NCBI Bookshelf. A service of the National Library of Medicine, National Institutes of Health.
National Academies of Sciences, Engineering, and Medicine; Health and Medicine Division; Board on Health Sciences Policy; Forum on Neuroscience and Nervous System Disorders; Stroud C, Bain L, editors. Advancing Gene-Targeted Therapies for Central Nervous System Disorders: Proceedings of a Workshop. Washington (DC): National Academies Press (US); 2019 Sep 20.

Advancing Gene-Targeted Therapies for Central Nervous System Disorders: Proceedings of a Workshop.
Show details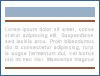
Box
Highlights.
Although most of the successful and unsuccessful studies discussed at this workshop focused on monogenic diseases, Chris Henderson suggested that going forward, it will be important to use learnings from these familial forms to find targets for treating sporadic and non-monogenic disorders. To achieve this goal while also advancing gene-targeted therapies for monogenic disorders will require technological innovation as well as attention to the safety of new approaches and a clearer understanding of which approaches are appropriate for specific diseases and targets, said Lamya Shihabuddin. Frances Jensen, professor and chair of neurology at the Perelman School of Medicine, University of Pennsylvania, added that it will also be important to consider how to coordinate implementing new discoveries in the clinic. The cancer field has been facing this challenge for decades, she said, as one new modality eclipses another. While trying to do what is best for patients by offering them the most advanced therapies, clinicians have at the same time had to consider the fact that there may be something even better in the pipeline, she said.
NEW TECHNOLOGIES ON THE HORIZON
As described in Chapter 3, viral vectors currently dominate the pipeline of gene-targeted therapies. Yet, Robert Kotin said that even as there are continuing efforts to find new and better vectors, other technologies are also advancing rapidly.
Non-Viral Methods
Kotin described a novel approach to gene transfer that delivers only the DNA of interest (the gene), flanked by inverted terminal repeats from the adeno-associated virus (AAV), that forms closed-end, linear duplex molecules (CELiD). Compared to bacterial plasmids, which have also been used for non-viral gene transfer, CELiD DNA has no prokaryotic modifications that might trigger an innate immune response and no endotoxins, said Kotin. He and his colleagues have produced close-ended DNA (ceDNA) constructs with the gene for green fluorescent protein or nuclear-localized b-galactosidase, and showed that following transfer by hemodynamic injection into the tail vein of mice, gene expression was constant over 7 days (Li et al., 2013). Adding a liver-specific promoter to the construct resulted in fairly constant gene expression in liver tissue over 10 weeks and essentially no change in CELiD copy number, said Kotin. More recently, investigators have demonstrated efficient transduction in the central nervous system (CNS) using ceDNA constructs delivered directly to rat brain using convection-enhanced delivery. One advantage of the ceDNA approach is that the vectors are produced using a good manufacturing practice-compatible process, said Kotin. However, optimizing the chemistry for specific tissue targeting remains a challenge in developing ceDNA methodologies, and it will not be possible to target the CNS with systemic delivery, he said.
Small Molecules to Modulate Splicing
Small molecules that modify post-transcriptional processing of RNA represent yet another novel therapeutic approach for genetic disorders, according to Anuradha Bhattacharyya of PTC Therapeutics, which has developed a platform to discover and develop small molecule splicing modifiers that are orally available and have broad tissue distribution. They are currently applying this technology to the development of treatments for spinal muscular atrophy (SMA), familial dysautonomia (FD), and Huntington's disease (HD), said Bhattacharyya.
The SMA therapeutic in development targets endogenous SMN2 with a small molecule splicing modifier that promotes the inclusion of exon 7, said Bhattacharyya. Two other strategies are also being pursued, she said. Each of these approaches provides many potential druggable targets, said Bhattacharyya. One approach corrects splicing mutations in pre-mRNA; this strategy is being used to enable inclusion of an exon that is usually skipped in the disease setting of FD. The other approach being pursued as a treatment for HD activates a pseudo-exon in the huntingtin gene pre-mRNA, which creates a premature stop codon, resulting in degradation of the mRNA and protein. In HD mouse models, Bhattacharyya said they have demonstrated a dose-dependent decrease in huntingtin protein across all relevant brain areas following oral delivery of the compound.
Nanoplatforms for Brain-Targeted Genome Editing
CRISPR/Cas9 genome editing, mentioned in Chapter 3, is a powerful technique enabling gene insertion, deletion, and alteration, said Shaoqin Sarah Gong, Vilas distinguished professor in biomedical engineering at the Wisconsin Institute of Discovery, University of Wisconsin–Madison. As part of the Somatic Cell Genome Editing Consortium established last year by the National Institutes of Health (NIH), Gong and colleagues are developing nanoplatforms to deliver Cas9 protein/single guided RNA (sgRNA) ribonucleoprotein complex (RNP) packaged into a nanocapsule. This approach, she said, allows precise control over the ratio of Cas9 and sgRNA within the RNP and enables rapid editing with a low incidence of off-target effects. Like other non-viral vector approaches, it also offers a good safety profile and versatile chemistry, and is easy to scale up, said Gong.
The nanocapsule, composed of a covalently cross-linked yet intracellularly biodegradable polymer coating, has a surface that can be conjugated with a wide array of targeting ligands, said Gong. It is taken up by specifically targeted cells through receptor-mediated endocytosis, she said, and once inside the target cell, the nanocapsule disintegrates and releases the RNP into the cytosol. The RNP is then transported to the nucleus, where it can carry out its editing of the targeted gene, she said. The nanocapsules achieve high editing efficiency even when freeze dried, which provides benefits in terms of purification, long-term storage, transportation, and dosage control, said Gong. She added that the nanocapsule is much less cytotoxic than Lipofectamine, a commercially available reagent often used to deliver DNA or RNA into cells.
Gong and colleagues have tested the efficiency of a nanocapsule targeting the human Alzheimer's precursor protein gene (APP) in cell culture, building on the work of her collaborator Subhojit Roy and colleagues, who recently used a CRISPR/Cas9 strategy to selectively silence APP through gene editing, suggesting that this may be an effective treatment modality (Sun et al., 2019). Gong and colleagues are also exploring other types of nanoplatforms to target different types of cells and deliver a wide range of hydrophilic payloads, including DNA, mRNA, proteins, and small-molecule drugs.
Novel Delivery Methods
Returning to the challenge of delivery, Junghae Suh, associate professor of bioengineering at Rice University, and Viviana Gradinaru, professor of neuroscience and biological engineering at the California Institute of Technology, addressed from the bioengineering perspective how delivery vectors can be engineered to be more efficient, more specific, and more controllable and how features of gene expression can be controlled.
Gradinaru provided some concrete examples from the field of protein engineering, where directed evolution has emerged as a means to engineer natural products for novel functionality. Working with Frances Arnold, who in 2018 won a Nobel Prize in chemistry (along with co-winners Gregory Winter and George Smith) for her development of the directed evolution approach, Gradinaru and colleagues generated thousands of variants of light-responsive proteins called opsins and then put them through very stringent selection criteria to get opsins that emit light in response to voltage, effectively turning them into voltage sensors (Flytzanis et al., 2014).
The same principles have also been applied to the development of improved viral vectors, said Gradinaru. Using directed evolution, she and her colleagues refined the properties of the AAV9 capsid to enable the virus to cross the blood–brain barrier in rodents, thus creating the PHP.B variant mentioned in Chapter 3 (Chan et al., 2017; Deverman et al., 2016). However, translating this from the rodent to non-human primate brain has proved difficult, in part because of the increased volume of the primate brain and cross-species variations, including different mechanisms to cross the blood–brain barrier, said Gradinaru. It becomes a numbers problem, she said, because meeting these challenges with directed evolution requires the generation of many offspring—as many as 1 billion or more depending on the molecule being targeted. Because no biological screen can fully sample this space, computational biologists, statisticians, and machine learning approaches are essential, she said. The concept is simple, but sounds like magic, she said: You feed training data into the computer, which spits out sequences that can be tested for the presence of desired characteristics.
Gradinaru said that once they had solved the challenge of crossing the blood–brain barrier in rodents, they encountered another challenge that required better opsins. Application of directed evolution and machine learning approaches to this problem yielded improved opsins for systemic delivery, which can be used for minimally invasive optogenetics (Bedbrook et al., in press). Now, in a parallel approach, they are applying directed evolution with deep sequencing to create variants that can achieve biodistribution to specific cell types such as endothelial cells in the vasculature or neurons.
Although the Parkinson's disease (PD) trial failures discussed by Jeffrey Kordower in Chapter 2 may have doomed glial-derived neurotrophic factor and neurturin as treatments for PD, they informed another approach that Kordower and colleagues are now pursuing: using intracellular antibodies (intrabodies) delivered with an AAV vector to clear α-synuclein (Chatterjee et al., 2018). The intrabodies are designed to bind to both monomeric and fibrillar forms of α-synuclein, prevent aggregation, and promote clearance. In a rat model of PD, direct delivery of the AAV-intrabody construct into the substantia nigra has resulted in improved motor behavior and increased intrastriatal dopamine, said Kordower. Next, they are hoping to use better vectors that will enable delivery throughout the brain. Steven Paul, chief executive officer of Karuna Pharmaceuticals, commented that a similar approach has been used to reduce tau pathology in mutant tau transgenic mice, and that this approach seemed to have superior efficacy in reducing tau-dependent neurodegeneration (Liu et al., 2016b).
DIVERSE EXPERTISE NEEDED TO TACKLE DELIVERY CHALLENGES
Protein engineering and directed evolution have made significant impacts on the development of better vectors to deliver cargo to a specific cell type, said Suh. The next challenge, she said, is to control the level of gene expression in the cargo. Other features of the expression profile that one might want to control include duration, periodicity, and response to the physiological state of the infected cell. To tackle this challenge, Suh advocated enlisting the help of synthetic biologists who apply their expertise in engineering control systems (i.e., inputs and outputs) to solve biological problems. For example, synthetic biologists have developed light-activatable transcriptional activators that optogenetically control gene expression (Olson et al., 2014) (see Figure 6-1). Suh said this group has gone on to demonstrate the multiplex control, that is, the ability to control the expression of two different genes in the same cells.

FIGURE 6-1
Optogenetic transcriptional control of gene expression. The input signal (light), represented by the dotted green line, shows the variation in light intensity over time; the corresponding output signal (green fluorescent protein, or GFP, expression) shows (more...)
The next challenge, said Suh, is deciding which genes to deliver for polygenic diseases. Fortunately, she said, systems biologists and computational biologists have already begun applying their expertise to the brain. What they do, she said, is apply data science tools to extract non-obvious patterns from complex datasets so that multipronged therapeutic approaches can be developed to treat complex diseases (Geschwind and Konopka, 2009).
To address the final challenge, administration of gene delivery vectors to the brain and spinal cord, Suh said scientists with expertise in computational fluid dynamics are needed. Through quantitative modeling of the transport of things (e.g., fluids, molecules, proteins, viruses) in complex environments, and applying these models to patient-derived imaging data, these scientists have been able to extract patient-specific three-dimensional maps of the vasculature, understand what blood flow patterns look like in that patient, and provide the information physicians need to decide how to treat the patient. Suh said that we now need to enlist these scientists to develop similar models that can elucidate how things move in and out of the CNS.
Suh added that identifying the synthetic biologists, computational biologists, and experts in computational fluid dynamics will not, by itself, be enough. Orchestrating a coherent effort, she said, will require a better structure and more connectors. In an orchestra, she noted, violins sit in one place and cellos in another, with all musical units clearly decoupled from one another. To create a symphony, they must have an organizational structure and a connector (the conductor) that enables them to work together. Similarly, to facilitate the robust solutions and pathways that will move gene therapy for CNS disorders forward, Suh said that connectors are needed who are skilled at forging links between disciplines and mediating effective communication.
Gradinaru added that help from the community and different disciplines would also help evolve vectors to bypass some of the challenges encountered in trying to develop designer AAVs for neuroscience that have the necessary cell type, circuit, organ, and/or region specificity; that can cross the blood–brain barrier or the placenta if intended to be delivered to embryos; and that are capable of carrying large cargo.
Cross-disciplinary collaborations are also needed to translate these new technologies from rodents to non-human primates, said Gradinaru. Because these experiments are difficult and resource intensive, the scientific community has been reluctant to test novel approaches in non-human primates, she said. “You rely on collaborators that are brave enough to test your rodent variants or that are generous enough to give you a few non-human primates to do these assays,” said Gradinaru. In an ongoing collaboration with investigators at the NIH/National Institute of Mental Health transgenic marmoset core, she and her colleagues have designed an experiment that tests pooled RNA-barcoded AAV9 variants in the monkeys. Although in its early days, this paradigm has the potential to efficiently, quickly, and cost-effectively screen many capsids in non-human primates, she said.
MOVING BEYOND MONOGENIC DISORDERS
As the examples presented in Chapter 2 illustrate, successful gene-targeted therapies for CNS disorders have thus far been restricted to rare monogenic disorders. However, Chapter 3 described progress in developing gene-targeted therapies for more common and complex polygenic disorders such as PD and Alzheimer's disease (AD) where the neuropathological underpinnings of the disease may not be fully understood. To determine which genes to target, Steven Hyman said there is no shortcut for basic biology. Genetic studies have pointed toward pathways and molecular complexes associated with elevated risk, he said, but to wisely select targets and think about a strategy for target validation one must first fully understand those pathways at a molecular level. Anastasia Khvorova added that translating an understanding of molecular mechanisms into new therapies will also require a clear understanding of how different targets interact with each other.
Developing Gene-Targeted Therapies of Psychiatric and Neurodevelopmental Disorders
Although scientists have begun to tackle complex polygenic disorders with gene therapy, they have not yet begun to apply this therapeutic approach to psychiatric disorders, said Hyman. He asserted that the time has come to think about this challenge while recognizing that clinical applications are a long way off.
Although neuropsychiatric disorders are highly heritable, all common neuropsychiatric disorders are highly polygenic, with phenotypes resulting from myriad small genetic nudges rather than a large genetic shove, said Hyman. He noted that this complicates efforts to identify which genes could be targeted. Moreover, he said, highly penetrant alleles that substantially elevate risk for early-onset behavioral disorders are quickly selected out of the gene pool because these highly disabling illnesses reduce the likelihood that an affected person will have children (Power et al., 2013). Large-effect alleles can occur de novo, but are rarely transmitted, while common and rare variants with low effect sizes can be readily transmitted, said Hyman.
Nonetheless, scientists have created animal models that replicate the human phenotype and have been able to reverse the phenotype by targeting specific genes, suggesting that gene therapy may be feasible. For example, Hyman cited the work of Adrian Bird and colleagues, who were able to reverse neurological defects in a mouse model of Rett syndrome (Guy et al., 2007).
Schizophrenia, however, is far more complex, said Hyman, with hundreds of genome-wide significant loci identified and extreme phenotypic heterogeneity (Huckins et al., 2019). He described a tool that could help scientists roughly stratify affected individuals at a genetic level by creating, for each individual, a weighted sum of risk alleles across the entire genome to produce a polygenic risk score (PRS). The PRS enables stratification of subjects by severity of genetic loading and permits identification of shared common variant risk across phenotypes, said Hyman. It can also help identify important genetic pathways and potentially identify targets for gene therapy interventions, he said. For example, about 70 percent of genome-wide association study (GWAS) hits in AD studies are expressed in microglia and are thought to be involved in inappropriate synapse elimination, said Hyman. He speculated that in schizophrenia, although none of the GWAS hits are expressed in microglia, there may be similar biological processes reached through different pathways such as genes encoding synaptic proteins and complement proteins that signal to microglia, adding that useful target selection can sometimes emerge from analysis of these pathways. What is important, he said, is to understand the biology. Transgenic animal models would require vastly improved methods of multiplexing using gene editing technologies.
The high degree of polygenicity seen in schizophrenia is also seen in common forms of neurodevelopmental disorders such as autism (Gaugler et al., 2014), said Hyman. Indeed, schizophrenia is considered to be neurodevelopmental in origin (Rund, 2018). A recent study by the Autism Sequencing Consortium (ASC) examining 36,000 exomes from affected and unaffected individuals identified 102 genes that are strongly associated with autism, said Joseph Buxbaum, director of the Seaver Autism Center for Research and Treatment at the Icahn School of Medicine at Mount Sinai in New York. Many of the gene mutations that have been identified are highly penetrant with serious deleterious impact and can be parsed into those that are autism specific and less autism specific, Buxbaum said.
Buxbaum summarized data from ASC and the Deciphering Developmental Disorders Study in the United Kingdom, which sequenced the genomes and phenotyped more than 4,000 individuals with severe developmental disorders (Deciphering Developmental Disorders Study, 2015). Together, he said, these studies and others have implicated multiple pathways. The genes discovered to date are overwhelmingly contributing to dominant disorders. The importance of a dominant disorder, said Buxbaum, is that there is a second allele that can be manipulated. He said most mutations identified to date result in a loss of function and are dose sensitive, typically displaying a U-shaped curve indicating that problems arise when there is either too much or too little gene expression. Most of the mutations result in severe conditions, he said, and in some cases re-expression of the gene later in life can be beneficial. For example, Guoping Feng and colleagues reported that in mouse models, mutations in SHANK3 result in synaptic dysfunction and autistic-like behaviors, but that re-expressing the gene in adult mice improved neural function and behavior, said Buxbaum (Mei et al., 2016).
Among the genes identified in these studies, those with the strongest findings are relatively common and highly penetrant, said Buxbaum. For example, SHANK3 haploinsufficiency explains about 0.5 percent of autism cases and 0.5 percent of intellectual disability cases, according to some studies (Betancur and Buxbaum, 2013), while other studies suggest an even higher association of SHANK3 with autism, he said. Loss of SHANK3 is called Phelan-McDermid syndrome (PMS), said Buxbaum. Taking PMS as one example, Buxbaum made the following points. Given that genetic testing can readily identify PMS; that it is thought to be fully penetrant with many potential biomarkers, rodent models, and even a primate model; and that there is an effective and engaged family foundation, Buxbaum suggested that this disorder may be an excellent target for gene therapy. Moreover, he said, a successful gene therapy for PMS might be repositioned to treat other forms of autism where there is no SHANK3 mutation, but where overlapping pathways are disrupted via other mechanisms. This is but one example, he said, suggesting there are already several dozen other known genes that are natural targets for gene therapy.
TARGETING NON-CODING RNAs
While most of the workshop discussions focused on genes that encode proteins, as much as 90 percent of genetic variants detected in GWAS are for non-coding genes, said Hyman. Only about one-third of those are known to be mapped to a particular gene, and even for those the directionality of their effect is mostly unknown, he said. But the fine mapping that is being achieved now may provide much of that information. Beverly Davidson challenged workshop participants to think about how these non-coding RNAs might be targeted, how target engagement might be assessed, and efficacy might be measured.
Khvorova suggested that non-coding RNA is as targetable as coding RNA. Modeling the expression of any of these genes can be accomplished with a panel of tools (e.g., animal models), she said, even when the mechanisms are unclear, she said. Hyman added that the non-coding portion of the genome may be the most evolvable and thus important in the context of developing gene-targeted therapies. Induced pluripotent stem cells, organoids, and xenotransplanted human neurons are increasingly being used in these studies, he said. Bhattarcharyya suggested that regulation of splicing may be particularly useful for targeting non-coding RNAs.
Abeliovich added that our growing knowledge of endogenous regulatory mechanisms may be leveraged in the development of gene therapeutics. For example, he said, a few examples of single-nucleotide polymorphisms appear to be regulatory and potentially disease modifying. A complicating issue, said Khvorova, is that many non-coding RNAs are only expressed in early developmental stages and not expressed or expressed at different levels or in different combinations in adults, adding complexity to data interpretation.
Sarah DeVos added that cells may also produce naturally occurring self-regulatory antisense that could potentially be targeted. Davidson said this is being attempted in some neonatal epilepsies caused by haploinsufficiency, which potentially could be blocked by targeting non-coding RNA that modulates that transcript.
POTENTIAL FOR PRE-COMPETITIVE COLLABORATION
Henderson commented that, while it is important that each company create its own intellectual property around specific programs, the large number of variables associated with gene-targeted therapies remains a significant challenge for individual companies. He suggested exploring the potential of pre-competitive partnerships to establish reproducible baseline data for standardized preparations of widely used capsids in primate models, including routes of administration and safety issues. He further suggested that although treating the adult brain will be “the next frontier,” this presents additional challenges not encountered in trials that enrolled infants and children. Ronald Crystal added standardizing assays for vectors to the list of issues that might be best addressed by pre-competitive partnerships. Hao Wang, global program leader for the CNS therapeutic area at Takeda Pharmaceuticals, noted that immune-related issues could also fall into potential collaborative activities.
Investing in Natural History Studies
To reach more diseases, particularly rare and ultrarare diseases, a collaborative platform approach starting with a natural history cohort is critical, added Petra Kaufmann. Such an approach could allow for the application of advanced and novel technologies and other resources to rare populations and provide recruitment registries and control populations, she said. Holly Tabor added that engaging with patients and patient communities can enable the building of natural history study databases. Rare disease groups and patients have long advocated for paying more attention to the natural history of their disorders, she said. Story Landis noted that academic groups and patient groups may not be aware of what is needed to make a natural history study useful for those who are conducting clinical trials.
Natural history studies are essential to developing compelling clinical trials, said Landis. Many single-gene defects are ripe for therapy development, she said, but in the absence of the natural history, selecting appropriate endpoints is not possible. Kaufmann agreed, noting that natural history studies should include “regulatory actionable” outcome measures. Kathleen Reape also noted that in ultrarare diseases, natural history data may be used as the control data. Indeed, in Chapter 2, Kaufmann described how natural history data were used in this way in the AVXS-101 trial, which led to the approval of a gene replacement therapy for SMA.
Landis said the National Institute of Neurological Disorders and Stroke (NINDS) has a clinical readiness funding program to support partnerships between academics and patient groups for natural history studies and identification of clinical endpoints. Walter Koroshetz, director of NINDS, reiterated Landis's comment, noting that NIH has funded several programs relevant to these proceedings, including not only natural history studies for nine different diseases, but other programs for biomarker validation and clinical trial readiness as well. They are also funding industry and disease collaborations to conduct clinical trials, he said. Frank Bennett added that having industry participation in the design of natural history studies is critical.
FINAL REMARKS
Treating CNS disorders by gene modification has become a reality in clinical applications, Shihabuddin said in her workshop wrap-up remarks. Moreover, the powerful platforms that have led to successful development of gene-targeted therapies for rare diseases have the potential to be tailored to target more common disorders, she said. Shihabuddin noted the many technical challenges that remain, including optimizing more efficient delivery to the correct brain regions and cell types, increasing the potency of antisense oligonucleotides and RNA interference chemistries, and designing bridging studies to accelerate moving from first generation to safer and more potent second generation vectors and other products.
Innovations in these and other areas may help to increase the efficacy of treatments and applicability to a broader range of diseases and may also drive down costs and improve patient access to treatments, said Shihabuddin. To address the challenges faced, she called for collaboration across academics, industry, NIH, regulators, and patient advocacy groups.
- Future Directions in the Development of Gene-Targeted Therapies - Advancing Gene...Future Directions in the Development of Gene-Targeted Therapies - Advancing Gene-Targeted Therapies for Central Nervous System Disorders
- PIWIL2 [Oryctolagus cuniculus]PIWIL2 [Oryctolagus cuniculus]Gene ID:100353983Gene
- LOC108425729 [Pygocentrus nattereri]LOC108425729 [Pygocentrus nattereri]Gene ID:108425729Gene
- Neurospora crassa ATPase proteolipid like gene and unidentified reading frame on...Neurospora crassa ATPase proteolipid like gene and unidentified reading frame on complementary strandgi|2938|emb|V00667.1|Nucleotide
- M.lini MldsB3 gene ORF1 and ORF2M.lini MldsB3 gene ORF1 and ORF2gi|2953|emb|X64371.1|Nucleotide
Your browsing activity is empty.
Activity recording is turned off.
See more...