NCBI Bookshelf. A service of the National Library of Medicine, National Institutes of Health.
Wang Y, Zhao S. Vascular Biology of the Placenta. San Rafael (CA): Morgan & Claypool Life Sciences; 2010.
Placenta villi are composed of three layers of components with different cell types in each: (1) syncytiotrophoblasts/cytotrophoblasts that cover the entire surface of the villous tree and bathe in maternal blood within the intervillous space; (2) mesenchymal cells, mesenchymal derived macrophages (Hofbauer cells), and fibroblasts that are located within villous core stroma between trophoblasts and fetal vessels. Hofbauer cells synthesize VEGF and other proangiogenic factors that initiate vasculogenesis in the placenta; and (3) fetal vascular cells that include vascular smooth muscle cells, perivascular cells (pericytes), and endothelial cells.
4.1. Cytotrophoblasts and syncytiotrophoblasts
Trophoblasts (from Greek to feed: threphein) are cells forming the outer layer of a blastocyst, which provides nutrients to the embryo, and develops into a large part of the placenta. They are formed during the first stage of pregnancy and are the first cells to differentiate from the fertilized egg. Villous trophoblasts have two cell populations: undifferentiated cytotrophoblasts and fully differentiated syncytiotrophoblasts. The syncytiotrophoblasts are a continuous, specialized layer of epithelial cells. They cover the entire surface of villous trees and are in direct contact with maternal blood. The surface area of syncytiotrophoblasts is about 5 square meters at 28 weeks’ gestation and reaches up to 11–12 square meters at term [1]. Figure 4.1 illustrates total placental surface areas at different gestational ages. Fully developed terminal villi are the functional unit of maternal-fetal oxygen exchange and nutrient transport. Figure 4.2 shows hematoxylin and eosin (H & E) staining of a cross section of terminal villi of a term placenta and an electron microscopic section of a terminal villous. Note the relation of syncytiotrophoblasts and fetal capillaries. The fetal capillary basement membrane is very close to the maternal blood in the intervillous space.
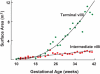
Figure 4.1
Placental surface areas at different gestational ages. () areas of intermediate villi; (
) areas of terminal villi. (Adapted from The Physiology of the Human Placenta, by Page K, Figure 2.7, published by UCL press). Reproduced from The Physiology of the (more...)

Figure 4.2
Hematoxylin and eosin (H & E) staining of a terminal villi tissue section and an electron microscopic section of a terminal villus. Fetal red blood cells are seen in villous core fetal vessels. Please note the intimate relationship of syncytiotrophoblasts (more...)
In terms of fetal-maternal communication, it is mainly the syncytialized trophoblasts that orchestrate the complex biomolecular interactions between the fetus and mother. Not only do placental trophoblasts provide structural and biochemical barriers between the maternal and fetal compartments during pregnancy, they also serve as an important endocrine organ that produces numerous growth factors and hormones that support and regulate placental and fetal development and growth [17–20]. Table 4.1 is a list of major hormones produced by placental syncytiotrophoblasts. The function of trophoblasts is tightly regulated by locally produced growth factors, components of the extracellular matrix (ECM), and binding between growth factors and proteoglycans. Furthermore, a successful pregnancy also largely depends on the angiogenic function of the trophoblast itself, i.e., its ability to invade maternal myometrial spiral arteries (during the first trimester) and the capacity to generate growth factors that vascularize the placenta during its mid- to later development (second and early third trimester). In terms of vasculogenesis and angiogenesis, it is believed that vascular endothelial growth factor (VEGF), placental growth factor (PlGF), fibroblast growth factors (FGFs), and their receptor families are key factors regulating trophoblast survival and angiogenesis in the placenta. It is well known that placental trophoblasts are the source of VEGF and PlGF. (See Chapter 7 for more details.)
Table 4.1
Function of major hormones produced by placental syncytiotrophoblasts.
Beneath the syncytiotrophoblasts are the cytotrophoblasts (Figure 3.2). These cells are considered to be stem cells for syncytiotrophoblasts. Cytotrophoblasts continually differentiate into syncytiotrophoblasts during villous formation and development. Cytotrophoblast invasion into the uterine spiral arteries is accompanied by loss of the endothelial lining and musculoelastic tissue in these vessels. This process of invasion is necessary for placental vascular remodeling in the early stages of the implantation process. As a result of this invasion process, there is a loss of elasticity and an increase in the luminal diameter of the spiral arteries. Consequently, the spiral arteries become low resistance vascular channels (Figure 2.3). Normal decidual vascular architecture formation is required to meet the gestational increases in the demand for blood flow to the placenta.
Preeclampsia is associated with shallow cytotrophoblast invasion: Preeclampsia is a hypertensive and multiple system disorder unique to human pregnancy. Preeclampsia is diagnosed with newly developed maternal hypertension and positive proteinuria after 20 weeks of gestation. Although the etiology of preeclampsia is unknown, evidence supports the notion that preeclampsia is associated with shallow cytotrophoblast invasion. Brosen et al. first described the abnormal “shallow” cytotrophoblast invasion in placentas from women whose pregnancies are complicated by preeclampsia [21]. They found that cytotrophoblast invasion of the uterus is only superficial, and the endovascular invasion does not proceed beyond the terminal portions of the spiral arterioles. The process of trophoblast invasion is normally completed by 20 to 22 weeks of gestation in normal pregnancy. However, in cases of preeclampsia it has been found that cytotrophoblast invasion of the uterine spiral arterioles is often incomplete by this time and spiral arteries fail to lose their muscular elastic components [21–23] Therefore, a critical underlying lesion in preeclampsia is the failure of extravillous trophoblasts to invade the muscular spiral arteries into their myometrial portion and convert them to “low-resistance” capacitance vessels. Consequently, placental hypoxia and reduced placental perfusion characterized with “low flow and high resistance” are the central hallmarks of preeclampsia. Figure 4.3 illustrates abnormal spiral artery remodeling that results in low flow and high resistance in cases of preeclamptic placenta [24].
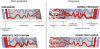
Figure 4.3
Illustration of uterine and placental vasculature in the non-pregnant, pregnant and immediate post-partum state. Normal pregnancy is characterized by the formation of large arterio-venous shunts that persist in the immediate post-partum period. By contrast (more...)
4.2. Villous core stroma cells
Demir et al. analyzed the ultrastructure of human placental villous tissues from 21 days p.c. through 40 weeks of gestation [25]. They found that early villous core fetal cells at 21 days p.c. appear to originate from macrophage-like mesenchymal precursor cells—mesenchymal stem cells. These cells are differentiated by vasculogenesis and angiogenesis. In the early stage of placental development, mesenchymal stem cells transform into hemangioblastic cell cords, which are believed to be the precursors of capillary endothelial cells and hematopoietic stem cells [25]. Mesenchymal stem cells can also differentiate into perivascular cells, which are considered predecessors of capillary endothelial cells. In the late stage of gestation, fetal villous core angiogenesis results from the proliferation of existing endothelial cells and pericytes rather than via the expansion of hemangioblastic cells [25].
Hofbauer cells were first described by Hofbauer in 1903 [26]. Hofbauer cells are the macrophages in the placenta villous stroma. These cells are of mesenchymal origin and expand during the first and second trimesters in placental villous tissues. Hofbauer cells are antigen-presenting cells in the placenta, which play a critical role in maintaining host defense. These placental macrophages also contribute to trophoblast differentiation and angiogenesis by producing various growth factors and cytokines. Although the exact mechanism underlying placental vascular development is not clear, it is thought that Hofbauer cells must govern mesenchymal villi and villous sprouting during the vasculogenic and angiogenic development of fetal vessels in the human placenta. Hofbauer cells express and produce many angiogenic factors including VEGF, FGF, vasculotropin, and vascular endothelial cell proliferation factor [27–30]. VEGF is a highly specific endothelial cell mitogen that induces endothelial proliferation, cell migration, and inhibits apoptosis. In vivo VEGF induces angiogenesis and plays a central role in the regulation of vasculogenesis. Demir et al. found that Hofbauer cells are often found close to cytotrophoblasts, vasculogenic and angiogenic precursor cells in the villous tissue [30]. Similar reports were published by Seval et al. [31] who examined CD31/PECAM-1 and CD68 immunoreactivities by staining tissues from first trimester placentas. They found that the majority of Hofbauer cells in the placental villous core are in close vicinity to angiogenic cell cords and primitive vascular tubes, with the number of Hofbauer cells being significantly correlated with vasculogenic structures [31]. The developmental patterns of expression and secretion of VEGF by Hofbauer cells suggests that they are involved in recruiting, maintaining, and forming angiogenic cells during the course of villous core development. These findings further support the idea that molecular interactions between Hofbauer-cell derived VEGF with its receptors VEGFR-1 (Flt-1) or VEGFR-2 (Flk-1/KDR) on vascular precursor target cells regulate vasculogenesis and angiogenesis in the placenta [30].
Hofbauer cells also express sprouty (Spry) proteins and Spry interaction protein c-Cbl [32]. Among the four isoforms of Spry proteins, Spry-2 has been found to be involved in the FGF signaling, modulating different types of tissue branching during placental development. Spry-2 expression was abundant in the Hofbauer cells first trimester placental tissues, localizing near the cytotrophoblasts and syncytiotrophoblasts [32]. Spry proteins are important regulators of branching morphogenesis and growth-factor signaling. Although Spry proteins have been shown to direct tubular morphogenesis associated with tracheal/lung development, uteretic budding, and endothelial angiogenesis [33], however, a reduction of Spry2 expression by siRNA in villous explant culture paradoxically shows a marked increase in villous outgrowth [34]. Although their roles in trophoblast sprouting have not yet been defined, Hofbauer cells clearly modulate trophoblast migration and branching during chorionic villous tree development [32].
4.3. Pericytes/endothelial cells
Pericytes are perivascular cells with dendritic processes that surround capillary endothelium and venules. The ability of pericytes to support endothelial cells is fundamental for maintaining vessel stability and microvascular integrity [35] by restricting growth-factor-induced endothelial cell migration and proliferation [36,37]. Pericytes have also been shown to influence blood vessel functions including microvascular contractility, solute permeability, and smooth muscle stem cell function.
In the placenta, pericyte function is poorly understood. However, it is believed that pericytes are derived from mesenchymal stem cells and that they participate in different phases of placental vasculogenesis. Challier et al. made several observations [37]. When pericytes are co-cultured with endothelial cells, pericytes rapidly become the dominant cell type, indicating higher proliferative potential of pericytes than endothelial cells. Both pericytes and endothelial cells are involved in the formation of vascular “nodules” seen in prolonged in vitro culture. Depending on their functional state, degree of differentiation, and spatial relationship with endothelial cells and trophoblasts, pericytes play diverse roles during placental vascular development and angiogenesis. Although signaling mechanisms between pericytes and endothelial cells and between pericytes and trophoblasts are largely unknown, it is believed that pericytes play critical roles in maintaining microvessel stability and integrity, especially in the terminal villi, which are characterized by their high degree of capillarization and the presence of highly dilated sinusoids. Their intimate relationship with endothelial cells makes them likely candidates for sensing and regulating blood flow in the terminal villi of the placenta.
Placental microvascular endothelial cells exhibit remarkable changes of their angiogenic status throughout pregnancy, i.e., dominant angiogenic activity is associated with rapid placental expansion during the first and second trimesters, followed by a dominant angiostatic condition that is associated with placental growth arrest when pregnancy approaching to term. Thus, adequate placental microvascular endothelial function is key for a successful pregnancy. The inherent angiogenic regulation of these cells, their complex interactions with perivascular cells, and the appropriate vascularization responses require endothelial cells to rapidly remodel and adapt, paralleling the special needs of pregnancy, all of which are genetically programmed. Most studies that attempt to elucidate the functional role of placental microvascular endothelial cells have been restricted to microscopic analysis of frozen tissue sections [38]. Although it has been reported that placental microvascular endothelial cells express VEGF and its receptors, integrins, thrombomodulin, adhesion molecules, and HLA-B/C [38], an understanding of the molecular and functional regulation of these cells during placental development remains limited.
Lang et al. isolated endothelial cells from fetal artery and vein of human placenta, termed HPAECs and HPVECs, respectively [39]. Contrasting differences were found between HPAECs and HPVECs in terms of phenotype, genotype, and function. HPAECs are polygonal in shape with a smooth surface growing in loose arrangements and forming monolayers with classical endothelial “cobblestone” morphology. HPAEC express more artery-related genes such as hey-2 (hairy/enhancer-of-split related with YRPW motif, 2), connexin 40, and depp (decidual protein induced by progesterone) and more endothelial-associated genes than HPVECs. In comparison, HPVECs are spindle-shaped cells with numerous microvilli at their surface. These cells grow closely against each other, forming fibroblastoid “swirling” morphology at confluence with shorter generation and population doubling times than HPAECs [39]. Compared to HPAECs, HPVECs express more development-associated genes including gremlin, mesenchymal homeobox 2, and stem cell protein DSC54. These cells also show an enhanced potential to differentiate into adipocytes and osteoblasts compared to HPAECs [39]. In addition, HPAECs have a higher proliferative response to VEGF stimulation, whereas HPVECs are more responsive to PlGF [39]. Table 4.2 shows a list of endothelial-associated genes and their respective function in HPAECs and HPAECs [39].
Table 4.2
Endothelial-associated genes in human placental artery and vein endothelial cells.
- Cell Types of the Placenta - Vascular Biology of the PlacentaCell Types of the Placenta - Vascular Biology of the Placenta
Your browsing activity is empty.
Activity recording is turned off.
See more...