This book is distributed under the terms of the Creative Commons Attribution-NonCommercial-NoDerivatives 4.0 International (CC BY-NC-ND 4.0) ( http://creativecommons.org/licenses/by-nc-nd/4.0/ ), which permits others to distribute the work, provided that the article is not altered or used commercially. You are not required to obtain permission to distribute this article, provided that you credit the author and journal.
NCBI Bookshelf. A service of the National Library of Medicine, National Institutes of Health.
StatPearls [Internet]. Treasure Island (FL): StatPearls Publishing; 2024 Jan-.
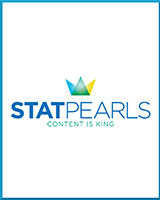
StatPearls [Internet].
Show detailsContinuing Education Activity
Hemolytic disease of the fetus and newborn is characterized by the destruction of fetal red blood cells, primarily due to Rh or ABO incompatibility between the mother and fetus, although more rare blood incompatibilities may also cause this condition. While Rh incompatibility typically manifests in subsequent pregnancies, ABO incompatibility may affect the first pregnancy due to preexisting maternal antibodies. The course of the disease varies, with Rh incompatibility posing a greater risk of severe complications such as intrauterine hydrops fetalis.
Clinical features of affected newborns include lethargy, jaundice, hepatosplenomegaly, and, in severe cases, hydrops fetalis signs. Hemolytic disease of the fetus and newborn can be diagnosed antenatally or postnatally through various laboratory and imaging studies, depending on when the evaluation is conducted. Treatment approaches include supplementation, phototherapy, and, in rare cases, transfusion. However, the most critical intervention is alloimmunization prevention in Rh-negative pregnant women. Conversely, ABO incompatibility typically has a milder course and rarely requires immunoglobulin prophylaxis. This activity for healthcare professionals aims to enhance learners' competence in selecting appropriate diagnostic tests, managing and preventing hemolytic disease of the fetus and newborn, and fostering effective interprofessional teamwork to improve the care provided to mothers and newborns affected by this condition.
Objectives:
- Identify the clinical features of hemolytic disease of the fetus and newborn.
- Screen patients for hemolytic disease of the fetus and newborn.
- Select the appropriate management for hemolytic disease of the fetus and newborn.
- Apply interprofessional team strategies to improve care coordination and outcomes of patients with hemolytic disease of the fetus and newborn.
Introduction
Hemolytic disease of the fetus and newborn (HDFN), also known as erythroblastosis fetalis, is a complex and potentially life-threatening condition arising from maternal-fetal blood group incompatibility. When a fetus inherits paternal blood group factors that are absent in the mother, antepartum or intrapartum fetal-maternal bleeding can provoke a maternal immune response.[1] This immune reaction produces maternal antibodies, a process known as alloimmunization, which can result in varying degrees of transplacental passage of these antibodies into the fetal circulation. Depending on the antigenicity and amount of antibodies involved, this transplacental transfer can lead to hemolytic disease in the fetus and neonate, presenting with complications including anemia, jaundice, and, in severe cases, hydrops fetalis or hyperbilirubinemia and kernicterus in the newborn.[2][3]
Despite significant advancements in the understanding and management of HDFN, the condition remains a significant cause of perinatal morbidity and mortality if left undiagnosed and untreated. However, with the advent of Doppler ultrasonography, noninvasive methods for managing alloimmunization in pregnant women have emerged, supplementing established protocols for HDFN management. These advancements offer a more thorough and less invasive approach to assessing fetal well-being, reducing risks to both the mother and fetus. Additionally, the intricate nomenclature of the Rh blood group system adds complexity to the management of Rh alloimmunization, necessitating a clear understanding of antigenic variants and their implications for clinical practice.[4][5] Therefore, enhanced knowledge of the pathogenesis, prevalence, prevention, and management strategies of HDFN, as well as the importance of timely intervention and the role of evolving diagnostic techniques in improving outcomes for affected pregnancies.
Etiology
HDFN is caused by the destruction of the fetus or neonate's red blood cells (RBCs) by maternal immunoglobulin G (IgG) antibodies due to maternal-fetal blood group incompatibility. These maternal antibodies are produced, termed alloimmunization, when a foreign RBC antigen from an exogenous source (eg, growing fetus or transfusion) enters the maternal circulation and becomes exposed to her immune system, resulting in sensitization. As little as 0.1 mL of fetal blood entering maternal circulation is sufficient to cause alloimmunization.[2] In fact, 15% to 50% of gestations have sufficient fetomaternal hemorrhage to cause alloimmunization, and only 1% to 2% of all Rh alloimmunization is caused by antepartum hemorrhage.[2] The primary mechanisms by which sensitization occurs involve the Rh and ABO systems.[1][2][3]
In Rh incompatibility, fetal blood carries paternal Rh-positive antigens and is perceived by the maternal immune system of an Rh-negative mother as foreign. Maternal antibodies are produced, particularly anti-D antibodies. Subsequent pregnancies with Rh-positive fetuses can lead to increased antibody production, as the mother's immune system retains memory of the antigen. A similar mechanism operates in ABO incompatibility, where maternal antibodies target fetal RBCs carrying A or B antigens not present in maternal blood. This immune response can result in severe complications for the fetus or neonate, including anemia, jaundice, hydrops fetalis, or kernicterus.[4][5]
Despite advancements in Rh immunoprophylaxis, HDFN remains a concern globally, albeit with decreased incidence following the introduction of prophylactic measures. Ethnic disparities exist in Rh-negative prevalence, with higher rates among certain populations. ABO incompatibility, though prevalent, results in a relatively lower proportion of HDFN cases, possibly due to the modest immune response elicited by ABO antigens. Fetomaternal hemorrhage, occurring during pregnancy, delivery, or obstetrical emergencies (eg, ectopic pregnancy or miscarriage), plays a crucial role in HDFN pathogenesis, facilitating the entry of fetal blood into the maternal circulation and subsequent alloimmunization. Obstetric procedures (eg, chorionic villus sampling and amniocentesis) also pose risks of fetomaternal hemorrhage, underscoring the importance of vigilance in managing maternal sensitization and preventing HDFN.[4][5]
Epidemiology
HDFN was first described by Dr. Louis K. Diamond in 1932 when he wrote about erythroblastosis fetalis in the newborn based on peripheral smears.[6] The reported prevalence of HDFN due to any cause is 1695 per 100,000 live births. ABO incompatibility occurs in approximately 20% of births, though only 1% of these newborns develop HDFN.[7] However, the prevalence of Rh-induced HDFN has declined due to RhD-negative immunoprophylaxis (ie, RhoGAM), which was first introduced in 1968. Since then, the prevalence of Rh-induced HDFN has decreased from 99 per 100,000 live births to 44 per 100,000 live births. Though RhD immune globulin has become more standardized, approximately 0.1% to 0.4% of at-risk women still become sensitized during pregnancy, most likely due to antigens other than RhD.[8] According to studies, the highest prevalence of HDFN in the United States is in Black female neonates from southern states and lowest in White male infants from the West or Midwest regions.[8] Furthermore, HDFN varies by ethnicity, estimated as 15% to 16% in White women, 8% in African-American women, 4% in African women, <1% in Asian women, and 30% to 35% in Spanish and French women.[1]
Pathophysiology
HDFN is precipitated by sensitization of the maternal immune system due to exposure to foreign RBC antigens, which produce maternal antibodies. RBC antigens vary in composition (ie, protein or carbohydrate) and function. Immunoglobulin G (IgG) arises from polypeptide antigens and is less reactive than IgM antibodies produced by carbohydrate antigens. Maternal and fetal blood incompatibility is primarily due to Rh and ABO antigen systems.[1] The severity of HDFN depends on the degree of anemia caused by the underlying incompatibility. Generally, Rh incompatibility results in HDFN that is more severe than ABO-induced HDFN.[1]
Hydrops fetalis, which can be a severe complication of HDFN, results when an underlying etiology increases the fluid transudation rate from the vascular compartment or delays lymphatic return to circulation. Hypoxia may occur secondary to immune hydrops fetalis, increasing catecholamines and decreasing hepatic and renal blood flow because of the redistribution of blood to the brain, heart, adrenals, and ductus venosus. Subsequently, the renin-angiotensin system is activated, leading to an increase in antidiuretic hormone and a decrease in albumin. As a result, central venous pressure is elevated, and lymphatic flow into the systemic circulation is decreased, in turn causing the loss of intravascular volume and severe and progressive fetal edema.[9]
Rh-Induced Hemolytic Disease
According to the Fisher-Race nomenclature system, the primary RBC antigens involved in blood typing are C, c, D, E, and e, and their antibodies are anti-C, anti-c, anti-D, anti-E, and anti-e. Furthermore, many antigen variants have been identified, including Cw and Du (ie, weak D) antigens, though less frequently encountered. Rh alloimmunization due to the D antigen is the most common cause of HDFN. Therefore, the Rh-positive classification refers to the presence of the D antigen, and the Rh-negative classification indicates an absent D antigen. Zygosity, or similarity of alleles, is also significant in antigen expression. Approximately 40% of Rh-positive individuals are homozygous for the D antigen (DD), and the remaining 60% are heterozygous (Dd).[10] Several other non-Rh antigen variants, including Lewis, I, Kell, Duffy, and Kidd, can also be associated with HDFN, though they differ in severity. Lewis and I are IgM antibodies and do not cross the placental barrier; therefore, HDFN does not result from these antigens. However, the Kell antibody (anti-K), typically produced following a transfusion, can result in severe HDFN with severe anemia, hydrops fetalis, and fetal death. Management of HDFN secondary to other antigenic derivations is the same as those cases caused by D alloimmunization.[2]
The physiologic mechanism underlying Rh alloimmunization involves fetal blood carrying paternal Rh-positive antigens, which the immune system of an Rh-negative mother perceives as foreign. Antibodies that develop are IgM type, which cannot cross the placental barrier. However, subsequent pregnancies are at risk for HDFN because maternal antibodies rapidly develop IgG antibodies after another encounter with the Rh-D antigen, which will cross the placenta and destroy fetal erythrocytes.[11][2] In utero, severe fetal anemia resulting from this immune response can cause hydrops fetalis when the fetal hemoglobin decreases to approximately <7 g/dL below the gestational age average, which is manifested by diffuse edema, pleural and pericardial effusions, and ascites.[12]
Additionally, as fetal RBCs are broken down, excess bilirubin enters fetal circulation, which the placenta in utero removes. However, at birth, the fetal liver must begin processing the bilirubin. Indirect bilirubin, or unconjugated bilirubin, is conjugated to direct bilirubin using the enzyme uridine diphosphate-glucuronosyltransferase. This conjugated bilirubin is excreted in bile and ultimately expelled through feces and urine. In infants, especially preterm infants, liver processing is less efficient, which often leads to natural physiologic jaundice.[13] With excess breakdown products from HDFN, these immature processing mechanisms are overwhelmed, and the resulting hyperbilirubinemia can be prominent. The accumulation of unconjugated bilirubin can lead to kernicterus, a neurologic condition that develops as the unbound bilirubin crosses the blood-brain barrier and deposits in the brain and central nervous system of the developing newborn.[14] Prompt recognition and treatment of hyperbilirubinemia and HDFN are paramount to avoiding long-term neurologic dysfunction in these infants.
ABO-Induced Hemolytic Disease
Inherent ABO incompatibility, which occurs in 15% to 25% of pregnancies, is another pathophysiologic mechanism that can cause HDFN.[15] Only about 1% of those pairs, those with high IgG titers, will develop HDFN due to ABO incompatibility.[15] The ABO system comprises the blood types A, B, AB, and O. Individuals produce anti-A or anti-B antibodies depending on the antigens not present in their RBCs at ages as young as 3 months, through exposure to these foreign antigens in various foods and bacteria. Consequently, ABO-induced HDFN can occur even during the first pregnancy, mainly in women with an O blood type and a fetus with an A, B, or AB blood type.[1] Compared with Rh-induced HDFN, ABO incompatibility generally causes a less severe HDFN. Studies have posited that this may be due to fetal RBCs expressing fewer ABO blood group antigens than adults or that ABO blood group antigens are expressed by many tissues, reducing the chance that antibodies specifically target the antigens on fetal RBCs.[16]
History and Physical
Hemolytic Disease of the Fetus and Newborn Clinical Features
The clinical features of HDFN vary based on several factors, including whether presentation occurs antenatally or postnatally. Antenatally, Rh incompatibility is typically identified incidentally during routine prenatal anti-D antibody screening, indicating a risk for HDFN; therefore, further evaluation through imaging studies is performed. Postnatally, characteristic findings of HDFN can include early onset jaundice and unconjugated hyperbilirubinemia within 24 hours after delivery, moderate to severe anemia, and hydrops fetalis.[1]
In patients with a risk for HDFN, a detailed history regarding maternal and paternal Rh blood grouping, blood transfusions, previous pregnancies, especially with prior Rh-induced hemolytic disease, trauma or invasive obstetric procedures, spontaneous or induced abortions, and administration of Rh IgG should be obtained. Emphasis should be placed on identifying any events that may have led to fetal-maternal hemorrhage, including trauma, miscarriages, ectopic pregnancies, early pregnancy terminations, placental abruption, molar pregnancies, chorionic villous sampling, amniocentesis, and routine delivery procedures (ie, vaginal or cesarean section).[2][1][2]
Blood typing is a component of standardized prenatal testing for all pregnant women in the first trimester. Women with blood type O naturally express antibodies to A and B blood types; thus, their infants should be monitored for the development of HDFN, especially at delivery and directly postpartum. The antibodies expressed in mothers with O blood type are typically IgG and can cross the placenta. Conversely, mothers with blood type A have antibodies against blood type B, which are predominantly IgM and do not cross the placenta.[15] Newborn blood types with maternal O blood types are routinely evaluated for HDFN signs, whereas infants whose mothers have Rh-positive A, B, or AB blood types are not at risk for this condition. Clinical features of HDFN include lethargy, jaundice, hepatosplenomegaly, scleral icterus, tachycardia, tachypnea, and hypotension. In severe cases, hydrops fetalis may arise, a severe HDFN manifestation associated with a significant mortality rate estimated to be >50%.[17][18][19][14][1]
Severe Disease Clinical Features
In patients with severe hemolytic anemia secondary to Rh or ABO incompatibility, hydrops fetalis may develop, which can be recognized due to clinical findings, eg, skin edema, pericardial effusions, pleural effusions, and ascites. Hydrops fetalis is commonly identified antenatally on ultrasound imaging with an abnormal fluid collection in 2 or more cavities or compartments. The number of fluid collection sites is directly correlated to the neonatal prognosis. Ascites can be the initial manifestation of hydrops fetalis as early as 20 weeks of gestation. Skin edema is a subcutaneous tissue thickness on the scalp >5 mm.[20] Other antenatal features include anemia, placentomegaly, polyhydramnios, and hepatosplenomegaly. Pleural effusion can be unilateral or bilateral.
The diagnosis is typically based on prenatal ultrasound; however, hydrops may present with cardiovascular shock due to severe anemia during neonatal evaluation. Mild effusions can cause respiratory distress, and severe effusions result in lung hypoplasia and respiratory or circulatory diseases associated with poor prognosis after birth.[21] Neonates can also present with pneumothorax or chylothorax, which are rare and serious clinical conditions with high mortality.[22][23] Additionally, untreated hemolytic anemia in a neonate will progress from hyperbilirubinemia with jaundice to kernicterus due to unconjugated bilirubin accumulating in the basal ganglia and causing neuronal death. Subsequently, kernicterus can result in neurodevelopmental impairment, sensorineural deafness, spastic cerebral palsy, and death.[24]
Evaluation
Prenatal Diagnostic Evaluation
Although the assessment approach differs, HDFN evaluation can be conducted antenatally or postpartum, depending on when the condition is suspected. Due to current prenatal care guidelines and diagnostic imaging advances, Rh incompatibility or clinical signs of HDFN on imaging are frequently identified incidentally. All pregnant women undergo an ABO blood group and Rh-D type and antibody screening assessment at the first prenatal visit with each pregnancy. HDFN evaluation begins by first determining risk by identifying the presence of maternal RBC antibodies in an indirect antibody test (ie, Coombs test) or a positive direct antibody test (DAT) of the infant's serum.[16] Detection of maternal anti-D antibodies is diagnostic of D alloimmunization and indicates fetal risk for HDFN. However, alloimmunization is diagnostic of HDFN.
If a woman is Rh-positive, no further testing is necessary. Clinicians should repeat an antibody screen in Rh-negative women before anti-D immune globulin administration. Maternal serum antibody identification and serial titers to determine antibody concentrations should be performed in Rh-negative pregnant women with alloimmunization of a clinically significant antibody. Some antibodies are not clinically significant (eg, anti-N, anti-Lea, and anti-Lu), and further evaluation is unnecessary, while women with antibodies (eg, anti-K and anti-c) associated with fetal complications with any titer require referral to a maternal medicine specialist. In women with other antibodies (eg, anti-D and anti-C), management by a specialist is only needed for elevated titers.[24]
Additionally, antibody titers should be interpreted based on clinical history and the antibody identified. In women without a history of Rh-induced hemolytic disease, titers reflect the immune response in the current pregnancy, and levels that are above the critical threshold help clinicians determine when further testing is needed due to the risk of HDFN; however, in those with a previous history of HDFN, elevated titer levels do not consistently correlate with an increased HDFN risk. For anti-D titers, a threshold between 1:8 and 1:32 is commonly utilized as an indication for fetal anemia evaluation due to a high risk for severe HDFN and hydrops fetalis. Further assessment is not indicated in patients with an antibody D titer of ≤1:8; expectant management with repeated titers every 4 weeks may be performed.[2] However, anti-K titers typically use a lower critical threshold, such as ≥1:4.[25]
In pregnant women with clinically significant titers, paternal RBC antigens should also be assessed to determine the risk of HDFN in cases where paternity is assured. For instance, heterozygosity for the D antigen indicates a 50% chance that the fetus is Rh-negative and not at risk for HDFN. A positive paternal evaluation for the antigen being tested indicates that fetal antigen testing should be conducted, preferably with cell-free DNA, though this is not currently widely available in the United States.[26][2] In a fetus with an antibody titer above the critical threshold and paternal zygosity is heterozygous or unknown paternity, clinicians should determine fetal blood type through polymerase chain reaction (PCR) of an amniotic fluid specimen obtained by amniocentesis if cell-free DNA is unavailable or was inconclusive.[2]. Furthermore, amniotic fluid PCR can be used if amniocentesis is performed for another reason (eg, aneuploidy diagnostic testing). Amniocentesis can be performed as early as 16 to 20 weeks to collect fetal cells for antigen analysis. If negative, the pregnancy can be followed expectantly; however, if the fetus is Rh-positive, fetal anemia is noninvasively assessed using fetal middle cerebral artery (MCA) Doppler measurements.[27]
Middle cerebral artery systolic velocity assessment
Historically, prior to the MCA Doppler, the evaluation of an Rh-positive fetus in an Rh-negative woman with positive titers ≥1:16 was conducted with serial amniocenteses to assess amniotic fluid bilirubin levels by a spectrophotometer.[2] However, MCA Doppler assessment of peak systolic velocity has become the standard evaluation for fetal anemia since studies demonstrated conclusive evidence of greater blood flow in fetal anemic brains. Typically, serial MCA Doppler assessment is performed every 1 to 2 weeks from 24 weeks gestation.[1] A peak systolic velocity measurement of >1.5 MoM is consistent with fetal anemia and merits more invasive testing and potential treatment.[28] However, the false-positive rate can increase after 34 to 35 weeks gestation. Clinical and MCA Doppler findings guide further antenatal and delivery management.[2]
Additional imaging evaluation
Hydrops fetalis may be an incidental finding during a routine prenatal anatomy ultrasound between 18 and 22 weeks gestation. The underlying cause directly influences the development of symptoms and their prognosis. Therefore, clinicians should conduct a detailed evaluation of differential diagnoses. The most common findings detected during early pregnancy are ascites and skin edema (>5 mm thickness) in the fetal head, back of the neck, thorax, and abdomen. Polyhydramnios and placental edema are most commonly seen before 20 weeks, whereas pleural effusion and pericardial effusion in the fetus are rarely seen before 15 weeks of gestation.[29] Furthermore, fetal ultrasounds are usually performed with MCA Doppler assessment to monitor for developing signs of fetal anemia and hydrops fetalis, including ascites, soft-tissue edema, scalp edema, pleural effusion, cardiomegaly, placental enlargement, hepatomegaly with portal hypertension associated with hydrops fetalis.[1][30]
Postnatal Diagnostic Evaluation
A fetus delivered due to known hemolytic disease requires surveillance for anemia and hyperbilirubinemia that can result secondary to hemolysis. Additionally, newborns with risk factors for hemolytic disease (eg, a positive or unknown maternal antibody screen) or with clinical signs of hemolytic disease, including jaundice within 24 hours after birth and symptomatic anemia, should be closely monitored. Typically, serial bilirubin levels are monitored through transcutaneous bilirubin (TcB) or total serum bilirubin (TSB) testing. Hemolysis from any cause will demonstrate characteristic findings of a rapid increase in the TSB or TcB of >0.3 mg/dL per hour within the 24 hours following delivery or >0.2 mg/dL per hour following the first 24 hours.[24][4] Infant blood type is confirmed through a DAT using cord blood collected immediately after delivery or obtained from the newborn. Furthermore, a complete blood count and reticulocyte count to evaluate anemia is common. A cross-match may also be performed when a transfusion is being considered.[4][2] In neonates with a maternal blood incompatibility (eg, Rh, non-Rh, or ABO) and laboratory findings of hemolysis, a positive DAT is diagnostic of HDFN. If the DAT is negative, an indirect antiglobulin test is recommended for diagnostic support since some incompatibilities (eg, ABO) are not as reliably excluded by DAT.[1]
Treatment / Management
Prenatal Management
A maternal-fetal medicine specialist should be consulted when HDFN is identified or suspected in utero for management, including intrauterine transfusions and delivery.[2] A peak systolic velocity measurement of >1.5 MoM is typically an indication for cordocentesis (ie, fetal blood sampling) performed by a maternal-fetal medicine specialist to determine the fetal hemoglobin level every 2 weeks. An intrauterine transfusion is considered when the fetal hemoglobin is <2 standard deviations below the mean for gestational age or the hematocrit is <30%.[10]
The optimal timing for delivery in patients with HDFN is debated. The most common management goal is to delay delivery until a gestational age of fetal survivability is attained. Continuing the pregnancy until 37 to 38 weeks gestation in patients with evaluation findings consistent with mild HDFN may be considered. However, in patients with severe HDFN, the benefit-risk ratio of infant prematurity versus multiple invasive procedures, including cordocentesis and transfusions. In these patients, many experts have recommended performing delivery at 32 weeks gestation, when most institutions have a high rate of neonatal survival, following maternal steroid administration and with the last intrauterine transfusion at 30 to 32 weeks gestation. However, others have suggested intrauterine transfusion be performed until 36 weeks gestation, with delivery between 37 and 38 weeks of gestation.[2]
Newborn Management
Following delivery, HDFN is managed by treating hyperbilirubinemia and anemia. Hydrops fetalis requiring acute treatment at delivery may require emergent transfusion, pleural effusion and ascites drainage, and stabilization of respiratory distress.[31] Blood transfusions should use ABO-matched packed RBCs. If immediate transfusion is needed, O-type, Rh-negative blood that has been leukodepleted and irradiated should be available at delivery.[32] HDFN not associated with hydrops fetalis can typically be treated with phototherapy and, if needed, exchange transfusions. Routine universal screening with TcB often occurs at 24 hours of life, but screening should be conducted as soon as hyperbilirubinemia is suspected. An elevated TcB should always be verified with a serum TSB. Nomograms, interpreted using the hour-specific postnatal age, the bilirubin level, and hyperbilirubinemia neurotoxicity risk factors, guide the need for monitoring and provide thresholds for starting phototherapy and exchange transfusions.[14][4] Risk factors for kernicterus include:
- Gestational age <38 weeks with increasing risk as gestational age decreases
- Albumin <3.0 g/dL
- Isoimmune hemolytic disease (ie, positive direct antiglobulin test), G6PD deficiency, or other hemolytic conditions
- Sepsis
- Significant clinical instability for the previous 24 hours [4]
Phototherapy was introduced in the 1970s and has become the preferred treatment for hyperbilirubinemia in newborns and is recommended by the American Academy of Pediatrics (AAP). Photo isomerization causes the transformation of bilirubin into a water-soluble isomer that the kidneys and stool can then excrete without the need for processing in the liver. The primary purpose of phototherapy is to maintain the serum bilirubin concentration below the level where invasive therapies, including exchange transfusion, are necessary. Phototherapy efficacy is determined by light wavelength, intensity, total dose (ie, time exposed and surface area exposed), and the threshold at which phototherapy is initiated. The optimal light for phototherapy has a wavelength of 460 to 490 nm at a close distance, approximately 20 cm above the infant. Moreover, double phototherapy has proven to be more efficacious than single. Evidence is limited regarding the efficacy of continuous versus intermittent phototherapy for infants >2000 g.[32][1] During phototherapy, mothers should be encouraged to breastfeed their infants at timely intervals despite needing to remove them from phototherapy to do so.[1]
An exchange transfusion may be needed for severely anemic newborns, which involves replacing infant RBCs with antigen-negative RBCs, thereby preventing further hemolysis. 5mL/kg aliquots are removed and replaced over several minutes for a total of 25 to 50 mL/kg exchange of RBCs. Before an exchange, transfusion escalation of care should be initiated, including intensive phototherapy, intravenous hydration, and serum laboratory studies, including complete blood count, comprehensive metabolic panel, and type and crossmatch. Additionally, a TSB level should be obtained every 2 hours.[4] The AAP states that escalation of care is indicated when an infant’s TSB reaches 2 mg/dL below the exchange transfusion threshold. Additionally, the AAP recommends exchange transfusions if total bilirubin levels remain above the exchange transfusion threshold despite intensive phototherapy or if signs of bilirubin encephalopathy are present. Similar to phototherapy, the exchange transfusion threshold is guided by a nomogram (see Image. Nomogram for Exchange Transfusions in Newborns).[4] An albumin level should be measured if an exchange transfusion is being considered. An albumin of ≤3.0 g/dL is considered an independent risk factor for hyperbilirubinemia and lowers the phototherapy threshold. Without sufficient albumin to bind bilirubin, the amount of free, unconjugated bilirubin increases, thereby increasing the risk for kernicterus. Adverse effects associated with exchange transfusion are severe cardiorespiratory conditions, thrombosis, catheter complications, metabolic abnormalities, necrotizing enterocolitis, hypothermia, and intestinal perforation.[32][1]
Other treatment modalities have been considered but are still controversial. Intravenous immunoglobulin (IVIG) in the infant may block Fc receptors on macrophages, thereby decreasing the breakdown of antibody-coated RBCs. The AAP recommends IVIG if total serum bilirubin continues to rise despite intensive phototherapy or is within 2 to 3 mg/dL of the exchange transfusion level. Administration of IVIG to mothers before delivery has not been shown to be efficacious and is not currently recommended. Other agents such as albumin, phenobarbital, metalloporphyrins, zinc, clofibrate, and prebiotics have been studied as possible treatment options for hyperbilirubinemia, but none are currently recommended.[33] In a recent randomized control trial of 70 infants with Rh-alloimmunization, delayed cord improved anemia without increasing the incidence of adverse events. Delayed cord clamping had no significant impact, however, on the need for exchange transfusion or duration of phototherapy.[34]
Differential Diagnosis
HDFN should be included in the differential diagnosis of infants with early, severe, or prolonged jaundice and anemia. Other etiologies of jaundice and hyperbilirubinemia in the newborn include physiologic jaundice, prematurity, breast milk and breastfeeding jaundice, G6PD deficiency, thalassemia, sepsis, birth trauma, Gilbert syndrome, and hypothyroidism.[35] Moreover, several conditions may have similar symptoms to HDFN and should be considered during evaluation. Polyhydramnios is the vertically measured amniotic fluid volume in the deepest pocket of more than 8 centimeters or an amniotic fluid index of more than 24 centimeters.[36] The most common causes include impaired fetal swallowing, impaired renal function, and intestinal obstruction.[22] Placentomegaly is an abnormally enlarged placenta that occurs due to disruption in the oncotic gradient. High cardiac output diseases like anemia and sacrococcygeal teratoma are conditions other than HDFN that may cause placentomegaly and polyhydramnios.[37] Hepatosplenomegaly is usually associated with myeloproliferative disease in trisomy 21.[38]
Hydrops fetalis can also develop due to nonimmune conditions, termed nonimmune hydrops fetalis, and accounts for approximately 80% to 90% of all cases. Nonimmune hydrops occurs when an underlying disease, genetic disorder, or birth defect interferes with the fetus's ability to manage fluid. These etiologies are broad, including cardiac (eg, paroxysmal supraventricular tachycardia, hypoplastic left heart), chromosomal (eg, Turner and Edward syndromes), lymphatic, and infectious (eg, Parvovirus B19 and cytomegalovirus) conditions.[39] Neonates with cyanosis and no response to oxygen supplements should raise suspicion of the presence of underlying cardiac diseases.[40] Neonates with hypotonia suggest the presence of congenital myopathy and congenital hypothyroidism.[41][42] In addition to hypotonia, the presence of hepatomegaly, cardiomyopathy, and facial dysmorphism suggest metabolic storage diseases.[43] Dermatitis, along with or without hepatosplenomegaly, suggests TORCH (toxoplasmosis, rubella, cytomegalovirus, and herpes simplex) infections.[44] Other differential diagnoses that should be considered include:
- Parvovirus-B19
- Bart hemoglobin
- Alpha-globin chain mutation
- Twin-twin transfusion
- Spherocytosis
- Microangiopathic hemolytic anemia
- Hereditary enzyme deficiencies
- Chronic fetomaternal hemorrhage
- Alpha thalassemia [45]
Prognosis
The overall prognosis of HDFN is good if identified and treated promptly. While permanent neurologic dysfunction may result from delays in care, this is now a rare occurrence with the advancements in monitoring and prophylaxis against HDFN.[46] In subsequent pregnancies of women with a previous alloimmunized pregnancy, the prognosis depends on the success of interventions to mitigate fetal hydrops development.[10]
Complications
Immune hydrops fetalis and kernicterus are complications that can result due to severe HDFN.[39] Kernicterus secondary to buildup of bilirubin in an infant's brain may manifest as hypotonia or poor suck reflex, which then progresses to irritability and hypertonia with retrocollis and opisthotonos. Long-term consequences of kernicterus can include cerebral palsy, auditory dysfunction, paralysis of upward gaze, and permanent intellectual dysfunction.[14] Thus, early recognition and treatment are imperative to prevent the detrimental progression of HDFN. Additional complications associated with HDFN include:
- Bradycardia
- Limb cyanosis
- Metabolic abnormalities
- Thrombocytopenia
- Anemia [1]
Consultations
A maternal-fetal medicine specialist should be consulted when HDFN is identified or suspected in utero for management, including intrauterine transfusions and delivery.[2] Additionally, radiologists, neonatologists, and pediatric gastroenterologists may be indicated in patients with severe HDFN.[4]
Deterrence and Patient Education
Anti-D immune globulin prevents sensitization of women with a Rh-negative blood type. Since its introduction, a significant decline of 80% to 90% in the incidence of Rh-hemolytic disease has been observed, along with a two-thirds decline in the mortality rate in nationwide surveillance. Administration of anti-D immune globulin to Rh-negative mothers at the time of antigen exposure, eg, the birth of an Rh-positive child, blocks the primary immune response to the foreign cells. Therefore, maternal antibodies to Rh-positive cells are not produced in subsequent pregnancies, and hemolytic disease of the neonate is averted.[47] Rh alloimmunization continues to be a significant issue in areas with limited anti-D immune globulin access or availability.[48]
A standard prophylactic dose of 300 mcg of anti-D immune globulin prevents Rh D alloimmunization following interaction with 15 mL of fetal RBCs. Consequently, a regimen of anti-D immune globulin 300 mcg should be administered once at 28 weeks, which is effective until 40 weeks gestation due to a half-life of approximately 12 weeks. A second dose should be given after birth if Rh D typing identifies a newborn as Rh-positive. However, if a woman has exposure to >15 mL of Rh D-positive fetal RBCs, alloimmunization may occur. Therefore, following delivery, an erythrocyte rosette screen is performed to identify if >2 mL of fetal whole blood is in the maternal circulation.
A positive rosette screen indicates a quantitative test, the Kleihauer-Betke acid-elution test, should be performed to calculate the percentage of fetal RBCs in maternal circulation as hemoglobin F resists acid elution. Based on this testing, clinicians can determine if additional vials of Rh immune globulin should be administered in addition to the standard 300 mcg dosage for patients where the standard prophylactic dose of 300 mcg of anti-D immune globulin is not sufficient due to more blood being transferred from the fetus to the mother than usual (eg, antepartum hemorrhage and abdominal trauma).[3]
Additionally, dose adjustment may be considered based on gestational age in women who may have undergone a potential sensitization event, including miscarriage, ectopic pregnancy, termination procedures, or amniocentesis. Although recommendations vary, most experts have recommended 50 to 120 mcg of anti-D immune globulin for pregnancies of ≤12 weeks gestation and 300 mcg for gestations >12 weeks.[3]
Patient education regarding routine lab testing and Rh-D immunoprophylaxis in Rh-negative women is necessary to ensure appropriate interventions. For parents of newborns, education on the signs and symptoms of hyperbilirubinemia to assist the interprofessional team in the early identification of possible HDFN cases is essential. Furthermore, a critical aspect of HDFN management is the written and verbal education about neonatal jaundice to parents at discharge. Parents should be given clear instructions regarding follow-up appointments, laboratory studies, and clinical signs requiring medical attention.[4]
Enhancing Healthcare Team Outcomes
Enhancing interprofessional team outcomes for patients with HDFN necessitates collaborative efforts among OB/GYN and pediatric clinicians, nurses, pharmacists, and blood bank personnel. Recognizing the dual patient population comprising the mother and the fetus or newborn, teams must be well-prepared to identify signs and symptoms of HDFN, particularly in utero cases requiring timely transfusions at birth. Pharmacists are critical in assessing the need for Rh-D immunoprophylaxis to prevent future HDFN occurrences throughout pregnancy. The development of Rh-D immunoprophylaxis and newborn work-up protocols has significantly reduced HDFN incidence, highlighting the importance of sustained interprofessional collaboration to maintain this downward trend.
Consultation with maternal-fetal medicine specialists is paramount when HDFN is identified or suspected in utero, guiding management decisions such as intrauterine transfusions and optimal delivery timing. Continuous monitoring, including peak systolic velocity measurements and intrauterine transfusions, facilitates proactive management and mitigates potential complications. The optimal timing for delivery remains debated, balancing fetal survivability with the risks of prematurity and invasive procedures, necessitating individualized approaches based on disease severity and gestational age. Following delivery, neonatal management focuses on treating hyperbilirubinemia and anemia, with interventions ranging from phototherapy to exchange transfusions as guided by nomograms and serum bilirubin levels. Integrating evidence-based practices and ongoing interdisciplinary communication ensures comprehensive care delivery, optimizing patient outcomes while prioritizing patient safety and team performance.

Figure
Nomogram for Exchange Transfusions in Newborns Contributed by R Kabir, MD
References
- 1.
- Myle AK, Al-Khattabi GH. Hemolytic Disease of the Newborn: A Review of Current Trends and Prospects. Pediatric Health Med Ther. 2021;12:491-498. [PMC free article: PMC8504549] [PubMed: 34675752]
- 2.
- ACOG Practice Bulletin No. 192: Management of Alloimmunization During Pregnancy. Obstet Gynecol. 2018 Mar;131(3):e82-e90. [PubMed: 29470342]
- 3.
- Practice Bulletin No. 181: Prevention of Rh D Alloimmunization. Obstet Gynecol. 2017 Aug;130(2):e57-e70. [PubMed: 28742673]
- 4.
- Kemper AR, Newman TB, Slaughter JL, Maisels MJ, Watchko JF, Downs SM, Grout RW, Bundy DG, Stark AR, Bogen DL, Holmes AV, Feldman-Winter LB, Bhutani VK, Brown SR, Maradiaga Panayotti GM, Okechukwu K, Rappo PD, Russell TL. Clinical Practice Guideline Revision: Management of Hyperbilirubinemia in the Newborn Infant 35 or More Weeks of Gestation. Pediatrics. 2022 Sep 01;150(3) [PubMed: 35927462]
- 5.
- de Winter DP, Kaminski A, Tjoa ML, Oepkes D. Hemolytic disease of the fetus and newborn: systematic literature review of the antenatal landscape. BMC Pregnancy Childbirth. 2023 Jan 07;23(1):12. [PMC free article: PMC9824959] [PubMed: 36611144]
- 6.
- Naiman JL. On Dr. Louis K. Diamond's 1932 article and subsequent contributions to erythroblastosis fetalis. J Pediatr Hematol Oncol. 2001 Nov;23(8):550-3. [PubMed: 11878786]
- 7.
- Gabbay JM, Agneta EM, Turkington S, Bajaj BM, Sinha B, Geha T. Rates of phototherapy among ABO-incompatible newborns with a negative direct antiglobulin test. J Perinatol. 2023 Nov;43(11):1357-1362. [PMC free article: PMC10034253] [PubMed: 36959468]
- 8.
- Yu D, Ling LE, Krumme AA, Tjoa ML, Moise KJ. Live birth prevalence of hemolytic disease of the fetus and newborn in the United States from 1996 to 2010. AJOG Glob Rep. 2023 May;3(2):100203. [PMC free article: PMC10205505] [PubMed: 37229151]
- 9.
- Faye-Petersen OM, Heller DS. Pathology of the stillborn infant for the general pathologist: part 2. Adv Anat Pathol. 2015 Mar;22(2):71-93. [PubMed: 25664943]
- 10.
- Dumitru A, Gica N, Botezatu R, Peltecu G. Prognosis and Management in Subsequent Rh Alloimmunized Pregnancies. Maedica (Bucur). 2021 Dec;16(4):681-684. [PMC free article: PMC8897780] [PubMed: 35261671]
- 11.
- Moinuddin I, Fletcher C, Millward P. Prevalence and specificity of clinically significant red cell alloantibodies in pregnant women - a study from a tertiary care hospital in Southeast Michigan. J Blood Med. 2019;10:283-289. [PMC free article: PMC6708388] [PubMed: 31692490]
- 12.
- Nicolaides KH, Thilaganathan B, Rodeck CH, Mibashan RS. Erythroblastosis and reticulocytosis in anemic fetuses. Am J Obstet Gynecol. 1988 Nov;159(5):1063-5. [PubMed: 3189438]
- 13.
- Mitra S, Rennie J. Neonatal jaundice: aetiology, diagnosis and treatment. Br J Hosp Med (Lond). 2017 Dec 02;78(12):699-704. [PubMed: 29240507]
- 14.
- American Academy of Pediatrics Subcommittee on Hyperbilirubinemia. Management of hyperbilirubinemia in the newborn infant 35 or more weeks of gestation. Pediatrics. 2004 Jul;114(1):297-316. [PubMed: 15231951]
- 15.
- Murray NA, Roberts IA. Haemolytic disease of the newborn. Arch Dis Child Fetal Neonatal Ed. 2007 Mar;92(2):F83-8. [PMC free article: PMC2675453] [PubMed: 17337672]
- 16.
- Metcalf RA, Khan J, Andrews J, Mayock D, Billimoria Z, Pagano MB. Severe ABO Hemolytic Disease of the Newborn Requiring Exchange Transfusion. J Pediatr Hematol Oncol. 2019 Nov;41(8):632-634. [PubMed: 30044350]
- 17.
- Hendrickson JE, Delaney M. Hemolytic Disease of the Fetus and Newborn: Modern Practice and Future Investigations. Transfus Med Rev. 2016 Oct;30(4):159-64. [PubMed: 27397673]
- 18.
- Czernik C, Proquitté H, Metze B, Bührer C. Hydrops fetalis--has there been a change in diagnostic spectrum and mortality? J Matern Fetal Neonatal Med. 2011 Feb;24(2):258-63. [PubMed: 20446894]
- 19.
- Delaney M, Matthews DC. Hemolytic disease of the fetus and newborn: managing the mother, fetus, and newborn. Hematology Am Soc Hematol Educ Program. 2015;2015:146-51. [PubMed: 26637714]
- 20.
- Kurdi W. Non-immune fetal hydrops: Are we doing the appropriate tests each time? J Prenat Med. 2007 Jan;1(1):26-8. [PMC free article: PMC3309343] [PubMed: 22470821]
- 21.
- Nakayama A, Oshiro M, Yamada Y, Hattori T, Wakano Y, Hayashi S, Kokubo M, Takemoto K, Honda S, Ieda K, Yamamoto H, Kouwaki M, Yokoi K, Shinohara O, Kato T, Miyata M, Tanaka T, Hayakawa M. Prognostic factors of hydrops fetalis with pleural effusion. Pediatr Int. 2017 Oct;59(10):1053-1057. [PubMed: 28672054]
- 22.
- An X, Wang J, Zhuang X, Dai J, Lu C, Li X, Yan Y. Clinical Features of Neonates with Hydrops Fetalis. Am J Perinatol. 2015 Nov;32(13):1231-9. [PubMed: 26070120]
- 23.
- Ibrahim A, Dammas AS. Congenital neonatal chylothorax with hydrops fetalis treated with octreotide. Sudan J Paediatr. 2013;13(1):43-8. [PMC free article: PMC4949963] [PubMed: 27493357]
- 24.
- Dziegiel MH, Krog GR, Hansen AT, Olsen M, Lausen B, Nørgaard LN, Bergholt T, Rieneck K, Clausen FB. Laboratory Monitoring of Mother, Fetus, and Newborn in Hemolytic Disease of Fetus and Newborn. Transfus Med Hemother. 2021 Oct;48(5):306-315. [PMC free article: PMC8578801] [PubMed: 34803574]
- 25.
- Slootweg YM, Lindenburg IT, Koelewijn JM, Van Kamp IL, Oepkes D, De Haas M. Predicting anti-Kell-mediated hemolytic disease of the fetus and newborn: diagnostic accuracy of laboratory management. Am J Obstet Gynecol. 2018 Oct;219(4):393.e1-393.e8. [PubMed: 30063902]
- 26.
- Rieneck K, Egeberg Hother C, Clausen FB, Jakobsen MA, Bergholt T, Hellmuth E, Grønbeck L, Dziegiel MH. Next Generation Sequencing-Based Fetal ABO Blood Group Prediction by Analysis of Cell-Free DNA from Maternal Plasma. Transfus Med Hemother. 2020 Feb;47(1):45-53. [PMC free article: PMC7036558] [PubMed: 32110193]
- 27.
- Lee L, Nasser J. Doppler ultrasound assessment of fetal anaemia in an alloimmunised pregnancy. Australas J Ultrasound Med. 2010 Nov;13(4):24-27. [PMC free article: PMC5024878] [PubMed: 28191093]
- 28.
- Andrei C, Vladareanu R. The value of reference ranges for middle cerebral artery peak systolic velocity in the management of rhesus alloimmunized pregnancies. Maedica (Bucur). 2012 Jan;7(1):14-9. [PMC free article: PMC3484790] [PubMed: 23118814]
- 29.
- Jauniaux E. Diagnosis and management of early non-immune hydrops fetalis. Prenat Diagn. 1997 Dec;17(13):1261-8. [PubMed: 9509544]
- 30.
- Jensen KK, Oh KY, Patel N, Narasimhan ER, Ku AS, Sohaey R. Fetal Hepatomegaly: Causes and Associations. Radiographics. 2020 Mar-Apr;40(2):589-604. [PubMed: 32125959]
- 31.
- Swearingen C, Colvin ZA, Leuthner SR. Nonimmune Hydrops Fetalis. Clin Perinatol. 2020 Mar;47(1):105-121. [PubMed: 32000919]
- 32.
- Ree IMC, Smits-Wintjens VEHJ, van der Bom JG, van Klink JMM, Oepkes D, Lopriore E. Neonatal management and outcome in alloimmune hemolytic disease. Expert Rev Hematol. 2017 Jul;10(7):607-616. [PubMed: 28503958]
- 33.
- Li S, He Z, Mo C, Ji Y, Luo Y, Fang Q, Gao Y. Hyporegenerative anemia in anti-M-associated hemolytic disease of the fetus. Transfusion. 2021 Jun;61(6):1908-1915. [PubMed: 33938570]
- 34.
- Sahoo T, Thukral A, Sankar MJ, Gupta SK, Agarwal R, Deorari AK, Paul VK. Delayed cord clamping in Rh-alloimmunised infants: a randomised controlled trial. Eur J Pediatr. 2020 Jun;179(6):881-889. [PubMed: 31974670]
- 35.
- de Haas M, Thurik FF, Koelewijn JM, van der Schoot CE. Haemolytic disease of the fetus and newborn. Vox Sang. 2015 Aug;109(2):99-113. [PubMed: 25899660]
- 36.
- Hamza A, Herr D, Solomayer EF, Meyberg-Solomayer G. Polyhydramnios: Causes, Diagnosis and Therapy. Geburtshilfe Frauenheilkd. 2013 Dec;73(12):1241-1246. [PMC free article: PMC3964358] [PubMed: 24771905]
- 37.
- Derderian SC, Jeanty C, Fleck SR, Cheng LS, Peyvandi S, Moon-Grady AJ, Farrell J, Hirose S, Gonzalez J, Keller RL, MacKenzie TC. The many faces of hydrops. J Pediatr Surg. 2015 Jan;50(1):50-4; discussion 54. [PMC free article: PMC4315667] [PubMed: 25598092]
- 38.
- Smrcek JM, Baschat AA, Germer U, Gloeckner-Hofmann K, Gembruch U. Fetal hydrops and hepatosplenomegaly in the second half of pregnancy: a sign of myeloproliferative disorder in fetuses with trisomy 21. Ultrasound Obstet Gynecol. 2001 May;17(5):403-9. [PubMed: 11380964]
- 39.
- Sparks TN, Thao K, Lianoglou BR, Boe NM, Bruce KG, Datkhaeva I, Field NT, Fratto VM, Jolley J, Laurent LC, Mardy AH, Murphy AM, Ngan E, Rangwala N, Rottkamp CAM, Wilson L, Wu E, Uy CC, Valdez Lopez P, Norton ME., University of California Fetal–Maternal Consortium (UCfC). Nonimmune hydrops fetalis: identifying the underlying genetic etiology. Genet Med. 2019 Jun;21(6):1339-1344. [PMC free article: PMC6509016] [PubMed: 30410095]
- 40.
- Knilans TK. Cardiac abnormalities associated with hydrops fetalis. Semin Perinatol. 1995 Dec;19(6):483-92. [PubMed: 8822332]
- 41.
- Afifi AM, Bhatia AR, Eyal F. Hydrops fetalis associated with congenital myotonic dystrophy. Am J Obstet Gynecol. 1992 Mar;166(3):929-30. [PubMed: 1550167]
- 42.
- Narchi H. Congenital hypothyroidism and nonimmune hydrops fetalis: associated? Pediatrics. 1999 Dec;104(6):1416-7. [PubMed: 10610498]
- 43.
- Sun A. Lysosomal storage disease overview. Ann Transl Med. 2018 Dec;6(24):476. [PMC free article: PMC6331358] [PubMed: 30740407]
- 44.
- Leung KKY, Hon KL, Yeung A, Leung AKC, Man E. Congenital infections in Hong Kong: an overview of TORCH. Hong Kong Med J. 2020 Apr;26(2):127-138. [PubMed: 32245914]
- 45.
- Komvilaisak P, Komvilaisak R, Jetsrisuparb A, Wiangnon S, Jirapradittha J, Kiatchoosakun P, Fucharoen G. Fetal Anemia Causing Hydrops Fetalis From an Alpha-Globin Variant: Homozygous Hemoglobin Constant Spring. J Pediatr Hematol Oncol. 2018 Jul;40(5):405-408. [PubMed: 29240037]
- 46.
- Zwiers C, Lindenburg ITM, Klumper FJ, de Haas M, Oepkes D, Van Kamp IL. Complications of intrauterine intravascular blood transfusion: lessons learned after 1678 procedures. Ultrasound Obstet Gynecol. 2017 Aug;50(2):180-186. [PMC free article: PMC5601196] [PubMed: 27706858]
- 47.
- Wysowski DK, Flynt JW, Goldberg MF, Connell FA. Rh hemolytic disease. Epidemiologic surveillance in the United States, 1968 to 1975. JAMA. 1979 Sep 28;242(13):1376-9. [PubMed: 113567]
- 48.
- Pegoraro V, Urbinati D, Visser GHA, Di Renzo GC, Zipursky A, Stotler BA, Spitalnik SL. Hemolytic disease of the fetus and newborn due to Rh(D) incompatibility: A preventable disease that still produces significant morbidity and mortality in children. PLoS One. 2020;15(7):e0235807. [PMC free article: PMC7371205] [PubMed: 32687543]
Disclosure: Victoria Hall declares no relevant financial relationships with ineligible companies.
Disclosure: Elsa Vadakekut declares no relevant financial relationships with ineligible companies.
Disclosure: Indirapriya Darshini Avulakunta declares no relevant financial relationships with ineligible companies.
- Review History and current standard of postnatal management in hemolytic disease of the fetus and newborn.[Eur J Pediatr. 2023]Review History and current standard of postnatal management in hemolytic disease of the fetus and newborn.De Winter DP, Hulzebos C, Van 't Oever RM, De Haas M, Verweij EJ, Lopriore E. Eur J Pediatr. 2023 Feb; 182(2):489-500. Epub 2022 Dec 5.
- Review Hemolytic disease of the fetus and newborn with late-onset anemia due to anti-M: a case report and review of the Japanese literature.[Transfus Med Rev. 2014]Review Hemolytic disease of the fetus and newborn with late-onset anemia due to anti-M: a case report and review of the Japanese literature.Yasuda H, Ohto H, Nollet KE, Kawabata K, Saito S, Yagi Y, Negishi Y, Ishida A. Transfus Med Rev. 2014 Jan; 28(1):1-6. Epub 2013 Oct 19.
- Hemolytic disease of the fetus and newborn: systematic literature review of the antenatal landscape.[BMC Pregnancy Childbirth. 2023]Hemolytic disease of the fetus and newborn: systematic literature review of the antenatal landscape.de Winter DP, Kaminski A, Tjoa ML, Oepkes D. BMC Pregnancy Childbirth. 2023 Jan 7; 23(1):12. Epub 2023 Jan 7.
- Review Hemolytic Disease of the Fetus and Newborn: Historical and Current State.[Clin Lab Med. 2021]Review Hemolytic Disease of the Fetus and Newborn: Historical and Current State.Jackson ME, Baker JM. Clin Lab Med. 2021 Mar; 41(1):133-151. Epub 2020 Dec 24.
- Review Red Blood Cell Alloimmunization in the Pregnant Patient.[Transfus Med Rev. 2018]Review Red Blood Cell Alloimmunization in the Pregnant Patient.Webb J, Delaney M. Transfus Med Rev. 2018 Oct; 32(4):213-219. Epub 2018 Jul 19.
- Hemolytic Disease of the Fetus and Newborn - StatPearlsHemolytic Disease of the Fetus and Newborn - StatPearls
Your browsing activity is empty.
Activity recording is turned off.
See more...