selected Nutrient and Disease Relationships. Washington, DC: National Academy Press; 2002. [PubMed: 25057605]
- IOM. The Development of DRIs 1994-2004: Lessons Learned and New Challenges: Workshop Summary. Washington, DC: The National Academies Press; 2008.
- Jones G. Pharmacokinetics of vitamin D toxicity. American Journal of Clinical Nutrition. 2008;88(2):582Sβ6S. [PubMed: 18689406]
- Katz I, Li M, Joffe I, Stein B, Jacobs T, Liang XG, Ke HZ, Jee W, Epstein S. Influence of age on cyclosporin A-induced alterations in bone mineral metabolism in the rat in vivo. Journal of Bone and Mineral Research. 1994;9(1):59β67. [PubMed: 8154310]
- Kawai M, Rosen CJ. Bone: adiposity and bone accrual-still an established paradigm? National Review of Endocrinology. 2010;6(2):63β4. [PubMed: 20098443]
- Keiver KM, Draper HH, Ronald K. Vitamin D metabolism in the hooded seal (Cystophora cristata). Journal of Nutrition. 1988;118(3):332β41. [PubMed: 2832569]
- Kenny DE, O'Hara TM, Chen TC, Lu Z, Tian X, Holick MF. Vitamin D content in Alaskan Arctic zooplankton, fishes, and marine mammals. Zoo Biology. 2004;23(1):33β43.
- Khosla S, Atkinson EJ, Riggs BL, Melton LJ 3rd. Relationship between body composition and bone mass in women. Journal of Bone and Mineral Research. 1996;11(6):857β63. [PubMed: 8725184]
- Koo WW, Krug-Wispe S, Neylan M, Succop P, Oestreich AE, Tsang RC. Effect of three levels of vitamin D intake in preterm infants receiving high mineral-containing milk. Journal of Pediatric Gastroenterology and Nutrition. 1995;21(2):182β9. [PubMed: 7472905]
- Kuhnlein HV, Barthet V, Farren A, Falahi E, Leggee D, Receveur O, Berti P. Vitamins A, D, and E in Canadian Arctic traditional food and adult diets. Journal of Food Composition and Analysis. 2006;19(6-7):495β506.
- Kuhnlein HV, Receveur O, Soueida R, Berti PR. Unique patterns of dietary adequacy in three cultures of Canadian Arctic indigenous peoples. Public Health Nutrition. 2008;11(4):349β60. [PubMed: 17610753]
- Lalor BC, France MW, Powell D, Adams PH, Counihan TB. Bone and mineral metabolism and chronic alcohol abuse. Quarterly Journal of Medicine. 1986;59(229):497β511. [PubMed: 3763813]
- Lamberg-Allardt C, Kirjarinta M, Dessypris AG. Serum 25-hydroxy-vitamin D, parathyroid hormone and calcium levels in adult inhabitants above the Arctic Circle in northern Finland. Annals of Clinical Research. 1983;15(4):142β5. [PubMed: 6651206]
- Lawson-Matthew PJ, Guilland-Cumming DF, Yates AJ, Russell RG, Kanis JA. Contrasting effects of intravenous and oral etidronate on vitamin D metabolism in man. Clinical Science (London). 1988;74(1):101β6. [PubMed: 3123117]
- Levine BS, Rodman JS, Wienerman S, Bockman RS, Lane JM, Chapman DS. Effect of calcium citrate supplementation on urinary calcium oxalate saturation in female stone formers: implications for prevention of osteoporosis. American Journal of Clinical Nutrition. 1994;60(4):592β6. [PubMed: 8092096]
- Lewandowski S, Rodgers AL. Renal response to lithogenic and anti-lithogenic supplement challenges in a stone-free population group. Journal of Renal Nutrition. 2004;14(3):170β9. [PubMed: 15232796]
- Liu SL, Lebrun CM. Effect of oral contraceptives and hormone replacement therapy on bone mineral density in premenopausal and perimenopausal women: a systematic review. British Journal of Sports Medicine. 2006;40(1):11β24. [PMC free article: PMC2491937] [PubMed: 16371485]
- Looker AC, Pfeiffer CM, Lacher DA, Schleicher RL, Picciano MF, Yetley EA. Serum 25-hydroxyvitamin D status of the US population: 1988-1994 compared with 2000-2004. American Journal of Clinical Nutrition. 2008;88(6):1519β27. [PMC free article: PMC2745830] [PubMed: 19064511]
- Lubin D, Jensen EH, Gies HP. Global surface ultraviolet radiation climatology from TOMS and ERBE data. Journal of Geophysical Research. 1998;103(D20):26061β91.
- Lucas A, Quinlan P, Abrams S, Ryan S, Meah S, Lucas PJ. Randomised controlled trial of a synthetic triglyceride milk formula for preterm infants. Archives of Disease in Childhood. Fetal and Neonatal Edition. 1997;77(3):F178β84. [PMC free article: PMC1720718] [PubMed: 9462186]
- MacLaughlin J, Holick MF. Aging decreases the capacity of human skin to produce vitamin D3. Journal of Clinical Investigation. 1985;76(4):1536β8. [PMC free article: PMC424123] [PubMed: 2997282]
- Matsuoka LY, Ide L, Wortsman J, MacLaughlin JA, Holick MF. Sunscreens suppress cutaneous vitamin D3 synthesis. Journal of Clinical Endocrinology and Metabolism. 1987;64(6):1165β8. [PubMed: 3033008]
- Morin S, Leslie WD. High bone mineral density is associated with high body mass index. Osteoporosis International. 2009;20(7):1267β71. [PubMed: 19034375]
- Palmer FJ, Sawyers TM, Wierzbinski SJ. Cimetidine and hyperparathyroidism. New England Journal of Medicine. 1980;302(12):692. [PubMed: 7354770]
- Park S, Pearle MS. Pathophysiology and management of calcium stones. Urologic Clinics of North America. 2007;34(3):323β34. [PubMed: 17678983]
- Pesonen J, Sirola J, Tuppurainen M, Jurvelin J, Alhava E, Honkanen R, Kroger H. High bone mineral density among perimenopausal women. Osteoporosis International. 2005;16(12):1899β906. [PubMed: 15959613]
- Pittard WB 3rd, Geddes KM, Hulsey TC, Hollis BW. How much vitamin D for neonates? American Journal of Diseases of Children. 1991;145(10):1147β9. [PubMed: 1928007]
- Premaor MO, Pilbrow L, Tonkin C, Parker RA, Compston J. Obesity and fractures in postmenopausal women. Journal of Bone and Mineral Research. 2010;25(2):292β7. [PubMed: 19821769]
- Prentice A, Shaw J, Laskey MA, Cole TJ, Fraser DR. Bone mineral content of British and rural Gambian women aged 18-80+ years. Bone and Mineral. 1991;12(3):201β14. [PubMed: 2021710]
- Recker RR, Davies KM, Dowd RM, Heaney RP. The effect of low-dose continuous estrogen and progesterone therapy with calcium and vitamin D on bone in elderly women. A randomized, controlled trial. Annals of Internal Medicine. 1999;130(11):897β904. [PubMed: 10375338]
- Reid IR. Relationships between fat and bone. Osteoporosis International. 2008;19(5):595β606. [PubMed: 17965817]
- Reinehr T, de Sousa G, Alexy U, Kersting M, Andler W. Vitamin D status and parathyroid hormone in obese children before and after weight loss. European Journal of Endocrinology / European Federation of Endocrine Societies. 2007;157(2):225β32. [PubMed: 17656603]
- Rejnmark L, Jorgensen ME, Pedersen MB, Hansen JC, Heickendorff L, Lauridsen AL, Mulvad G, Siggaard C, Skjoldborg H, Sorensen TB, Pedersen EB, Mosekilde L. Vitamin D insufficiency in Greenlanders on a westernized fare: ethnic differences in calcitropic hormones between Greenlanders and Danes. Calcified Tissue International. 2004;74(3):255β63. [PubMed: 14708040]
- Riedt CS, Cifuentes M, Stahl T, Chowdhury HA, Schlussel Y, Shapses SA. Overweight postmenopausal women lose bone with moderate weight reduction and 1 g/day calcium intake. Journal of Bone and Mineral Research. 2005;20(3):455β63. [PMC free article: PMC4042194] [PubMed: 15746990]
- Rona RJ, Keil T, Summers C, Gislason D, Zuidmeer L, Sodergren E, Sigurdardottir ST, Lindner T, Goldhahn K, Dahlstrom J, McBride D, Madsen C. The prevalence of food allergy: a meta-analysis. Journal of Allergy and Clinical Immunology. 2007;120(3):638β46. [PubMed: 17628647]
- Ruffing JA, Nieves JW, Zion M, Tendy S, Garrett P, Lindsay R, Cosman F. The influence of lifestyle, menstrual function and oral contraceptive use on bone mass and size in female military cadets. Nutrition and Metabolism (London). 2007;4 [PMC free article: PMC1997123] [PubMed: 17683610]
- Schanler RJ, Abrams SA, Garza C. Mineral balance studies in very low birth weight infants fed human milk. Journal of Pediatrics. 1988;113(1 Pt 2):230β8. [PubMed: 3392643]
- Schanler RJ, Abrams SA. Postnatal attainment of intrauterine macromineral accretion rates in low birth weight infants fed fortified human milk. Journal of Pediatrics. 1995;126(3):441β7. [PubMed: 7869208]
- Schanler RJ. The role of human milk fortification for premature infants. Clinics in Perinatology. 1998;25(3):645β57. ix. [PubMed: 9779339]
- Shah M, Salhab N, Patterson D, Seikaly MG. Nutritional rickets still afflict children in north Texas. Texas Medicine. 2000;96(6):64β8. [PubMed: 10876374]
- Shaukat A, Levitt MD, Taylor BC, MacDonald R, Shamliyan TA, Kane RL, Wilt TJ. Systematic review: effective management strategies for lactose intolerance. Annals of Internal Medicine. 2010;152(12):797β803. [PubMed: 20404262]
- Smith PJ. PhD Diss, Smith, P.J. Winnipeg, Canada: U Manitoba; 1999. Vitamin D deficiency in three northern Manitoba communities.
- Specker BL, Valanis B, Hertzberg V, Edwards N, Tsang RC. Sunshine exposure and serum 25-hydroxyvitamin D concentrations in exclusively breast-fed infants. Journal of Pediatrics. 1985;107(3):372β6. [PubMed: 3839846]
- Stathos TH, Shulman RJ, Schanler RJ, Abrams SA. Effect of carbohydrates on calcium absorption in premature infants. Pediatric Research. 1996;39(4 Pt 1):666β70. [PubMed: 8848343]
- Statistics Canada. Canadian Health Measures Survey (CHMS). Ottawa, Ontario: Health Canada; 2010.
- Stein EM, Laing EM, Hall DB, Hausman DB, Kimlin MG, Johnson MA, Modlesky CM, Wilson AR, Lewis RD. Serum 25-hydroxyvitamin D concentrations in girls aged 4-8 y living in the southeastern United States. American Journal of Clinical Nutrition. 2006;83(1):75β81. [PubMed: 16400053]
- Suchy FJ, Brannon PM, Carpenter TO, Fernandez JR, Gilsanz V, Gould JB, Hall K, Hui SL, Lupton J, Mennella J, Miller NJ, Osganian SK, Sellmeyer DE, Wolf MA. National Institutes of Health Consensus Development Conference: lactose intolerance and health. Annals of Internal Medicine. 2010;152(12):792β6. [PubMed: 20404261]
- Sukumar D, Schlussel Y, Riedt CS, Gordon C, Stahl T, Shapses SA. Obesity alters cortical and trabecular bone density and geometry in women. Osteoporosis International. 2011;22(2):635β45. Epub June 9, 2010. [PMC free article: PMC2994953] [PubMed: 20533027]
- Taylor CL. Framework for DRI Development: Components “Known” and Components “To Be Explored.”. Washington, DC: 2008.
- Tzotzas T, Papadopoulou FG, Tziomalos K, Karras S, Gastaris K, Perros P, Krassas GE. Rising serum 25-hydroxy-vitamin D levels after weight loss in obese women correlate with improvement in insulin resistance. Journal of Clinical Endocrinology and Metabolism. 2010;95(9):4251β7. [PubMed: 20534751]
- Ward LM, Gaboury I, Ladhani M, Zlotkin S. Vitamin D-deficiency rickets among children in Canada. Canadian Medical Association Journal. 2007;177(2):161β6. [PMC free article: PMC1913133] [PubMed: 17600035]
- Weaver CM, Proulx WR, Heaney R. Choices for achieving adequate dietary calcium with a vegetarian diet. American Journal of Clinical Nutrition. 1999;70(Suppl 3):543Sβ8S. [PubMed: 10479229]
- Williams SE, Wardman AG, Taylor GA, Peacock M, Cooke NJ. Long term study of the effect of rifampicin and isoniazid on vitamin D metabolism. Tubercle. 1985;66(1):49β54. [PubMed: 3838603]
- Williams CP, Child DF, Hudson PR, Davies GK, Davies MG, John R, Anandaram PS, De Bolla AR. Why oral calcium supplements may reduce renal stone disease: report of a clinical pilot study. Journal of Clinical Pathology. 2001;54(1):54β62. [PMC free article: PMC1731261] [PubMed: 11271790]
- Wilt TJ, Shaukat A, Shamliyan T, Taylor BC, MacDonald R, Tacklind J, Rutks I, Schwarzenberg SJ, Kane RL, Levitt M. Lactose intolerance and health. Evidence Report Technology Assessment (Full Report). 2010;(192):1β410. [PMC free article: PMC4781456] [PubMed: 20629478]
- Wortsman J, Matsuoka LY, Chen TC, Lu Z, Holick MF. Decreased bioavailability of vitamin D in obesity. American Journal of Clinical Nutrition. 2000;72(3):690β3. [PubMed: 10966885]
- Wu H, Gozdzik A, Barta JL, Wagner D, Cole DE, Vieth R, Parra EJ, Whiting SJ. The development and evaluation of a food frequency questionnaire used in assessing vitamin D intake in a sample of healthy young Canadian adults of diverse ancestry. Nutrition Research. 2009;29(4):255β61. [PubMed: 19410977]
- Yesudian PD, Berry JL, Wiles S, Hoyle S, Young DB, Haylett AK, Rhodes LE, Davies P. The effect of ultraviolet B-induced vitamin D levels on host resistance to Mycobacterium tuberculosis: a pilot study in immigrant Asian adults living in the United Kingdom. Photodermatology, Photoimmunology and Photomedicine. 2008;24(2):97β8. [PubMed: 18353091]
- Yetley EA. Assessing the vitamin D status of the US population. American Journal of Clinical Nutrition. 2008;88(2):558Sβ64S. [PubMed: 18689402]
- Yetley EA, Brule D, Cheney MC, Davis CD, Esslinger KA, Fischer PW, Friedl KE, Greene-Finestone LS, Guenther PM, Klurfeld DM, L'Abbe MR, McMurry KY, Starke-Reed PE, Trumbo PR. Dietary reference intakes for vitamin D: justification for a review of the 1997 values. American Journal of Clinical Nutrition. 2009;89(3):719β27. [PMC free article: PMC2667666] [PubMed: 19176741]
- Zhao LJ, Liu YJ, Liu PY, Hamilton J, Recker RR, Deng HW. Relationship of obesity with osteoporosis. Journal of Clinical Endocrinology and Metabolism. 2007;92(5):1640β6. [PMC free article: PMC1868430] [PubMed: 17299077]
- Zhao LJ, Jiang H, Papasian CJ, Maulik D, Drees B, Hamilton J, Deng HW. Correlation of obesity and osteoporosis: effect of fat mass on the determination of osteoporosis. Journal of Bone and Mineral Research. 2008;23(1):17β29. [PMC free article: PMC2663586] [PubMed: 17784844]
- Zittermann A. Decreased urinary calcium loss and lower bone turnover in young oral contraceptive users. Metabolism. 2000;49(8):1078β82. [PubMed: 10954029]
- Zittermann A, Frisch S, Berthold HK, Gotting C, Kuhn J, Kleesiek K, Stehle P, Koertke H, Koerfer R. Vitamin D supplementation enhances the beneficial effects of weight loss on cardiovascular disease risk markers. American Journal of Clinical Nutrition. 2009;89(5):1321β7. [PubMed: 19321573]
Footnotes
- 1
“New methodologies—many from other fields of study—are emerging and can be useful for examining and approximating dose–response relationships when available data are limited. These should be more closely examined and incorporated into the DRI process as appropriate” (Taylor, 2008).
- 2
“There is considerable interest—as well as more than 10 years of experience—surrounding the inclusion of chronic disease indicators within DRI development. A variety of perspectives were put forward. There is a need for focused discussions about how to include chronic disease indicators in the DRI process, including specific approaches for addressing their confounders, identification of appropriate biomarkers, and quantifying their effects” (Taylor, 2008).
- 3
“There is broad interest in addressing the AIs as a component of the DRI values, but no clear path has emerged in terms of clarifying, adapting or eliminating AIs. Nor is there agreement about directions to be taken in the future for AI development” (Taylor, 2008).
- 4
Available online at http://www
β.aad.org/media β/background/news β/Releases/American_Academy β_of_Dermatology β_Issues_Updated_Pos/.(accessed July 28, 2010). - 5
Available online at http://www
β.skincancerprevention.org/ (accessed July 28, 2010). - 6
Available online at http://www
β.vrg.org/journal β/vj2006issue4/vj2006issue4poll.htm (accessed July 28, 2010). - 7
Some algal supplements and mushrooms that have been processed with irradiation contain vitamin D, but not in significant amounts. Available online at http://ods
β.od.nih.gov β/factsheets/vitamind.asp (accessed July 28, 2010). - 8
First Nation: A term that came into common usage in the 1970s to replace the word “Indian.” Among its uses, the term “First Nations peoples” refers to the Indian peoples in Canada, both Status and non-Status. Definitions available online at http://www
β.ainc-inac β.gc.ca/ap/tln-eng.asp (accessed July 28, 2010).
- Implications and Special Concerns - Dietary Reference Intakes for Calcium and Vi...Implications and Special Concerns - Dietary Reference Intakes for Calcium and Vitamin D
- Taxonomy Links for Nucleotide (Select 1674988518) (1)Taxonomy
Your browsing activity is empty.
Activity recording is turned off.
See more...