This book is distributed under the terms of the Creative Commons Attribution-NonCommercial-NoDerivatives 4.0 International (CC BY-NC-ND 4.0) ( http://creativecommons.org/licenses/by-nc-nd/4.0/ ), which permits others to distribute the work, provided that the article is not altered or used commercially. You are not required to obtain permission to distribute this article, provided that you credit the author and journal.
NCBI Bookshelf. A service of the National Library of Medicine, National Institutes of Health.
StatPearls [Internet]. Treasure Island (FL): StatPearls Publishing; 2024 Jan-.
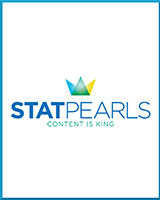
StatPearls [Internet].
Show detailsIntroduction
Baroreceptors and mechanoreceptors respond to changes in pressure or stretch in blood vessels within the aortic arch and carotid sinus. In part, they can respond to changes in pH and changes in specific metabolites in the blood. They help maintain mean arterial pressure, adjusting blood pressure based on physiological input and return to their baseline level of activity upon attaining homeostatic arterial pressure. They are a part of the afferent system, which senses pressure inputs and relaying these via cranial nerve signals to adjust blood pressure accordingly. Understanding the physiological principles of the baroreceptor mechanism is clinically significant in understanding the mechanism of carotid massage, carotid occlusion, and in the Cushing reflex.[1] In this review, we will explore the structure, function, and clinical relevance of the baroreceptors within the aortic arch and carotid sinus.
Structure and Function
Peripheral baroreceptors reside in the aortic arch and carotid sinus. The baroreceptors of the aortic arch transmit signals via the vagus nerve, or cranial nerve ten, to the solitary nucleus of the medulla. The location of the baroreceptors of the carotid sinus is where the common carotids bifurcate and transmit signals via the glossopharyngeal nerve, or cranial nerve nine, to the solitary nucleus of the medulla.[2]
The aortic arch and carotid sinus have stretch fibers which send electrical signals to the brain based on how much stretch stimulus they receive. As blood pressure increases, there is an increase in the stretch of the fibers of the aortic arch and carotid sinus, and this increases signals to the brain. The nucleus solitarius is the portion of the brain that receives neural messages and makes changes to blood pressure based on afferent inputs from the vagus nerve via the aortic arch and glossopharyngeal nerve via the carotid sinus.[3]
When increased blood pressure is detected, there is increased stretch fiber stimulation along the aortic arch and carotid sinus. The vagus and glossopharyngeal nerves send afferent inputs to the solitary nucleus of the medulla; this then leads to the efferent signal that exits the brain to cause veins to dilate, serving as a storage basin for blood and fluid in the heart, and for arteries to dilate to decrease blood pressure. This response is because decreased efferent sympathetic outflow causes decreased vasoconstriction which leads to reduced total peripheral resistance. Efferent signals also cause the heart to lower heart rate and to decrease contractility, because there is an increase in parasympathetic efferent outflow to the sinoatrial node which will reduce the heart rate. There is also a decrease in efferent sympathetic outflow, which causes a reduction in contractility of the heart and decreased heart rate, leading to reduced cardiac output. The increase in blood pressure also causes the kidney to decrease salt or water retention, leading to a decrease in blood pressure. These efferent signals from the heart, veins, arteries, and kidneys are compensatory for the increased blood pressure detected. Baroreceptor activity returns to baseline level upon attaining homeostatic arterial pressure.[4]
Hemorrhage, on the other hand, causes a decrease in blood pressure. This decreased blood pressure leads to fewer signals to be sent to stretch fibers in the aortic arch via the vagus nerve and carotid sinus via the glossopharyngeal nerve. Thus there are decreased inputs sent to the solitary nucleus of the medulla, which then leads to the efferent signal that exits the brain to cause veins and arteries to constrict, such that more fluid gets pushed into the heart and arteries. Efferent signals also cause the heart to increase heart rate and to increase contractility. These responses are because there is a decrease in parasympathetic efferent outflow which leads to an increase in heart rate to compensate. There is also an increase in efferent sympathetic outflow, which causes an increase in cardiac contractility, heart rate, vasoconstriction, and arterial pressure. This decrease in blood pressure also causes the kidney to increase salt or water retention, leading to an increase in blood pressure. These efferent signals from the heart, veins, arteries, and kidneys are compensatory for the decreased blood pressure detected.[5]
Embryology
During development, the baroreceptors are active. The motivation is to improve synaptic plasticity, thanks to several neurotrophic relationships with BDNF - brain-derived neurotrophic factor.
Their derivation is from the ectodermal sheet.
Clinical Significance
Baroreceptors in the aortic arch and carotid sinus have significant clinical significance. For example, carotid massage can occur when there is increased pressure on the carotid artery. Increased pressure on the carotid artery leads to increased signaling of stretch fibers, which causes increased electrical signaling of the baroreceptors from the increased stretch. The carotid sinus detects this increased firing of afferent signals via the glossopharyngeal nerve, leading to a misinterpretation of the existence of hypertension. To compensate for this, efferent signals to the nucleus solitarius will cause venous dilation, arterial dilation, decreased heart rate with increased atrioventricular node refractory period, and ultimately reduced blood pressure. This sudden decrease in blood pressure can cause syncope, which often presents in patients who have a history of syncope while shaving or buttoning their shirts, activities which cause increased pressure on the carotid artery.[6]
In contrast, during carotid occlusion, there is no blood flow to the carotid sinus. Decreased blood flow to the carotid artery leads to decreased signaling of the stretch fibers, which causes lower levels of electrical signaling of the baroreceptors from the reduced stretch. The carotid sinus detects this decreased firing of afferent signals via the glossopharyngeal nerve, incorrectly appearing to be hypotension. To compensate for this, efferent signals to the nucleus solitarius will cause venous constriction, arterial constriction, increased heart rate, and ultimately increased blood pressure. Carotid occlusive disease, or carotid stenosis, can present in patients with ischemic stroke.[7]
The Cushing reaction is another clinically significant application of the baroreceptor mechanism. This reaction is a triad of bradycardia, hypertension, and respiratory depression. In the Cushing reflex, increased intracranial pressure causes arterioles to constrict, which leads to cerebral ischemia, which leads to an increased pCO2 and decreased pH, leading to increased sympathetic in perfusion pressure, or hypertension. Hypertension causes and increases sympathetic afferent signal because of increased total peripheral resistance and increased arterial pressure. This hypertension leads to increased stretch signals, which leads to increased electrical signals of baroreceptor firing. This increased peripheral baroreceptor activity then leads to compensatory reflex bradycardia.[8]
Other Issues
The perception of pain diminishes with the retention of breath following a deep inhalation; this event reflects the intervention of the baroreceptors. The activation of the baroreceptors during systole attenuates the nociceptive stimulus.[9]
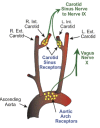
Figure
The relationship between the baroreceptors and the vagal system. Contributed by Bruno Bordoni, PhD.
References
- 1.
- Lau EO, Lo CY, Yao Y, Mak AF, Jiang L, Huang Y, Yao X. Aortic Baroreceptors Display Higher Mechanosensitivity than Carotid Baroreceptors. Front Physiol. 2016;7:384. [PMC free article: PMC5006318] [PubMed: 27630578]
- 2.
- Saku K, Kishi T, Sakamoto K, Hosokawa K, Sakamoto T, Murayama Y, Kakino T, Ikeda M, Ide T, Sunagawa K. Afferent vagal nerve stimulation resets baroreflex neural arc and inhibits sympathetic nerve activity. Physiol Rep. 2014 Sep 01;2(9) [PMC free article: PMC4270242] [PubMed: 25194023]
- 3.
- Fahim M. Cardiovascular sensory receptors and their regulatory mechanisms. Indian J Physiol Pharmacol. 2003 Apr;47(2):124-46. [PubMed: 15255616]
- 4.
- Lantelme P, Harbaoui B, Courand PY. [Resistant hypertension and carotid baroreceptors stimulation]. Presse Med. 2015 Jul-Aug;44(7-8):730-6. [PubMed: 26144275]
- 5.
- Geerdes BP, Frederick KL, Brunner MJ. Carotid baroreflex control during hemorrhage in conscious and anesthetized dogs. Am J Physiol. 1993 Jul;265(1 Pt 2):R195-202. [PubMed: 8342687]
- 6.
- Mateos JCP. Carotid Sinus Massage in Syncope Evaluation: A Nonspecific and Dubious Diagnostic Method. Arq Bras Cardiol. 2018 Jul;111(1):92-93. [PMC free article: PMC6078375] [PubMed: 30110050]
- 7.
- Förster A, Wenz H, Böhme J, Groden C, Alonso A. Asymmetrical Gadolinium Leakage in Ocular Structures in Stroke Due to Internal Carotid Artery Stenosis or Occlusion. Clin Neuroradiol. 2020 Jun;30(2):221-228. [PubMed: 30593604]
- 8.
- Saleem S, Teal PD, Howe CA, Tymko MM, Ainslie PN, Tzeng YC. Is the Cushing mechanism a dynamic blood pressure-stabilizing system? Insights from Granger causality analysis of spontaneous blood pressure and cerebral blood flow. Am J Physiol Regul Integr Comp Physiol. 2018 Sep 01;315(3):R484-R495. [PubMed: 29668325]
- 9.
- Bordoni B, Marelli F, Bordoni G. A review of analgesic and emotive breathing: a multidisciplinary approach. J Multidiscip Healthc. 2016;9:97-102. [PMC free article: PMC4778783] [PubMed: 27013884]
Disclosure: Yasaman Pirahanchi declares no relevant financial relationships with ineligible companies.
Disclosure: Bruno Bordoni declares no relevant financial relationships with ineligible companies.
- Anatomy, Head and Neck: Carotid Sinus.[StatPearls. 2024]Anatomy, Head and Neck: Carotid Sinus.Andani R, Khan YS. StatPearls. 2024 Jan
- Selective contribution of two types of carotid sinus baroreceptors to the control of blood pressure.[Circ Res. 1993]Selective contribution of two types of carotid sinus baroreceptors to the control of blood pressure.Seagard JL, Hopp FA, Drummond HA, Van Wynsberghe DM. Circ Res. 1993 May; 72(5):1011-22.
- Importance of aortic baroreflex in regulation of sympathetic responses during hypotension. Evidence from direct sympathetic nerve recordings in humans.[Circulation. 1989]Importance of aortic baroreflex in regulation of sympathetic responses during hypotension. Evidence from direct sympathetic nerve recordings in humans.Sanders JS, Mark AL, Ferguson DW. Circulation. 1989 Jan; 79(1):83-92.
- Review Baroreceptors in the Aortic Arch and Their Potential Role in Aortic Dissection and Aneurysms.[J Clin Med. 2022]Review Baroreceptors in the Aortic Arch and Their Potential Role in Aortic Dissection and Aneurysms.Reutersberg B, Pelisek J, Ouda A, de Rougemont O, Rössler F, Zimmermann A. J Clin Med. 2022 Feb 22; 11(5). Epub 2022 Feb 22.
- Review Paracrine role of prostanoids in activation of arterial baroreceptors: an overview.[Clin Exp Hypertens A. 1991]Review Paracrine role of prostanoids in activation of arterial baroreceptors: an overview.Chapleau MW, Hajduczok G, Abboud FM. Clin Exp Hypertens A. 1991; 13(5):817-24.
- Anatomy, Head and Neck: Carotid Baroreceptors - StatPearlsAnatomy, Head and Neck: Carotid Baroreceptors - StatPearls
Your browsing activity is empty.
Activity recording is turned off.
See more...