This book is distributed under the terms of the Creative Commons Attribution-NonCommercial-NoDerivatives 4.0 International (CC BY-NC-ND 4.0) ( http://creativecommons.org/licenses/by-nc-nd/4.0/ ), which permits others to distribute the work, provided that the article is not altered or used commercially. You are not required to obtain permission to distribute this article, provided that you credit the author and journal.
NCBI Bookshelf. A service of the National Library of Medicine, National Institutes of Health.
StatPearls [Internet]. Treasure Island (FL): StatPearls Publishing; 2024 Jan-.
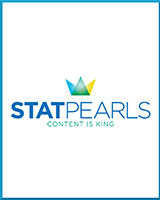
StatPearls [Internet].
Show detailsIntroduction
The primary function of the motor cortex is to send signals to direct the body's movement. The motor cortex is part of the frontal lobe and is anterior to the central sulcus. This region consists of the primary motor cortex, premotor cortex, and supplementary motor area.[1] Not all parts of the motor cortex have the granular cell layer. The primary motor cortex in Brodmann area 4 sends most electrical impulses from the motor cortex. These fibers synapse with spinal cord motor neurons (see Image. Motor Cortex). Corticospinal fibers originate both from the frontal cortex and also the parietal cortex. Anterior to the primary motor cortex, the premotor cortex is situated in Brodmann area 6. The function of the premotor cortex is to prepare for movement, especially proximal musculature. The supplementary motor area is in the medial surface of the longitudinal fissure, anterior to the area represented by the leg in the primary motor cortex. Although not fully understood, proposed functions include body postural stabilization and coordination.[2]
Significant differences exist in the projections from the cortical motor neurons. Fibers from the primary motor cortex (area 4) terminate mainly in the spinal cord, synapsing directly on motor neurons. The rostral frontal motor areas do not terminate directly at the spinal cord. They send fibers that terminate in various areas of the brainstem. Therefore, they control movements indirectly through the brainstem, such as the tectospinal and reticulospinal tracts. Accordingly, the projections control the proximal and axial muscles.
Cortical afferents to the frontal motor cortex arise from 3 sources: the parietal somatosensory cortex, the prefrontal cortex, and the cingulate cortex. The parietal-frontal connections represent different sensory-motor circuits. The cingulate cortex sends fibers to the premotor and supplemental motor areas for limbic information, motivation, and decision. Finally, the prefrontal cortex sends fibers to the motor cortex, leading to motor planning and execution.[3][4]
Issues of Concern
Weakness and paralysis resulting from motor cortex dysfunction can significantly reduce the quality of life in patients. Fortunately, some risk factors for stroke can be mitigated, such as hypertension, smoking, diabetes, poor diet, high cholesterol, and physical inactivity. Clinicians counsel patients regarding these factors to prevent severe neurologic harm.[5]
Cellular Level
The neuron is responsible for communication across the motor cortex at the cellular level. The neuron comprises 3 main parts: dendrites, soma, and axon (other neuron signals received by the dendrites). The dendrite then transmits this signal to the cell body or soma. The soma contains the nucleus and axon hillock. The nucleus creates all proteins and membranes that keep the neuron alive. The axon hillock is where the action potential is transmitted to the axon. The axon then delivers the action potential to the axon terminals, which synapse with the dendrites of other neurons. The motor cortex has somas that generate action potentials to the spinal cord, called the upper motor neurons (UMN). The UMNs communicate with the lower motor neuron (LMN) via glutamate.[6] The motor cortex has only a rudimentary layer IV that receives thalamocortical projections. Therefore, these areas are also called the agranular cortex, with no input from the thalamus.[7]
Development
The development of the central nervous system begins with the formation of the neural tube, which gives rise to the brain and spinal cord.[8] This process, known as neurulation, occurs during the third and fourth weeks of gestation. The neural tube differentiates into 3 primary brain vesicles: the prosencephalon (forebrain), mesencephalon (midbrain), and rhombencephalon (hindbrain). The prosencephalon further develops into the telencephalon and diencephalon, with the former giving rise to the cerebral hemispheres, including the motor cortex. In contrast, the diencephalon develops into the thalamus and hypothalamus. Following the formation of the different brain regions, neurons proliferate and migrate from the periventricular zone to the cortex inside-out to the final 6-layered cortical cytoarchitecture. The UMNs originate from layers 5 and 6 of the cerebral hemispheres, the primary motor cortex.[9]
When neurons have reached their positions within the developing motor cortex, they extend axons to establish connections with target neurons. Axon guidance cues play a crucial role in directing the growth of these axons toward their appropriate targets, including the spinal cord and other brain regions involved in motor control. Synaptic connections form between neurons, facilitating communication within the motor circuitry. The motor cortex undergoes further maturation and refinement throughout late prenatal development and early postnatal life. This process involves pruning excess connections, strengthening functional circuits through activity-dependent mechanisms, and fine-tuning neural circuits to support precise motor control.[10]
Organ Systems Involved
The musculoskeletal system relies on the motor pathway. The LMN synapses with the muscle fiber at the neuromuscular junction after the action potential reaches the axon terminal of the LMN. Following, voltage-gated calcium channels open to increase intracellular calcium ion concentrations, allowing acetylcholine vesicles to fuse with the membrane and be released into the synaptic cleft to produce muscle contractions.
Skeletal Muscle System
The primary function of the motor cortex is to control skeletal muscle movements. The motor cortex sends signals via descending pathways, such as the corticospinal tract, to the spinal cord, where motor neurons innervate skeletal muscles, initiating and modulating voluntary movements. Fine motor control, such as precise finger movements or complex limb actions, mainly depends on the motor cortex's intricate organization and precise neural coding.[11]
Motor Coordination and Balance
The motor cortex coordinates movements across multiple joints and muscle groups to achieve smooth and purposeful actions. This coordination extends beyond individual muscles to include the synchronization of muscle groups involved in complex tasks like walking, running, or reaching. Additionally, the motor cortex interacts with other brain regions, including the cerebellum and basal ganglia, to integrate sensory feedback and maintain balance and posture during movement.
Speech and Language Production
Specific regions of the motor cortex, particularly in the dominant hemisphere, produce speech and language. The Broca area in the left frontal lobe is crucial for speech-motor integration to articulate words and sentences. Damage to this area can result in expressive aphasia, impairing the ability to produce fluent speech while leaving comprehension intact.
Facial Expressions
The motor cortex controls the muscles of the face, allowing for a wide range of facial expressions that convey emotions and social cues. Fine control of facial muscles, such as those involved in smiling, frowning, or raising eyebrows, is mediated by cortical areas dedicated to facial motor control. These regions are interconnected with limb motor areas and are essential for non-verbal communication.
Hand and Finger Movements
A significant portion of the motor cortex is devoted to controlling hand and finger movements, reflecting the importance of manual dexterity in human behavior. The precise organization of the motor cortex facilitates intricate finger movements required for tasks such as typing, writing, playing musical instruments, and manipulating objects precisely.
Eye Movements
While specialized brainstem nuclei primarily control eye movements, the motor cortex contributes to certain aspects of voluntary eye movements, particularly those involved in visual tracking and saccadic eye movements. Cortical regions, such as the frontal eye fields, play a role in directing gaze and coordinating eye movements with head and body orientation.
Function
The primary function of the motor cortex is to plan and create electrical impulses that cause voluntary muscle contractions. The nerves from the motor cortex are cranial or spinal. Cranial nerves are derived from the brainstem, whereas spinal nerves emerge from the spinal cord. Several cranial nerves (CN) have a motor function, including CN III (oculomotor nerve), CN IV (trochlear nerve), CN V (trigeminal nerve), CN VI (abducens nerve), CN VII (facial nerve), CN IX (glossopharyngeal nerve), CN X (vagus nerve), CN XI (accessory nerve), and CN XII (hypoglossal nerve). CN III, IV, and VI are involved in eye movement. CN V innervates the muscles of mastication. CN VII causes movement of the facial muscles. CN IX innervates the stylopharyngeus muscle, causing pharyngeal and laryngeal movement. CN X has many functions in speaking and swallowing. CN XI involves shoulder shrugging and head-turning with innervation of the trapezius and sternocleidomastoid. CN XII innervates most muscles of the tongue.[12]
Primarily, the supplementary motor area projects to the motor cortex. The area appears to be involved in motor sequencing. Any lesions of this area in monkeys produce awkwardness in performing complex activities with bimanual coordination. When humans count to themselves without speaking, the motor cortex is quiescent. However, when they declare the number out loud, blood flow increases in the motor cortex and the supplementary motor area. Furthermore, the supplementary motor area and the motor cortex are involved in voluntary movements when the movements being performed are complex and require planning. The blood flow increases whether or not a planned movement is carried out. The increase in blood flow occurs when the contralateral or the ipsilateral hand performs the movement.[13]
Mechanism
The execution of motor functions by the brain motor cortex involves a sophisticated network of neural pathways or tracts that transmit signals between the cortex and the spinal cord and other brain regions involved in motor control. These pathways are essential for initiating, modulating, and coordinating voluntary movements throughout the body.[14]
Corticospinal Tract
This is the primary pathway through which motor commands from the motor cortex reach the spinal cord.[15] The UMN originates from the pyramidal cells of the primary motor cortex and other motor areas in the frontal lobe. Corticospinal tract fibers descend through the ipsilateral posterior limb of the internal capsule, cerebral peduncle, and brainstem, eventually crossing over to the contralateral side of the brainstem at the medullary pyramids (pyramidal decussation). Once crossed, the fibers descend into the lateral or anterior corticospinal tract, depending on the target muscles they innervate. In the spinal cord, corticospinal fibers synapse with LMNs in the ventral horn, connecting the motor cortex and skeletal muscles.[16]
Corticobulbar Tract
This tract conveys motor signals from the motor cortex to the brainstem nuclei that control head and neck muscles. The tract originates from the same cortical regions as the corticospinal tract and descends through the internal capsule alongside corticospinal fibers. Unlike the corticospinal tract, most corticobulbar fibers terminate bilaterally in the brainstem, allowing for bilateral control of facial and head muscles.
Extrapyramidal Tract
In addition to the corticospinal and corticobulbar tracts, extrapyramidal tracts originate from various subcortical nuclei, such as the basal ganglia and cerebellum, and indirectly influence motor function. The extrapyramidal tracts include the rubrospinal tract, reticulospinal tract, tectospinal tract, and vestibulospinal tract. These tracts modulate and refine motor commands generated by the cortex, contribute to postural control, and regulate involuntary movements such as reflexes and locomotion.
Related Testing
Neurologic testing to assess the functions of the motor cortex involves various clinical techniques to evaluate motor control, coordination, strength, and other aspects of voluntary movement. These tests are critical for diagnosing neurologic conditions affecting the motor cortex, such as strokes, tumors, and neurodegenerative diseases.
Motor Examination
The exam begins with assessing muscle tone, strength, and bulk in various muscle groups. Muscle tone is evaluated by passive manipulation of limbs to detect abnormalities such as spasticity (increased tone) or flaccidity (decreased tone). Muscle strength is assessed using manual muscle testing or dynamometry, where the patient is asked to resist against applied force in different muscle groups. Specific movements, such as flexion, extension, abduction, and adduction, are tested on individual joints to assess muscle strength and coordination.
Reflex Testing
Testing for an intact motor cortical pathway can involve deep tendon reflex testing. The biceps (C5, C6), brachioradialis (C6), triceps (C7), patellar (L4), and Achilles tendon (S1) can be tested to assess intact nerve roots.[17] When stretched, the muscle spindle sends a signal down the 1 alpha nerve fiber. The fiber enters the spinal column via the dorsal root and synapses with a LMN and interneuron. The LMN will cause the agonist muscle to contract, while the interneuron will cause the antagonist muscle to relax.[18]
Coordination Testing
These tests assess the patient's ability to perform precise and coordinated movements. The testing can help localize lesions affecting motor coordination.
- Finger-to-nose test: The patient is asked to touch their nose with their index finger and then touch the examiner's finger held at varying distances and positions.
- Rapid alternating movements: The patient is instructed to rapidly alternate between pronation and supination of the forearm or tapping the palm and dorsum of the hand.
- Heel-to-shin test: When sitting or lying down, the patient is asked to run the heel of one foot down the shin of the opposite leg.
Gait Assessment
Observing the patient's walking pattern, including stride length, step symmetry, arm swing, and heel-to-toe coordination, can reveal abnormalities indicative of motor cortex dysfunction. Additional tests, such as tandem gait (walking heel-to-toe in a straight line) and the Romberg test (standing with feet together and eyes closed), may be performed to assess balance and proprioception.
Pronator Drift Test
The patient extends their arms with palms facing up and eyes closed. Drifting or pronation of one arm may indicate subtle weakness or corticospinal tract dysfunction.[19]
Imaging and Electrophysiologic Studies
Neuroimaging techniques, such as magnetic resonance imaging (MRI) and computed tomography (CT), may be used to visualize structural abnormalities in the brain, including lesions affecting the motor cortex.[20] Electrophysiologic studies, such as electromyography (EMG) and nerve conduction studies, can assess the integrity of peripheral nerves and neuromuscular junctions, localizing motor deficits.
Pathophysiology
Stroke
A stroke occurs when a portion of the brain does not receive sufficient blood. As a result, stroke symptoms can manifest as motor function deficits should parts of the motor cortex be infarcted.[21] However, this often presents other symptoms, such as speech or vision deficits. A pure motor stroke is possible and is symptomatic of a lacunar stroke, causing an infarct of the posterior limb of the internal capsule. This infarct can present with hemiparesis or weakness of 1 side of the body.[22]
Multiple Sclerosis
Multiple sclerosis (MS) is a chronic autoimmune demyelinating disease of the central nervous system characterized by the formation of inflammatory plaques, axonal damage, and subsequent neurological dysfunction. In the context of UMN lesions related to the brain motor cortex, MS primarily affects the corticospinal tract and other descending pathways originating from the motor cortex. In MS, immune-mediated inflammation destroys myelin sheaths surrounding axons, disrupting signal conduction and impairing motor function.[23] Demyelination and axonal loss within the motor cortex and the descending projections result in a range of UMN signs, including weakness, spasticity, hyperreflexia, and impaired fine motor control.
Amyotrophic Lateral Sclerosis
The degeneration of upper and lower motor neurons characterizes this progressive neurodegenerative disease. The pathophysiology involves genetic mutations, protein aggregation, oxidative stress, excitotoxicity, and neuroinflammation, leading to selective vulnerability and degeneration. Degeneration of UMNs within the motor cortex results in characteristic signs, including muscle weakness, spasticity, hyperreflexia, and, eventually, paralysis. Furthermore, as the disease progresses, degeneration spreads to LMNs in the spinal cord and brainstem, leading to muscle atrophy, fasciculations, and, ultimately, respiratory failure.[24]
Tetanus
Tetanus is a condition caused by the exotoxin tetanospasmin from Clostridium tetani. Tetanus causes painful muscle contractions, especially in the jaw and neck. Tetanospasmin inhibits the release of glycine and GABA, which are inhibitory neurotransmitters. Without inhibition of the skeletal muscles, sustained contraction occurs.
Clinical Significance
Damage to the motor cortex results in a clinical condition called UMN syndrome. This can also arise due to damage to the axons from the motor cortex (ie, the corticospinal tract). Clinically, weakness without significant muscle wasting, spasticity (increased muscle tone with clasp-knife rigidity), hyperreflexia, and positive Babinski sign would occur. Babinski sign is a normal reflex in infants but abnormal in adults. The reflex occurs when the big toe moves upward after the sole is stroked firmly.[25]
Clasp knife rigidity or spasticity characteristically presents an initial resistance in movement followed by a rapid decrease in resistance. UMN lesions present a pattern of weakness, with less weakness affecting the anti-gravity muscles. This pattern gives rise to the familiar appearance after a stroke with damage to the motor cortex. The patient will have a flexed posture in the affected upper extremity and an extended posture in the lower extremity. The patient will walk with a typical hemiparetic gait. LMN lesions arise from the damage of the motor neuron in the spinal cord and characteristically present with weakness, atrophy, fasciculations, hyporeflexia, decreased tone, and flaccid paralysis.

Figure
Motor Cortex. The primary motor cortex in Brodmann area 4 sends most electrical impulses from the motor cortex. These fibers directly synapse with motor neurons of the spinal cord. Contributed by S Bhimji, MD
References
- 1.
- Meier JD, Aflalo TN, Kastner S, Graziano MS. Complex organization of human primary motor cortex: a high-resolution fMRI study. J Neurophysiol. 2008 Oct;100(4):1800-12. [PMC free article: PMC2576195] [PubMed: 18684903]
- 2.
- He SQ, Dum RP, Strick PL. Topographic organization of corticospinal projections from the frontal lobe: motor areas on the medial surface of the hemisphere. J Neurosci. 1995 May;15(5 Pt 1):3284-306. [PMC free article: PMC6578253] [PubMed: 7538558]
- 3.
- Bhattacharjee S, Kashyap R, Abualait T, Annabel Chen SH, Yoo WK, Bashir S. The Role of Primary Motor Cortex: More Than Movement Execution. J Mot Behav. 2021;53(2):258-274. [PubMed: 32194004]
- 4.
- Svoboda K, Li N. Neural mechanisms of movement planning: motor cortex and beyond. Curr Opin Neurobiol. 2018 Apr;49:33-41. [PubMed: 29172091]
- 5.
- de Brouwer EJM, Kockelkoren R, De Vis JB, Dankbaar JW, Velthuis BK, Takx RA, De Jonghe A, Emmelot-Vonk MH, Koek HL, de Jong PA., Dutch acute stroke study investigators (DUST). Prevalence and vascular risk factors of basal ganglia calcifications in patients at risk for cerebrovascular disease. J Neuroradiol. 2020 Sep;47(5):337-342. [PubMed: 31034898]
- 6.
- de Carvalho M. Pathophysiological significance of fasciculations in the early diagnosis of ALS. Amyotroph Lateral Scler Other Motor Neuron Disord. 2000 Mar;1 Suppl 1:S43-6. [PubMed: 11464925]
- 7.
- Nolan M, Scott C, Hof PR, Ansorge O. Betz cells of the primary motor cortex. J Comp Neurol. 2024 Jan;532(1):e25567. [PMC free article: PMC10952528] [PubMed: 38289193]
- 8.
- Hadders-Algra M. Early human brain development: Starring the subplate. Neurosci Biobehav Rev. 2018 Sep;92:276-290. [PubMed: 29935204]
- 9.
- Stiles J, Jernigan TL. The basics of brain development. Neuropsychol Rev. 2010 Dec;20(4):327-48. [PMC free article: PMC2989000] [PubMed: 21042938]
- 10.
- Darnell D, Gilbert SF. Neuroembryology. Wiley Interdiscip Rev Dev Biol. 2017 Jan;6(1) [PMC free article: PMC5193482] [PubMed: 27906497]
- 11.
- de Carvalho M, Swash M. Upper and lower motor neuron neurophysiology and motor control. Handb Clin Neurol. 2023;195:17-29. [PubMed: 37562869]
- 12.
- Davis MC, Griessenauer CJ, Bosmia AN, Tubbs RS, Shoja MM. The naming of the cranial nerves: a historical review. Clin Anat. 2014 Jan;27(1):14-9. [PubMed: 24323823]
- 13.
- Shibata S, Yamao Y, Kunieda T, Inano R, Nakae T, Nishida S, Inada T, Takahashi Y, Kikuchi T, Arakawa Y, Yoshida K, Matsumoto R, Ikeda A, Mima T, Miyamoto S. Intraoperative Electrophysiologic Mapping of Medial Frontal Motor Areas and Functional Outcomes. World Neurosurg. 2020 Jun;138:e389-e404. [PubMed: 32145417]
- 14.
- Lee C, Kim Y, Kaang BK. The Primary Motor Cortex: The Hub of Motor Learning in Rodents. Neuroscience. 2022 Mar 01;485:163-170. [PubMed: 35051529]
- 15.
- Welniarz Q, Dusart I, Roze E. The corticospinal tract: Evolution, development, and human disorders. Dev Neurobiol. 2017 Jul;77(7):810-829. [PubMed: 27706924]
- 16.
- Lemon RN. Descending pathways in motor control. Annu Rev Neurosci. 2008;31:195-218. [PubMed: 18558853]
- 17.
- Lees AJ, Hurwitz B. Testing the reflexes. BMJ. 2019 Aug 14;366:l4830. [PubMed: 31412997]
- 18.
- Hofstoetter US, Danner SM, Freundl B, Binder H, Mayr W, Rattay F, Minassian K. Periodic modulation of repetitively elicited monosynaptic reflexes of the human lumbosacral spinal cord. J Neurophysiol. 2015 Jul;114(1):400-10. [PMC free article: PMC4507962] [PubMed: 25904708]
- 19.
- Weaver DF. Rotator drift: A sign of upper motor neuron leg weakness. Clin Neurol Neurosurg. 2020 Oct;197:106084. [PubMed: 32683196]
- 20.
- Madkhali Y, Aldehmi N, Pollick F. Functional Localizers for Motor Areas of the Brain Using fMRI. Comput Intell Neurosci. 2022;2022:7589493. [PMC free article: PMC9167083] [PubMed: 35669664]
- 21.
- Chen X, Li M, Chen N, Lai H, Huang Z, Tu Y, Chen Q, Hu J. Structural Covariance of the Ipsilesional Primary Motor Cortex in Subcortical Stroke Patients with Motor Deficits. Neural Plast. 2022;2022:1460326. [PMC free article: PMC8930265] [PubMed: 35309255]
- 22.
- Khan A, Kasner SE, Lynn MJ, Chimowitz MI., Warfarin Aspirin Symptomatic Intracranial Disease (WASID) Trial Investigators. Risk factors and outcome of patients with symptomatic intracranial stenosis presenting with lacunar stroke. Stroke. 2012 May;43(5):1230-3. [PMC free article: PMC3336041] [PubMed: 22363054]
- 23.
- Bergsland N, Laganà MM, Tavazzi E, Caffini M, Tortorella P, Baglio F, Baselli G, Rovaris M. Corticospinal tract integrity is related to primary motor cortex thinning in relapsing-remitting multiple sclerosis. Mult Scler. 2015 Dec;21(14):1771-80. [PubMed: 25791368]
- 24.
- Masrori P, Van Damme P. Amyotrophic lateral sclerosis: a clinical review. Eur J Neurol. 2020 Oct;27(10):1918-1929. [PMC free article: PMC7540334] [PubMed: 32526057]
- 25.
- Acharya AB, Jamil RT, Dewey JJ. StatPearls [Internet]. StatPearls Publishing; Treasure Island (FL): Jan 1, 2023. Babinski Reflex. [PubMed: 30085551]
Disclosure: Derek Yip declares no relevant financial relationships with ineligible companies.
Disclosure: Ayoola Awosika declares no relevant financial relationships with ineligible companies.
Disclosure: Forshing Lui declares no relevant financial relationships with ineligible companies.
- The origin of corticospinal projections from the premotor areas in the frontal lobe.[J Neurosci. 1991]The origin of corticospinal projections from the premotor areas in the frontal lobe.Dum RP, Strick PL. J Neurosci. 1991 Mar; 11(3):667-89.
- A comparison of the ipsilateral cortical projections to the dorsal and ventral subdivisions of the macaque premotor cortex.[Somatosens Mot Res. 1995]A comparison of the ipsilateral cortical projections to the dorsal and ventral subdivisions of the macaque premotor cortex.Ghosh S, Gattera R. Somatosens Mot Res. 1995; 12(3-4):359-78.
- Prosencephalic afferents to the mediodorsal thalamic nucleus.[J Comp Neurol. 1985]Prosencephalic afferents to the mediodorsal thalamic nucleus.Velayos JL, Reinoso-Suárez F. J Comp Neurol. 1985 Dec 8; 242(2):161-81.
- Review Motor areas in the frontal lobe of the primate.[Physiol Behav. 2002]Review Motor areas in the frontal lobe of the primate.Dum RP, Strick PL. Physiol Behav. 2002 Dec; 77(4-5):677-82.
- Review Motor areas on the medial wall of the hemisphere.[Novartis Found Symp. 1998]Review Motor areas on the medial wall of the hemisphere.Strick PL, Dum RP, Picard N. Novartis Found Symp. 1998; 218:64-75; discussion 75-80, 104-8.
- Physiology, Motor Cortical - StatPearlsPhysiology, Motor Cortical - StatPearls
Your browsing activity is empty.
Activity recording is turned off.
See more...