This book is distributed under the terms of the Creative Commons Attribution-NonCommercial-NoDerivatives 4.0 International (CC BY-NC-ND 4.0) ( http://creativecommons.org/licenses/by-nc-nd/4.0/ ), which permits others to distribute the work, provided that the article is not altered or used commercially. You are not required to obtain permission to distribute this article, provided that you credit the author and journal.
NCBI Bookshelf. A service of the National Library of Medicine, National Institutes of Health.
StatPearls [Internet]. Treasure Island (FL): StatPearls Publishing; 2024 Jan-.
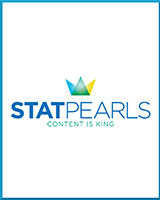
StatPearls [Internet].
Show detailsIntroduction
Lipopolysaccharides (LPS) are important outer membrane components of gram-negative bacteria. They are large amphipathic glycoconjugates that typically consist of a lipid domain (hydrophobic) attached to a core oligosaccharide and a distal polysaccharide. These molecules are also known as lipogylcans due to the presence of lipid and sugar molecules. The lipopolysaccharides are composed of:
- Lipid A: the hydrophobic domain, which is an endotoxin and the main virulence factor
- O-antigen, the repeating hydrophilic distal oligosaccharide
- The hydrophilic core polysaccharide
The lipid A component varies from one organism to another and is essential in imparting specific pathogenic attributes to the bacteria.[1] Inherent to gram-negative bacteria, LPS provides integrity to the bacterial cell and a mechanism of interaction of the bacteria to other surfaces.[2] Most bacterial LPS molecules are thermostable and generate a robust pro-inflammatory stimulus for the immune system in mammals. Since different types of LPS are present in different genera of gram-negative bacteria, LPS is used for serotyping gram-negative bacteria.[3] More specifically, the O-antigen imparts serological distinction to the bacterial species. Also, the size and composition of LPS are highly dynamic among bacterial species. Due to its unique properties, LPS has gained considerable research focus to understand its complex structure, biogenesis, transport, and assembly. Besides, LPS is also a recognized biomarker due to its central role in host-pathogen interaction that facilitates the infection process.
Fundamentals
The gram-negative bacterial cell membrane is composed of an outer membrane and an inner membrane. The outer membrane is exposed to the outside environment, while the inner membrane envelops the cytoplasm. Contrary to the usual biological membrane, the gram-negative bacterial membrane does not have a phospholipid bilayer but an asymmetric bilayer with LPS on the outside and phospholipid on the inside.[4] In non-capsulated bacterial strains, such as E.coli, Pseudomonas, etc., LPS is exposed to the cell surface, while in the capsulated strains such as Klebsiella pneumonia, Haemophilus influenza, etc., LPS is present below the capsular layer.
Of the three components, lipid A is the most bioactive component of LPS and a potent part of the endotoxin response generated by a molecule. Thus, LPS can be used for the early detection of infection since it induces an innate immune response, specifically through Toll-like receptors (TLRs). Even when the immune system causes lysis of the bacterial cells, lipid A containing fragments of the membrane released in the circulation cause fever, diarrhea, and in adverse circumstances, lead to septic (endotoxic) shock. Though lipid A moiety is a very conserved part of the LPS, the structure differs among different strains, species, and subspecies of bacteria.[5] Hence, the overall immune activation and response depend upon the structure of lipid A moiety of LPS. LPS recognition by the host is crucial for clearing the infections of invading bacterial pathogens. On the other hand, most gram-negative bacteria show innate resistance to many antimicrobial therapies due to the presence of LPS because it develops a permeability barrier at the cell surface.
In contrast to lipid A, O-antigens are the most variable part of the LPS molecule that imparts antigenic specificity to the molecule. The size or the composition of the O-antigen can reliably indicate the virulence potential of a bacterial strain. Modifications in the O-antigen plausibly play an essential role in the infection process. It mainly imparts the potential to induce attachment, colonization of the host, and the ability to circumvent host defense mechanisms.
Interestingly, canonical Lipid A, such as from non-invading E.coli, are speculated to be agonistic, while less conical lipid A, such as P. gingivalis, may activate a different signal (such as TLR4 instead of TLR2 and the strictly cylindrical lipid A, as Rhodobacter sphaeroides, tend to be antagonistic to TLRs. Hence, more pathogenic bacteria have evolved their lipid A moiety to evade host immune attacks.
Issues of Concern
LPS is a serologically reactive bacterial toxin, and as little as 1 to 2 mg entered intravenously can be lethal. LPS can enter the bloodstream through intestinal absorption of the LPS produced by gut bacteria. The LPS does not damage the gut epithelium of the luminal side. However, it can induce toxicity if it reaches the basal side, which has exposure to the deeper tissues. The body has developed compartmentalization to prevent high amounts of LPS from entering the bloodstream. However, gut lesions or a diet rich in lipids facilitate transport across the membrane into the systemic circulation.[6] LPS is a potent pyrogen for which the immune system mounts an immediate response. Many food products, supplements, and probiotics can pose a health risk as they contain gram-negative bacteria or LPS. Though these products are subject to digestion upon oral intake, it represents a health risk for patients with gastrointestinal disease.[7] Furthermore, LPS can enter the human system through pharmaceutical preparations such as parenteral drug products, which can activate the complement system by the alternative pathway and can result in the death of a patient.
A weak immune system is another breach in the barrier that leads to the endotoxic effects of LPS during an infection process. If allowed to progress unabated, it can lead to serious consequences such as septic shock or hypotension. Bacterial modifications in LPS structure, mostly the Lipid A portion, are a sophisticated strategy employed by Gram-negative bacteria to adapt to the host environment. Recently, there have been suggestions that certain types of autoimmune diseases and allergies may correlate with LPS. A large cohort study conducted on the fecal samples from North European infants indicates that the presence of Bacteroides species LPS is associated with higher levels of food allergy and anti-insulin antibodies, indicating early signs of immune dysfunction. On the other hand, high levels of bacteria from the genus Bifidobacterium correlates with the development of a healthy immune system. Thus, LPS from the gut may assist in the development of the immune system in infants.[8]
Besides lipid-binding protein (LBP), lipopolysaccharide-binding proteins are carriers for LPS in the blood. They can transfer LPS either to the macrophages or to the serum carrier lipoproteins (HDL and LDL). The transfer of LPS to macrophages initiates signal transduction to induce proinflammatory cytokines, while the delivery of LPS to HDL or LDL compromises the immune reaction against the infection. Additionally, the binding of LPS to lipoproteins induces dyslipidemia.[9][10]
Cellular Level
LPS, the major glycolipid of the outer membrane in gram-negative bacteria, is stabilized by divalent cations that increase the overall negative charge to the membrane. The LPS divides into three parts: Lipid A anchors the molecule to the outer membrane, the core oligosaccharide that is integral to imparting and maintaining membrane integrity, and the O-antigen polysaccharide that is connected to the core oligosaccharide as is in direct contact with the external environment.[4]
Overall, LPS structurally provides an effective permeability barrier against molecules that could be harmful to the bacterial cell. The LPS molecules only including lipid A and core oligosaccharides, are generally referred to as ‘rough’ and often called lipooligosaccharides, while the complete LPS capped with O antigen is called ‘smooth.’ The only gram-positive bacteria to contain an authentic lipopolysaccharide is Listeria monocytogenes.[11]
Most of the commensal and pathogenic gram-negative bacteria have been shown to form biofilms. These biofilms provide stability to the bacterial cell and resilience to these bacterial populations against various drugs and antibiotics. LPS modification through palmitoylation is one of the strategies that lead to stable biofilm formation. The bacterias such as E.coli and Pseudomonas aeruginosa display increased incorporation of palmitate acyl chain in the lipid A moiety. Palmitic acid imparts increased hydrophobicity to LPS, which is inherent to biofilms forming over both biotic and abiotic surfaces. Biofilms are refractory to drug therapy, and thus bacteria can develop a resistant phenotype in vivo. Biofilms also accumulate various nutrients, such as amino acids and antiadhesion molecules.[12] Biofilms also demonstrate increased tolerance to host immune responses, which enhance bacterial survival in vivo. Many hospital-acquired or resistant infections, such as catheter infections predominantly caused by Escherichia coli, Pseudomonas aeruginosa, Staphylococcus aureus, etc., show higher resistance to treatment due to their ability to form biofilms. Besides precluding host defense, the biofilm matrix also provides antimicrobial resistance by subduing antibiotic penetration and altering the host microenvironment.[12]
Molecular Level
LPS biogenesis employs the process of assembly at the bacterial inner membrane and subsequent translocation to the bacterial cell surface. The hydrophobic Lipid A part of the molecule is an acylated β-1‘-6-linked glucosamine disaccharide, which forms the outer leaflet of the outer membrane. These glucosamines are acylated at the 2, 3, 2’ and 3’ positions and phosphorylated at the 1 and 4’ positions, which typically gives a Hexa-acylated feature to the mature Lipid A. Hexacylated, bisphosphorylated lipid A as seen in E. coli and Salmonella is highly immunogenic than other forms of lipid A. Secondly, the core oligosaccharide is a non-repeating polymer linked to lipid A through the glucosamine moiety. It is inherently composed of 3-deoxy-D-manno-oct-2-ulosonic acid (Kdo) residues, various hexoses, and heptoses. In some bacterial strains, the sugars become substituted with phosphates, phosphoethanolamine, etc.[13] Thirdly, O-antigen is the most variable part of LPS imparting immunogenic properties to the LPS. It lies towards the inner leaflet of the outer membrane and is attached to the core polysaccharide. It is a repeating oligosaccharides unit of two to eight sugars. There are few bacterial strains, such as Neisseria, that do not synthesize O-antigen.[14] However, the presence of O-antigens is necessary for the adherence specificity of the bacteria to tissues.
The overall structure of the LPS is conserved. However, considerable structural differences lead to variations at the species and strain level. These variations are the basis of altered host immune response. Nonetheless, the host immune system has evolved to recognize LPS as a pathogen-associated molecular pattern (PAMP) and respond to it. The production of LPS and phospholipids are shared at the point of the common precursor, β-hydroxymyristate-ACP. FabZ utilizes the substrate in the phospholipid pathway and LpxA in the LPS biosynthetic pathway. Hence LPS has to be regulated so that proper balance remains in the synthesis of lipid and thus the development of inner and outer bacterial membranes. Since LpxC facilitated the first committed step in LPS biosynthesis, it is the control point for the pathway.[15]
Function
The primary function of LPS is to provide structural integrity and a permeability barrier to protect the bacterial cell from the entry of deleterious molecules such as toxins and bile salts during its inhabitation in the gastrointestinal tract. The presence of a large number of saturated fatty acid moieties leads to extensive interaction within the acyl chain, which results in low fluidity of the membrane bilayer. The high negativity of the membrane due to the presence of the phosphate group becomes stabilized by divalent cations such as Mg, which intercalate between LPS molecules. The final structure formed by polyionic interactions enhances LPS packing, finally making LPS a structural barrier to the bacterial cell.
Additionally, LPS is the primary component that imparts pathogenicity to the bacterial cell. Compared to the classical bacterial exotoxins, the endotoxins (LPS) are quite stable and are the primary biological response modifiers to induce specific symptoms and pathologies of the diseases. LPS exerts a potent and pleiotropic stimulus of host immune cells. The immune system recognizes the Lipid A component of LPS, which is released from the dividing cells in soluble form, from the lysed bacterial cells upon autolysis, or killing by complement activation or phagocytosis or by the effect of antibiotics. The primary response is through the identification and binding of the lipid A component to the TLR4 of the host cell. Since Lipid A is quite variable in its composition, different strains of bacteria mount different levels of the immune response. Lipid A produced by E. coli and Salmonella is highly immunogenic, while others, such as that of Yersinia pestis, modulate the extent of acylation of lipid A upon infection to produce LPS of low immunogenicity in vivo. Interestingly, synthesizing an LPS of low immunogenicity is a bacterial strategy to evade host immune response and increase intracellular survival.[3]
The O-antigen part of the LPS imparts antigenicity to the bacterial cell leading to the production of antibodies. Nonetheless, the variability in the length of the O-antigen chain can prevent complement-mediated bacterial controls and killing.[16] O antigen also contributes to pathogen evasion of phagocytosis by immune cells. Another essential function of LPS is in biofilm formation. The clustering of bacteria into a biofilm is induced by LPS that helps colonization and development of a drug-resistant phenotype that is refractory to most antibiotic therapies.
Mechanism
Inside the host system, the lipid A moiety of LPS is detected at picomolar levels by the receptor on the surface of macrophages and endothelial cells. Initially, LPS binds to the LBP in the serum, which transfers it to the CD14 receptor present on the cell membrane of the immune cells. The CD14 transfers it to the MD2 (a non-anchored protein), which interacts with Toll-like receptor-4 (TLR4). Thus, LPS binds with the CD14/TLR4/MD2 receptor complex present in many host cell types, such as monocytes, dendritic cells, macrophages, and B cells. The subsequent response depends on the cell type to which LPS is bound.[17] In monocyte and macrophages, three plausible responses, mentioned below, are triggered;
1. Production of cytokines like TNF, IL-1, IL-6, IL-8, which stimulates prostaglandins and leukotrienes release, finally leading to the inflammation and septic shock that are the significant features of endotoxemia.
2. Complement activation initiates histamine release causing vasodilation with neutrophil chemotaxis and accumulation.
3. Activation of coagulation cascade: Blood-clotting Factor XII activates humoral systems leading to coagulation, thrombosis, acute disseminated intravascular coagulation. Plasmin activation leads to fibrinolysis and hemorrhage; activation of the alternative pathway leads to inflammation. Activation of kinin releases bradykinins and other vasoactive peptides, causing hypotension. Finally, this induces the development of inflammation, hemorrhage, intravascular coagulation, and septic shock.
Testing
LPS is a virulence factor, and based on its structure and function, is used to classify bacteria in serogroups. Thus, it serves as a pathogen-specific biomarker to aid in serological discrimination of Gram-negative bacteria. Timely identification and characterization of pathotypes are essential for the early mitigation and treatment of infections. Being the primary immune stimulator is in host cells, it serves as an early indicator of acute infection. Thus, LPS testing is more specific and meaningful than other serological assays. The present methods of LPS testing are quite sensitive, but many cannot differentiate between LPS serogroups. Also, the amphiphilic property of LPS projects a critical bottleneck to the sensitivity and ease of use of the assays. The usual detection methods depend on lipid A antigen detection. However, LPS detection through lipid-A limits its ability to accurately identify a bacterial species because Lipid A is highly conserved among species and serotypes. The testing methods divide into six overlapping categories: in vivo and in vitro tests, modified immunoassays, biological assays, and chemical assays.[18]
Limulus Amoebocyte Lysate Assay
It utilizes the property of amoebocytes from Limuluspolyphemus to agglutinate upon the addition of endotoxin through a protease cascade reaction. The detection method uses the lysates of amoebocytes. Hence it was called the Limulus amoebocyte lysate (LAL) assay. LAL is the gold standard for the detection of lipid A. Nevertheless, this assay is subject to variability and inhibition through several chemical reactions. Variants of the LAL assay use chromogenic, turbidimetric, or viscometric methods to measure the degree of clotting. The sensitivity of this assay method depends on the sample type, processing method, time, and the dilution factor. The LAL assay is used in urine, cerebral spinal fluid, synovial fluid, ascites fluid, vaginal and cervical fluids, bronchoalveolar lavage samples, and even seawater.[19]
Biological and Chemical-based LPS Sensing
These technologies use biosensors that are activated with proteins or molecules to pull down LPS from a sample. The natural carriers for LPS, such as LBP or serum carrier proteins such as HDL and LDL, as well as synthetic aptamers, peptides, and metal-cation complex, are used to bind and pull down LPS in these assay methods. Different types of signals serve to detect and enhance the readout of the LPS signal. Electrochemical (EC) sensing requires a recognition ligand and a transducer to measure the variation in signal. Fluorescence-based sensing needs a receptor that captures LPS, and another molecule emits a fluorescent signal upon binding to the antigen. Aptamers attached to gold nanoparticles have been used to detect LPS using EIS. This technique has an enhanced detection limit of 0.1 pg/mL. Aptamers are also being used in a magnetic aptasensor to detect LPS. The use of lipid complexes (liposomes) has also been useful as a testing mechanism. This method manipulated the amphipathic nature of LPS. These biosensors provide high sensitivity, up to picogram or femtomolar range. However, these assays are incapable of discriminating between LPS serogroups.[20]
Immunoassays for LPS Detection and Antibody Selection
Enzyme-linked Immunosorbent assays (ELISA), based on the reaction between antigen and antibody, have been used to test LPS. However, the amphipathic nature of LPS leads to inconsistent binding on ELISA plates and variable conformations of epitope binding sites. This inconsistency resulted in low sensitivity and reproducibility of ELISA for LPS. There are two basic types of LPS-ELISAs, one which detects LPS antigen, and the other detects LPS antibody titers. An enhanced sandwich ELISA form that minimizes the low sensitivity and reproducibility associated with LPS-ELISA was developed and commercialized as ENDOLisa. It has a sensitivity between 0.05 and 500 EU/mL.
The second type of ELISA format is designed to detect antibodies against LPS. The plate surface is coated with the antigen to pull down antibodies such as IgA, IgG, etc., from serum. This method has its basis in testing the adaptive immune responses; hence it cannot be utilized to check the initial exposure to the pathogen. This type of ELISA format is useful to test population health risks and monitor epidemiology. The major drawback of LPS detection is that many LPS antigens have not been isolated yet. Thus screening methods are only present for the strains for which antigens are available. Alternative methods for antibody screening utilized immunoblotting and flow cytometry. Methods of LPS testing with the above techniques are affected by endogenous endotoxins present on glassware, plastics, or reagents, potentially contributing to false-positive results.[21]
In addition to medical diagnostics, LPS detection also provides a method for detecting Escherichia coli in the food industry, an organism often associated with food-borne illnesses. The successful detection methods for LPS couple sensitive detection platforms with surfaces designed to maximize the binding of amphiphilic PAMPs. Monitoring LPS levels in pharmaceutical products and medical devices is done using LAL assays.
Pathophysiology
LPS is a potent endotoxin that binds to cell surface receptors such as TLR4/CD14/MD2 that induces the secretion of proinflammatory cytokines, nitric oxide, and eicosanoids. The presence of LPS in the blood or interstitial fluid can lead to endotoxemia through Lipid A moiety, which can cause septic shock under exaggerated immune response. Septic shock includes tachycardia, tachypnea, temperature modulations, and coagulation cascade activation, leading to arterial and venous dilation.[22] The resulting hypovolemia leads to cellular dysfunction as a result of inadequate tissue perfusion. Recently, a low dose of LPS exposure has correlated with autoimmune diseases, and allergies while high concentrations of LPS in the blood lead to metabolic syndrome. This increases the risk of serious diseases such as type 2 diabetes, heart diseases, and liver diseases. Furthermore, endotoxins are inherently responsible for the clinical manifestations of infections with Gram-negative bacterial pathogens, such as Neisseria meningitides, that cause meningococcal disease, such as Waterhouse-Friderichsen syndrome, meningococcemia, and meningitis. Specific opportunistic pathogens like Pseudomonas aeruginosa, Burkholderia cepacia complex bacteria, Helicobacter pylori, and Salmonella enterica can adapt through LPS structure-function changes to develop a chronic infection in the respiratory and gastrointestinal tract.[3]
Recently, research has demonstrated that LPS induces membrane lipid disturbances, which affect cholesterol interacting proteins, lipoprotein metabolism, and membrane apo E/amyloid-beta interactions. These alterations predispose to hypercholesterolemia, dyslipidemia, and non-alcoholic fatty liver disease.[23] In some cases, the presence of LPS can interfere with the clearance of toxins from the body linking it to neurological degeneration.[24]
Clinical Significance
Diagnostic Marker: LPS can serve as an early diagnostic marker for infections. The presence of LPS in the serum, as low as 1 to 2 mg, can induce toxicity in the host mainly through the lipid A portion (the endotoxin). Endotoxin can induce symptoms of inflammation, fever, and leukopenia, and damage to blood vessels, finally leading to hypotension. High endotoxin can cause septicemia and shock.
Immunomodulation: LPS can help immune modulation in low doses by stimulating the polyclonal differentiation and multiplication of B-cells to secrete antibodies, especially IgG and IgM. In infants, LPS from the gut assists the development of the immune system. Bifidobacterium LPS is helpful during the development of a healthy immune system, while Bacteroides LPS demonstrates induction of food allergy and anti-insulin antibodies, an early sign of immune dysfunction.
Enhance the effect of Vaccines and Drugs: TLR activation is inducible by low levels of endotoxin, present in vaccines, and other immunotherapeutics, which provides improved immunization and drug effect, leading to better therapeutic outcomes. Low levels of LPS present in adjuvant can enhance their activity. Hence, the term for it is innate immune response modulation impurities (IIRMIs).[25]
Clinical Research: In clinical research, LPS preparations are useful for elucidating LPS biosynthesis, metabolism, immunology, physiology, and toxicity. LPS is also used to induce the synthesis and secretion of growth-promoting factors such as interleukins in in-vitro and in vivo research studies. Because of its direct function in causing septicemia, LPS has been studied to identify possible antibody targets and inhibitors.
References
- 1.
- Allen KN, Imperiali B. Structural and mechanistic themes in glycoconjugate biosynthesis at membrane interfaces. Curr Opin Struct Biol. 2019 Dec;59:81-90. [PMC free article: PMC6885101] [PubMed: 31003021]
- 2.
- Li Y, Shi Z, Radauer-Preiml I, Andosch A, Casals E, Luetz-Meindl U, Cobaleda M, Lin Z, Jaberi-Douraki M, Italiani P, Horejs-Hoeck J, Himly M, Monteiro-Riviere NA, Duschl A, Puntes VF, Boraschi D. Bacterial endotoxin (lipopolysaccharide) binds to the surface of gold nanoparticles, interferes with biocorona formation and induces human monocyte inflammatory activation. Nanotoxicology. 2017 Nov-Dec;11(9-10):1157-1175. [PubMed: 29192556]
- 3.
- Maldonado RF, Sá-Correia I, Valvano MA. Lipopolysaccharide modification in Gram-negative bacteria during chronic infection. FEMS Microbiol Rev. 2016 Jul;40(4):480-93. [PMC free article: PMC4931227] [PubMed: 27075488]
- 4.
- Sperandeo P, Martorana AM, Polissi A. Lipopolysaccharide Biosynthesis and Transport to the Outer Membrane of Gram-Negative Bacteria. Subcell Biochem. 2019;92:9-37. [PubMed: 31214983]
- 5.
- Steimle A, Autenrieth IB, Frick JS. Structure and function: Lipid A modifications in commensals and pathogens. Int J Med Microbiol. 2016 Aug;306(5):290-301. [PubMed: 27009633]
- 6.
- Boutagy NE, McMillan RP, Frisard MI, Hulver MW. Metabolic endotoxemia with obesity: Is it real and is it relevant? Biochimie. 2016 May;124:11-20. [PMC free article: PMC4695328] [PubMed: 26133659]
- 7.
- Wassenaar TM, Zimmermann K. Lipopolysaccharides in Food, Food Supplements, and Probiotics: Should We be Worried? Eur J Microbiol Immunol (Bp). 2018 Sep 28;8(3):63-69. [PMC free article: PMC6186019] [PubMed: 30345085]
- 8.
- Vatanen T, Kostic AD, d'Hennezel E, Siljander H, Franzosa EA, Yassour M, Kolde R, Vlamakis H, Arthur TD, Hämäläinen AM, Peet A, Tillmann V, Uibo R, Mokurov S, Dorshakova N, Ilonen J, Virtanen SM, Szabo SJ, Porter JA, Lähdesmäki H, Huttenhower C, Gevers D, Cullen TW, Knip M, DIABIMMUNE Study Group. Xavier RJ. Variation in Microbiome LPS Immunogenicity Contributes to Autoimmunity in Humans. Cell. 2016 Jun 02;165(6):1551. [PubMed: 27259157]
- 9.
- Opal SM, Scannon PJ, Vincent JL, White M, Carroll SF, Palardy JE, Parejo NA, Pribble JP, Lemke JH. Relationship between plasma levels of lipopolysaccharide (LPS) and LPS-binding protein in patients with severe sepsis and septic shock. J Infect Dis. 1999 Nov;180(5):1584-9. [PubMed: 10515819]
- 10.
- Mani V, Hollis JH, Gabler NK. Dietary oil composition differentially modulates intestinal endotoxin transport and postprandial endotoxemia. Nutr Metab (Lond). 2013 Jan 10;10(1):6. [PMC free article: PMC3577458] [PubMed: 23305038]
- 11.
- Casey A, Fox EM, Schmitz-Esser S, Coffey A, McAuliffe O, Jordan K. Transcriptome analysis of Listeria monocytogenes exposed to biocide stress reveals a multi-system response involving cell wall synthesis, sugar uptake, and motility. Front Microbiol. 2014;5:68. [PMC free article: PMC3937556] [PubMed: 24616718]
- 12.
- Valle J, Da Re S, Schmid S, Skurnik D, D'Ari R, Ghigo JM. The amino acid valine is secreted in continuous-flow bacterial biofilms. J Bacteriol. 2008 Jan;190(1):264-74. [PMC free article: PMC2223729] [PubMed: 17981982]
- 13.
- Klein G, Raina S. Regulated Control of the Assembly and Diversity of LPS by Noncoding sRNAs. Biomed Res Int. 2015;2015:153561. [PMC free article: PMC4651636] [PubMed: 26618164]
- 14.
- Iguchi A. [A complete view of the Escherichia coli O-antigen biosynthesis gene cluster and the development of molecular-based O-serogrouping methods]. Nihon Saikingaku Zasshi. 2016;71(4):209-215. [PubMed: 27980292]
- 15.
- Thomanek N, Arends J, Lindemann C, Barkovits K, Meyer HE, Marcus K, Narberhaus F. Intricate Crosstalk Between Lipopolysaccharide, Phospholipid and Fatty Acid Metabolism in Escherichia coli Modulates Proteolysis of LpxC. Front Microbiol. 2018;9:3285. [PMC free article: PMC6339880] [PubMed: 30692974]
- 16.
- Thiriard A, Raze D, Locht C. Diversion of complement-mediated killing by Bordetella. Microbes Infect. 2018 Oct-Nov;20(9-10):512-520. [PubMed: 29454132]
- 17.
- Tsukamoto H, Takeuchi S, Kubota K, Kobayashi Y, Kozakai S, Ukai I, Shichiku A, Okubo M, Numasaki M, Kanemitsu Y, Matsumoto Y, Nochi T, Watanabe K, Aso H, Tomioka Y. Lipopolysaccharide (LPS)-binding protein stimulates CD14-dependent Toll-like receptor 4 internalization and LPS-induced TBK1-IKKϵ-IRF3 axis activation. J Biol Chem. 2018 Jun 29;293(26):10186-10201. [PMC free article: PMC6028956] [PubMed: 29760187]
- 18.
- Fennrich S, Hennig U, Toliashvili L, Schlensak C, Wendel HP, Stoppelkamp S. More than 70 years of pyrogen detection: Current state and future perspectives. Altern Lab Anim. 2016 Jul;44(3):239-53. [PubMed: 27494624]
- 19.
- Neun BW, Dobrovolskaia MA. Detection of Endotoxin in Nano-formulations Using Limulus Amoebocyte Lysate (LAL) Assays. J Vis Exp. 2019 Jan 30;(143) [PubMed: 30774143]
- 20.
- Jackie J, Lau WK, Feng HT, Li SFY. Detection of Endotoxins: From Inferring the Responses of Biological Hosts to the Direct Chemical Analysis of Lipopolysaccharides. Crit Rev Anal Chem. 2019;49(2):126-137. [PubMed: 30821472]
- 21.
- Schenk F, Weber P, Vogler J, Hecht L, Dietzel A, Gauglitz G. Development of a paper-based lateral flow immunoassay for simultaneous detection of lipopolysaccharides of Salmonella serovars. Anal Bioanal Chem. 2018 Jan;410(3):863-868. [PubMed: 28971240]
- 22.
- Gomes JMG, Costa JA, Alfenas RCG. Metabolic endotoxemia and diabetes mellitus: A systematic review. Metabolism. 2017 Mar;68:133-144. [PubMed: 28183445]
- 23.
- Martins IJ. Overnutrition Determines LPS Regulation of Mycotoxin Induced Neurotoxicity in Neurodegenerative Diseases. Int J Mol Sci. 2015 Dec 10;16(12):29554-73. [PMC free article: PMC4691133] [PubMed: 26690419]
- 24.
- Vance JE. Dysregulation of cholesterol balance in the brain: contribution to neurodegenerative diseases. Dis Model Mech. 2012 Nov;5(6):746-55. [PMC free article: PMC3484857] [PubMed: 23065638]
- 25.
- Haile LA, Puig M, Kelley-Baker L, Verthelyi D. Detection of innate immune response modulating impurities in therapeutic proteins. PLoS One. 2015;10(4):e0125078. [PMC free article: PMC4406594] [PubMed: 25901912]
Disclosure: Aisha Farhana declares no relevant financial relationships with ineligible companies.
Disclosure: Yusuf Khan declares no relevant financial relationships with ineligible companies.
- Biochemistry, Lipopolysaccharide - StatPearlsBiochemistry, Lipopolysaccharide - StatPearls
Your browsing activity is empty.
Activity recording is turned off.
See more...