NCBI Bookshelf. A service of the National Library of Medicine, National Institutes of Health.
National Academies of Sciences, Engineering, and Medicine; Health and Medicine Division; Board on Population Health and Public Health Practice; Committee on the Review of the Health Effects of Electronic Nicotine Delivery Systems; Eaton DL, Kwan LY, Stratton K, editors. Public Health Consequences of E-Cigarettes. Washington (DC): National Academies Press (US); 2018 Jan 23.
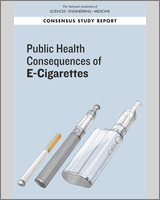
Public Health Consequences of E-Cigarettes.
Show detailsIn general, e-cigarettes often contain ingredients such as propylene glycol (PG) and glycerol, mixed with concentrated flavors and, optionally, a variable percentage of nicotine. Quantitative and qualitative studies have identified a wide variety of chemical components in the cartridges, refill solutions, and aerosols of e-cigarettes. Herrington and Myers (2015) have detected approximately 60 to 70 compounds (unidentified and identified) in each liquid tested, only varying by several constituents throughout the liquid. Kucharska and colleagues (2016) have identified 113 chemicals in 50 brands of liquids. Even more compounds are observed in the aerosol over their respective solution because some chemicals are generated during the vaporization process. An aerosol generated from a single product tested by Herrington and Myers (2015) showed 18 additional compounds observed in the solution.
Substances identified in e-cigarette liquids and aerosols include nicotine, solvent carriers (PG and glycerol), tobacco-specific nitrosamines (TSNAs), aldehydes, metals, volatile organic compounds (VOCs), phenolic compounds, polycyclic aromatic hydrocarbons (PAHs), flavorings, tobacco alkaloids, and drugs. Most reviewed studies have evaluated nicotine and impurities in the liquids such as TSNAs and nicotine-related impurities, while other studies have focused on identifying potentially harmful chemicals in the aerosol, such as carbonyl compounds, VOCs, TSNAs, metals, and silicates. Various chemical substances and ultrafine particles known to be toxic, carcinogenic, and/or to cause respiratory and cardiac disease have been identified in e-cigarette aerosols, cartridges, refill liquids, and environmental emissions. Some of the identified TSNAs, aldehydes, metals, VOCs, phenolic compounds, PAHs, and tobacco alkaloids are harmful or potentially harmful constituents, and their general health risks are described below.
HUMECTANTS (DELIVERY SOLVENTS)
E-cigarettes use humectants as solvent carriers in e-liquids to produce aerosols that simulate combustible tobacco cigarette smoke. In addition to these humectants, water is a common ingredient of e-liquids. PG and glycerol (commonly referred to as a “vegetable glycerin” in liquid formulations) are the most common vaporizing solvents used in e-cigarettes. Hutzler and colleagues (2014) analyzed 28 liquids of 7 manufacturers purchased in Germany and detected both PG and glycerol in all samples. Both PG and glycerol are also commonly used as humectant ingredients in manufactured cigarettes to control and maintain the moisture content of the cut tobacco filler (Uryupin et al., 2013). Users of e-cigarettes often report that PG produces better “throat hit” and carries flavor better than glycerol while glycerol is much smoother than PG. PG is physically much thinner than glycerol (Cheng, 2014; Etter, 2016; Li et al., 2016). Outside of usage in e-cigarette liquids, dermal exposure to PG and glycerol is more common than exposure via inhalation, as most consumer products containing PG and glycerol are liquids or creams. Thus, there are few animal or human studies providing evidence of the possible toxicity of inhaled PG or glycerol. Studies identifying PG and glycerol in e-liquids are described below, and toxicological evidence is described in the following sections.
Hahn and colleagues (2014) used nuclear magnetic resonance methodology for analysis of 54 commercially available liquids for use in e-cigarettes. The study looked at several types of humectants, including dihydroxy (diols, glycols) and polyhydroxy alcohols. PG and glycerol were detected in all samples at concentrations ranging from 0.4 to 98 g/100 g (average 57 g/100 g) and from 0.3 to 95 g/100 g (average 37 g/100 g), respectively. Generally, lower levels of another solvent, ethylene glycol (average 10 g/100 g), were detected. 1,3-Propanediol was detected only in seven samples in the concentration range of 3.3–10 g/100 g. 1,3-Butanediol and diethylene glycol were negative in all samples. The presence of the major compounds glycerol and PG corresponded to the labeling in the majority of cases, except three products contained no labeling information at all. Glycerol was not labeled on five products despite being present. PG was not labeled in two products despite being present. In one case, “vegetal glycol” was labeled without specifying the exact chemical compound. Hutzler and colleagues (2014) analyzed 28 liquids purchased from 7 manufacturers in Germany and, like Hahn and colleagues, detected both PG and glycerol in all samples. Geiss and colleagues (2016) extrapolated lung concentration of PG and glycerol emitted from e-cigarettes using a smoking machine by measuring the average amounts condensed on the filter pad. The estimated lung concentrations were 160 and 220 mg/m3 for PG and glycerol, respectively.
The most common symptom reported by e-cigarette users is a dry mouth and throat, which is considered to originate from the water-absorbing property of PG and glycerol. However, the health consequences of long-term exposure to PG and glycerol from e-cigarettes have not been investigated. Both compounds might pyrolyze, leading to the formation of carbonyl compounds (aldehydes), which contribute to potential health risks in e-cigarette users (for discussion about carbonyl compounds, see the subsequent section in this chapter).
Propylene Glycol
PG (also known as 1,2-dihydroxypropane, 1,2-propanediol, methyl glycol, and trimethyl glycol) is a clear, colorless, slightly syrupy liquid at room temperature. It is practically odorless and tasteless. It is used by the chemical, food, and pharmaceutical industries as a humectant to absorb extra water and maintain moisture in certain medicines, cosmetics, or food products. It is also used as a solvent for food colors and flavors, and in the paint and plastics industries. PG has been widely used for decades as a solvent for many intravenous drugs, and in some oral preparations such as cough syrups. PG was listed as generally recognized as safe (GRAS) by the Food and Drug Administration (FDA) in 1973 (HHS, 2015). Substances listed as GRAS are deemed as generally safe under conditions of intended use as a food additive. Thus, GRAS substances are safe for ingestion, but not necessarily for other routes of administration like inhalation. PG may exist in air in the aerosol form, but must be heated or briskly shaken to produce a mist. PG is also used to create artificial smoke or fog used in firefighter training and in theatrical productions.
Human Studies and Case Reports on PG Toxicity
Some people have reported having an allergic reaction to PG. Some people have reported upper respiratory irritation after inhaling aerosolized PG for 1 minute (Wieslander et al., 2001), but the longer term health effects in humans are not well defined. Though some preclinical studies showed inhalation of PG and glycerol can be safe up to 28 days (Werley et al., 2011) or 18 months (Robertson et al., 1947), breathing aerosolized PG can also affect the risk of asthma development (Choi et al., 2010). For example, one woman exhibited signs of exogenous lipoid pneumonia (e.g., fever, productive cough, and labored breathing) after using e-cigarettes for half a year (McCauley et al., 2012). The e-cigarette's oil-based humectants likely caused her pneumonia, as her symptoms improved when she quit the device (McCauley et al., 2012).
PG is frequently used as a vehicle for intravenous delivery of anti-seizure medications in pediatric populations, typically at concentrations of 40 to 80 percent v:v with saline (Lim et al., 2014). Thus, there have been numerous human studies on the toxicity of relatively large doses of both oral and intravenously administered PG. Lim and colleagues (2014) conducted a systematic literature review of case reports and other clinical studies on the toxicity of PG in pediatric populations. They identified numerous case reports and several small studies that identified a “toxidrome” for PG toxicity that can result following repeated, relatively high-dose intravenous administration of PG. The adverse effects include hyperosmolarity, lactic acidosis, hemolysis, central nervous system (CNS) toxicity, and cardiac arrhythmia. In one particularly striking case study, an 11-year-old was given 2–4 ml per day of PG containing vitamin D for 13 months. Estimated daily dose for this subject was 114 mg (2-ml dose) to 228 mg (4-ml dose) of PG/kg body weight (Arulanantham and Genel, 1978; LaKind et al., 1999). After 13 months of repeated exposures, the child began to have seizures and lapsed into unconsciousness. Once the PG/vitamin D preparation was stopped, the child recovered (LaKind et al., 1999). In another example, a 15-month-old infant receiving large doses of a vitamin C suspension in PG orally had episodes of unresponsiveness, diaphoresis, tachycardia, tachypnea, and hypoglycemia (Martin and Finberg, 1970).
Based on analyses of case reports, Lim and colleagues (2014) attempted to arrive at a “safe” dose of PG for repeated administration of antiseizure drugs that are routinely compounded in 40 percent PG (see Table 5-1). They suggested maximum cumulative dose of 69 g/day in a pediatric population. Although such clinical studies on relatively high doses of orally and intravenously administered PG in pediatric populations is clinically relevant for those populations, it is perhaps of modest relevance to potential health consequence of inhalation of PG vapors from repeated vaping. However, diagnostic procedures, such as characterization of anion gap (or osmolal gap, defined as the discrepancy between the measured and calculated osmolalities) (Lim et al., 2014), and evaluation for the presence of lactic acidosis, could be of potential value in suspected cases of high-dose PG toxicity from extensive vaping.
TABLE 5-1
Dose Limits of Commonly Used Drugs to Avoid Propylene Glycol Intoxication Based on a Maximum Amount of PG Equal to 69 g/day.
Pharmacokinetics of PG
PG is well-absorbed orally and can also be absorbed through skin or mucous membranes from topical preparations. Following absorption, the kidneys eliminate 45 percent of the PG, and the liver metabolizes the remainder to lactic acid, pyruvic acid, or acetone. Thus, patients with impaired liver and/or kidney function are generally thought to be at increased risk for developing PG toxicity following high-dose oral or intravenous administration.
Speth and colleagues (1987) conducted a relatively detailed pharmacokinetic analysis of PG following intravenous administration of PG at different dose rates, administered over 4 hours. The elimination half-life of PG was dose dependent; at doses of either 3 or 4.5 g/m2 (over 4 hours) the terminal half-life was approximately 1.8 hour. However, at a dose rate of 7.5 g/m2 over 4 hours the half-life increased to approximately 3.1 hours, suggesting saturable elimination at dose rates above about 5 g/m2 (see Table 5-2).
TABLE 5-2
Plasma Pharmacokinetics of Propylene Glycol Given as a 4-Hour Intravenous Infusion.
Yu and colleagues (1985) also reported elimination half-lives of PG following multiple large oral doses (20.7 g three times per day, or 41.4 g two times per day) of PG, with terminal half-lives of 3.8 ± 0.8 hours, with relatively large interpatient variability in plasma concentration. Blood concentrations of PG associated with hyperosmolality and anion gap have been reported, ranging from 177 to 1,520 µg/ml (Fligner et al., 1985; Kelner and Bailey, 1985). However, Yu and colleagues (1985) did not observe any evidence of toxicity (hyperosmolality or lactic acidosis) in subjects with plasma concentrations as high as 425 µg/ml. No studies have evaluated blood concentrations of PG in subjects using e-cigarettes or other vaping devices with PG as the humectant.
Evidence of Health Effects from Occupational Exposures to PG
There is relatively limited evidence of toxicity from occupational exposures to PG. However, glycols are used in theatrical fogs, so actors and performers in the entertainment industry may have routine exposures to relatively high concentrations of PG, as it is often a major component of these fogs. Varughese and colleagues (2005) studied 101 employees in 19 different locations who were routinely exposed to such fogs. They measured the levels of exposure, lung function, and acute and chronic symptoms. The mean concentration of exposure for employees exposed only to PG-based fog on the testing day was 0.49 mg/m3 (maximum 3.22 mg/m3). They reported that theatrical fog exposures were significantly associated with chronic work-related wheezing and chest tightness. Although these acute effects appeared to be specific to PG-based fogs, most of the workers were also exposed to mineral oil. Thus, the authors were unable to distinguish the role of PG or mineral oil fogs in the development of chronic effects and work-related symptoms from increasing chronic exposure.
Another study addressed the same general issue regarding the safety of PG used in theatrical fog (Moline et al., 2000). Based on their analysis of symptoms reported by 218 theatrical actors, detailed integrated PG dose and peak exposure estimates were available. They found statistically significant associations between peak PG exposure and reported symptoms of mucous membrane irritation. They also found other respiratory symptoms, including throat and nasal symptoms associated with peak exposure but not integrated dose. The measured peak concentrations during “fogging” at on-stage locations ranged from less than 1 to 16 mg/m3. Estimates of actors' “per performance” exposures to PG ranged from 0.1 to ~8 µg/show (Moline et al., 2000).
Wieslander and colleagues (2001) conducted a study to examine the effects of PG mist in aviation emergency training. Twenty-seven non-asthmatic volunteers were exposed in an aircraft simulator to a mist of PG at 309 mg/m3 (176–851 mg/m3 range) for 1 minute. Subjects were then evaluated for a range of pulmonary function tests and symptoms assessment. Although measures of pulmonary function (FEV1, vital capacity) were not significantly affected, symptoms reported included eye and throat irritation in some of the subjects. Four subjects also reported development of an irritating cough. The reported symptom of eye irritation was supported by measurement of tear film stability, which was decreased following PG exposure.
The Occupational Safety and Health Administration (OSHA, 2006) established an interim 8-hour threshold limit value (TLV) of 10 mg/m3 for all organic mists (applicable to PG and glycerol) with no specific exposure limits or identified toxicity. The Health Council of the Netherlands (2007) recommends an exposure limit for PG of 50 mg/m3 over 8 hours. Although they noted a concern about short-term respiratory effects, the proposed limit was not based on observed adverse effects from workplace exposures. Thus, although occupational exposure limits have been proposed for PG, it is important to note that neither of these proposed exposure limits are based on evidence of adverse effects, but rather are “precautionary” in nature. Nevertheless, studies in some workplace populations relate symptoms of eye and throat irritation to acute, and possibly chronic, exposures to PG mist in the low milligram per cubic meter concentrations.
Relevance of Occupational Exposures and Clinical Case Reports of Pharmaceutical Exposures of PG to Exposures from E-Cigarettes
Although the clinical case reports of PG exposures demonstrate that high-dose oral and intravenous exposure to PG can induce toxicity, the relevance of those studies to potential health effects of PG from e-cigarettes depends on the dose and pharmacokinetics of PG following inhalation exposure through e-cigarettes. Burstyn (2014) estimated the potential levels of exposure to PG from e-cigarettes, “assuming extreme consumption of the liquid per day via vaping (5 to 25 ml/day and 50–95 percent propylene glycol in the liquid)” and concluded that “levels of propylene glycol in inhaled air can reach 1–6 mg/m3.” With an assumption of complete absorption via inhalation, Burstyn concluded that “estimated levels of exposure to propylene glycol and glycerin are close enough to TLV to warrant concern.” However, putting these values in perspective with the clinical data from intravenous administration of PG in adults may be useful. Speth and colleagues (1987) reported that doses from 5 to 21 g/day (see Table 5-2), which are comparable to the 5 to 25 ml/day calculated by Burstyn (2014), were not associated with any evidence of any adverse effects. In the Speth and colleagues (1987) study, peak plasma concentrations of PG ranged from 48 µg/ml (5.1 g/day; ~88 mg/kg/day) to 425 µg/ml (21 g/day; ~488 mg/kg/day). In one clinical report in a 60-year-old male showing toxicity and for whom blood concentrations were measured, serum levels of PG greater than 180 µg/ml were reported to be associated with toxicity (Arbour and Esparis, 2000). Other investigators found clinical evidence of toxicity at serum PG concentrations that exceeded 250 µg/ml (Hansen et al., 2015), although it is important to note that these are following intravenous administration. Nevertheless, absorption of PG via inhalation theoretically could be very rapid and largely complete, so the comparison of blood levels between patients administered PG intravenously over 4 hours and individuals with extensive vaping may not be unreasonable.
In 1974, the World Health Organization recommended a maximum dose of 25 mg/kg/day of PG when ingested as a food additive. Thus, for a typical young adult with a body weight of 60 kg, this would be equivalent to 1.5 g/day, which is considerably less than the 5–25 ml/day “worst case” exposure to PG from vaping estimated by Burstyn (2014).
There are no studies of clinical measures of potential PG toxicity (e.g., anion gap, lactic acidosis) among heavy users of e-cigarettes, or which have measured blood/serum levels of PG following use of vaping devices containing PG-based liquids.
Allergic Reactions to PG
It has been known for years that some individuals can develop allergic reactions to PG following repeated dermal applications (Aberer et al., 1993; Catanzaro and Smith, 1991; Funk and Maibach, 1994; Lessmann et al., 2005; Warshaw et al., 2009). Although most dermal reactions to PG are the result of irritation, true immunological reactions have been confirmed through patch testing. For example, in a patch test of 1,226 patients who received an application of 5 percent PG in Vaseline, or 10, 30, or 50 percent in water, 208 (17 percent) of the subjects had evidence of irritation and/or allergic dermatitis. Of those showing some dermal reaction, 195 were from irritation, but 13 exhibited an allergic reaction (Aberer et al., 1993). However, a more recent analysis of allergic dermatitis found an incidence of only 2.1 percent in a large sample (5,083 subjects in 2007–2008), and this was significantly decreased from previous years (3.8 percent of 4,095 subjects in 1996–1998) (Fransway et al., 2013). Whether PG could induce allergic reactions via inhalation from e-cigarettes has not been studied.
In Vivo Animal Toxicology Studies of PG
Because of its widespread use as a food additive and other industrial uses, PG was subjected to standard in vivo toxicological assays many years ago, and these studies, coupled with the relative lack of human evidence of toxicity of PG from its use as a food additive, form the basis for FDA's listing of PG as GRAS. A study of male and female Sprague-Dawley rats found that larynx, trachea, and lung tissues were not affected by nose-only exposure to different levels of PG for 90 days (Suber et al., 1989). Additional studies of aerosolized PG found no effects on rat or monkey gross pathology, respiratory tract function, histology, or hematology and clinical chemistry (Robertson et al., 1947). LaKind and colleagues (1999) provide a comprehensive review of the animal toxicology data for PG prior to that date.
Acute Toxicity
PG is considered “practically non-toxic” orally, with acute lethal dose (LD50) values of 20 g/kg or greater (see Table 5-3). Signs and symptoms of acute toxicity included increased respiratory rate, loss of equilibrium, CNS depression, analgesia, coma, and death in 18 to 36 hours. Of more relevance are animal studies using inhalation exposures to PG. Konradova and colleagues (1978) evaluated the effects on airway epithelia of exposure of rabbits to a 10 percent aerosol of PG for 20 and 120 minutes. The 20-minute exposure had no visible effect on ciliated cells in the tracheal epithelium, but did produce alterations in goblet cells. Longer exposure, for 120 minutes, altered goblet cells and induced some visible alterations in ciliated epithelial cells. Another study examined the results of 15-minute inhalation exposure of dogs to either 10 or 20 percent aerosol of PG on hemodynamic effects and hemolysis (effects seen following large oral doses of PG). No effects on either endpoint were reported (MacCannell, 1969; Renne et al., 1992).
TABLE 5-3
Acute Lethal Dose (LD50) of Propylene Glycol in Rats, Mice, Guinea Pigs, and Rabbits.
Repeated Dose Exposures to PG to Evaluate Potential Reproductive Effects in Animals
Three standard reproductive assays of PG have been performed, all in male and female mice, using repeated doses and multigeneration assessment for reproductive outcomes (Kavlock et al., 1987; Morrissey et al., 1989; OECD, 2001). None of these studies reported any statistically significant effects of PG exposure on measures of reproductive outcome in different strains of mice given 10,100 mg/kg/day for 14 weeks.
Repeated Dose Exposures to PG to Assess Developmental/Teratogenic Effects in Animals
Several animal studies using standard teratogenicity protocols have been completed for PG. An FDA-sponsored study in pregnant CD-1 mice, Wistar rats, golden hamsters, and Dutch-belted rabbits found no evidence of teratogenicity at the highest doses tested (1,600, 1,600, 1,550, and 1,250 mg/kg/day for 10 days, respectively) (FDRL, 1973).
Long-Term (Chronic Exposure) Bioassays on PG for Assessment of Organ System Function
Because of the well-documented nephrotoxic effects of ethylene glycol, early studies on the toxicity of PG focused on potential effects of chronic PG exposure on kidney functions. Van Winkle and Newman (1941) administered PG in drinking water to female (5 percent PG, twice daily) and male dogs (600 ml of 10 percent PG, once daily) for up to 9 months. Animals were evaluated for liver and kidney function and by histopathology at the end of the experiment. No effects on liver or kidney were observed in any of the animals. A 2-year chronic bioassay in albino rats given PG in the diet at doses approximately equivalent to 1,225 or 2,450 mg/kg/day found no evidence of any organ system toxicity (LaKind et al., 1999; Morris et al., 1942). A 2-year feeding study in dogs given up to 2,000 mg/kg/day also found no significant effects on renal weight. However, a dose of 5,000 mg/kg/day was associated with increased urinary output and decreased water consumption, suggestive of adverse effects on kidney function (LaKind et al., 1999). Other chronic studies of PG in mice and dogs reported in Patty's Industrial Hygiene and Toxicology (Clayton and Clayton, 1995) found no significant effects of PG on any organ system.
Inhalation Exposure Levels of PG from E-Cigarette Use
Of importance to the question of the potential health effects of PG in the context of e-cigarette use are the actual concentrations and doses inhaled during a puff. Kienhuis and colleagues (2015) evaluated exposures to both PG and glycerol from a “shisha-pen” device. The authors define a shisha-pen as “an electronic cigarette (e-cigarette) variant that is advertised to mimic the taste of a water pipe, or shisha. . . . The shisha-pen operates in the same manner as an e-cigarette, it can be disposable or rechargeable and refillable, and it is available with and without nicotine” (Kienhuis et al., 2015). They estimated that the PG exposure from one 50-to 70-mL puff would be from 430 to 603 mg/m3, and noted that “These exposure concentrations were higher than the points of departure for airway irritation based on a human study (propylene glycol, mean concentration of 309 mg/m3) and a rat study (glycerol, no-observed adverse effect level of 165 mg/m3)” (Kienhuis et al., 2015, p. 1). As discussed above, Wieslander and colleagues (2001) exposed healthy human subjects in an aircraft simulator to a mist of PG at 309 mg/m3 (176–851 mg/m3 range) for 1 minute. This is similar to the range of PG concentrations in puffs of e-liquid from a shisha-pen device. No effects on lung function were noted by Wieslander and colleagues, although some subjects did complain of eye and throat irritation. This is consistent with Web-based literature from vaping groups.1
Summary of Toxicological Effects of PG
PG has long been considered “practically non-toxic,” consistent with FDA's inclusion of PG on the GRAS list. Animal studies, including chronic studies at very high levels, have consistently failed to identify any target organ, or other evidence of toxicity at doses less than several grams per kilogram per day. Although most of these studies were done decades ago and would not generally meet today's “good laboratory practices” standards, the large doses used, coupled with the consistent lack of any evidence of organ system effect or reproductive or developmental toxicity, provides strong support for the general lack of toxic effects of PG in humans from dietary or occupational exposures. However, there is limited, but consistent evidence from case reports that very high doses of PG administered orally or intravenously to humans can produce toxic effects that appear to be related to osmolar changes in the blood and lactic acid formation secondary to the metabolism of PG.
Finding: Substantial toxicological data indicate that oral exposure to propylene glycol is not likely to be associated with adverse health effects. However, the data from inhalation exposure to propylene glycol are limited. In some individuals, exposure to propylene glycol aerosols in concentrations found in e-cigarettes has been shown to cause irritation to the eyes and throat.
Glycerol
Glycerol (also known as glycerin) is an oily, hygroscopic liquid with a warm, sweet taste. Although glycerol can be derived from naturally occurring fats and oils (“vegetable glycerin”), synthetic glycerol is produced from petrochemical products in a multistep process. Glycerol is used in food products, nutritional supplements, pharmaceutical products, personal care products, and oral care products.
As discussed above, most liquids used in e-cigarettes and other vaping devices contain a mixture of PG and glycerol. Typically, the mixtures are somewhere in the range of 30–50 percent glycerol, with the balance as PG. Among the vaping community, there is a perception, which is supported by acute toxicology studies, that PG is more irritating to upper respiratory airways than glycerol.2 FDA considers glycerol GRAS.3
Human Toxicology Studies
The toxicology of glycerol was reviewed by the Organisation for Economic Co-operation and Development (OECD, 2002). A study of 10 male and 4 female volunteers who were administered glycerol in orange juice with each meal at a dose of 1.3 to 2.2 g/kg/day for 50 days reported no evidence of toxicity or adverse effects on blood or urine production. Based on the highest administered dose, they estimated a no observed adverse effect level (NOAEL) for glycerol greater than or equal to 2.2 g/kg/day (CIR, 2014; OECD, 2002; Tourtellotte et al., 1972).
When used as a drug, reported adverse effects following the oral administration of glycerol at unspecified doses included mild headache, dizziness, nausea, vomiting, thirst, and diarrhea. Headache is likely a result from dehydration (CIR, 2014). Venable and colleagues (CIR, 2015; Venable et al., 1980) evaluated 64 male employees involved in the manufacture of synthetic glycerol for potential effects on reproductive function. They found no differences in sperm counts and percentage of normal forms compared with a similar size control group (n = 63) that had no known occupational exposures to glycerol.
Absorption, distribution, metabolism, and excretion Glycerol is a natural product and endogenous component in the body, largely as triglycerides with fatty acids, but free glycerol is also naturally present in human plasma. Typical serum levels of glycerol in adult humans range from 0.05 to 0.1 mmol/L (Nelson et al., 2011). Exogenous glycerol is rapidly absorbed from the stomach and intestine, with distribution occurring throughout the extracellular space (CIR, 2015). The primary pathway of biotransformation is via glycerol kinase-mediated phosphorylation to α-glycerophosphate in the liver (80 to 90 percent) and kidneys (10 to 20 percent). α-Glycerophosphate is then transformed to form glucose (gluconeogenesis) and glycogen through intermediary metabolic pathways (Lin, 1977). Most of the dose of orally administered glycerol is metabolized in about 2.5 hours, with 7 to 14 percent of eliminated glycerol unchanged in urine. In the liver, exogenously administered glycerol can undergo lipogenesis (combining with free fatty acids to form triglycerides), and these fats can be distributed to adipose tissues. The turnover rate for glycerol is proportional to plasma concentration of glycerol (Bortz et al., 1972).
Glycerol has been used clinically because of its ability to increase the osmotic pressure in plasma. Orally administered glycerol can reduce the volume of intraocular fluids in order to decrease intraocular pressure (IOP). The extent of IOP reduction depends on both the etiology and magnitude of the increased pressure and the glycerol dose. Glycerol's osmotic effect has also been used to decrease in cerebrospinal fluid pressure (Tourtellotte et al., 1972).
In Vivo Animal Toxicological Studies
Acute toxicity As summarized in the Cosmetic Ingredients Review for glycerol (CIR, 2015), oral LD50 values of glycerol ranged from 2.53 to 58.4 g/kg in rats. The highest dose used in one study was 24 g/kg, and no deaths were reported. Oral LD50 values reported for glycerol were 4.1 to greater than 38 g/kg in mice, 27 g/kg in rabbits, and 77.5 g/kg in guinea pigs (CIR, 2015). The dermal LD50 value of glycerol in rats was reported to be greater than 21.9 g/kg, and in rabbits, greater than 18.7 g/kg. The approximate value for the time to death for 50 percent of the rats (LT50) was 423 minutes for exposure to glycerol aerosols at 11.0 mg/L (CIR, 2015). Reported intraperitoneal LD50 values of glycerol were 4.42–10.1 g/kg in rats and 8.6–9.5 g/kg in mice. LD50 values of glycerol via subcutaneous administration were 100 mg/kg in rats and 91–100 mg/kg in mice (CIR, 2015).
Repeated dose toxicity studies Because glycerol has been used extensively as a vehicle for drug delivery in many drug toxicology studies, Gad and colleagues (2006) surveyed four laboratories on their use of glycerol and other vehicles for in vivo experiments. They found the highest NOAEL was 500 mg/kg for guinea pigs and 15 g/kg for rats for 1 month of oral administration. A study in mice also reported a NOAEL for glycerol of 500 mg/kg for 90 days.
Numerous repeated dose studies, ranging from a few days to 2 years, have been conducted. Glycerol was administered in the diet of rats for 2 years at 5 percent and 10 percent of the diet. There were no pathological or toxicological effects noted, although food consumption increased in males (CIR, 2015).
Undiluted glycerol caused a variety of irritant-related effects, including petechial hemorrhage and erosions in the small intestine that were dose dependent. In several short-term feeding experiments, 20 percent glycerol administered in the diet for 4 weeks had no adverse effects, although an increase in kidney weights and increased liver enzymes were observed in more than half of the animals. Renne and colleagues (1992) established a NOAEL for glycerol of between 115 and 2,300 mg/kg when administered in drinking water to rats for 44 days. In another short-term drinking water study, calcification in kidney tubules between the cortex and medulla was observed in 3 of 5 rats administered 3,335 mg/kg/day glycerol in drinking water for 6 months (CIR, 2014).
A 3-day oral dosing study of glycerol in mixed-breed dogs established a NOAEL of 950 mg/kg/day. At the highest dose of 3,800 mg/kg/day, the stomach mucosa was severely hyperemic with petechial hemorrhages (Latven and Molitor, 1939). Another longer term feeding study in dogs using 35 percent glycerol in the diet found weight loss after 36 weeks. The weight loss continued after reduction of glycerol by 50 to 80 percent for the remainder of a 50-week study (CIR, 2015). Guinea pigs given 6,300 mg/kg/day of glycerol orally for 30–40 days showed no observable pathological changes (CIR, 2015; Ostwald, 1962).
Inhalation—non-human One study exposed rats for 6 hours per day, 5 days per week for 2 weeks to concentrations of 0, 1,000, 1,930 and 3,910 mg/m3 of aerosolized glycerol (Renne et al., 1992). The authors reported minimal squamous metaplasia of the epiglottis in 2/25, 1/19, 4/20, and 10/21 rats at 0, 33, 167, and 662 mg/L, respectively; one male in the high-dose group showed mild squamous metaplasia. The authors did not observe macroscopic or systemic effects, or changes in organ weights (Renne et al., 1992). They determined a lowest observed adverse effect level for local irritant effects on the upper respiratory tract of 1,000 mg/m3.
In another study by the same researchers, 11 rats exposed to the highest concentration of respirable glycerol for 13 weeks (6 hours per day, 5 days per week) similarly exhibited mild squamous metaplasia but did not display macroscopic changes or differences in organ weights (Renne et al., 1992). Male rats in the study showed reduced triglyceride levels, but there was no dose–response relationship (Renne et al., 1992). Based on this study, the inhalation NOAEL was 0.167 mg/L (Renne et al., 1992).
Reproductive and developmental toxicity A two-generation reproductive study of 10 rats administered glycerol (0, 20 percent; ~2,000 mg/kg/day in drinking water) for 8 weeks before mating until weaning of pups (CIR, 2015). The researchers observed no adverse effects on the reproductive efficiency of the parents (F0 generation), or the growth, fertility, or reproductive performance of the untreated F1 generation offspring. In the F0 generation, all 10 females became pregnant with similar litter size as the controls (9.0 versus 8.1). In the F1 generation, 9 of 10 females became pregnant. Additionally, there were no significant differences in the onset of estrus cycles, weight gain, and microscopic observations of the endocrine organs between the F1 and the F2 generations and the controls. Tissues from both the F1 and F2 generations showed no histological changes.
Another study administered glycerol (13.1, 60.8, 282, and 1,310 mg/kg/day) by gavage to Wistar rats (n = 25–28) on days 6 through 15 of gestation (CIR, 2015). No adverse effects were observed in the dams (NTIS, 1974). The number of pregnancies, implantations, resorptions, litter sizes, weights, and sex ratio, and the incidences of external, visceral, and skeletal abnormalities were similar among treatment groups compared with controls. The NOAEL for maternal toxicity and teratogenicity was 1,310 mg/kg/day.
A similar study administered glycerol (12.8, 59.4, 276, and 1,280 mg/kg/day) by gavage to CD-1 mice (n = 25) on days 6 through 15 of gestation. As with the study of Wistar rats, the researchers found no adverse effects in the dams (CIR, 2015), and the number of pregnancies, implantations, resorptions, litter sizes, weights, and sex ratio and incidences of external, visceral, and skeletal abnormalities were similar among treated mice compared with controls. The NOAEL for maternal toxicity and teratogenicity was 1,280 mg/kg/day.
A study of Dutch-belted rabbits (n = 25) administered glycerol (11.8, 54.8, 254.5, and 1,180 mg/kg/day) by gavage on days 6 through 18 of gestation, and also reported no adverse effects in the dams (CIR, 2015). Again, the number of pregnancies, implantations, resorptions, litter sizes, weights, sex ratio, and external, visceral, and skeletal abnormalities were similar among treated rabbits compared with controls. The NOAEL for maternal toxicity and teratogenicity was 1,180 mg/kg/day.
Male Fertility
One study found that glycerol injected into the testes of rats (50–200 µL and 862 mg/kg body weight) and monkeys (119 mg/kg body weight) suppressed spermatogenesis (CIR, 2015; Wiebe and Barr, 1984a,b; Wiebe et al., 1989).
Genotoxicity and carcinogenicity Numerous studies have examined the mutagenic potential of glycerol in the Ames Salmonella assay, in dose ranges from 0.2 to 50 mg/plate, and using a variety of strains of S. typhimurium, with and without metabolic activation, and all reported negative results (CIR, 2015; Clark et al., 1979; Doolittle et al., 1988; Haworth et al., 1983; Ishidate et al., 1984; Stolzenberg and Hine, 1979; Yamaguchi, 1982). Carmines and Gaworski (2005) measured the mutagenicity of mainstream tobacco smoke condensate in the presence and absence of various concentrations of glycerol (5, 10, and 15 percent) and found no difference in mutagenicity in the presence or absence of glycerol.
Glycerol also tested negative in the hypoxanthine-guanine phosphoribosyl transferase mutagenicity assay, sister chromatid exchange assay in Chinese hamster ovary cells, and unscheduled DNA synthesis in rat hepatocytes, at concentrations up to 1 mg/ml (CIR, 2015). Another study looking at interlaboratory comparisons of the DNA damage assay in rat hepatocytes evaluated glycerol as one of three “negative” vehicles for administration of other carcinogens, and confirmed the lack of any effect on DNA damage in rat hepatocytes (CIR, 2015). An in vivo bone marrow chromosome aberration assay tested negative following intraperitoneal injection administration of 1,000 mg/kg glycerol (CIR, 2015).
A chronic bioassay in rats, with glycerol administered at concentrations up to 20 percent for 1 year or up to 10 g/kg for 2 years, failed to increase tumor incidence (CIR, 2015). Thus, there is substantial evidence indicating that glycerol itself is not mutagenic. However, when combusted, glycerol can form thermal decomposition products (see the Carbonyl Compounds section for discussion of thermal decomposition products).
Ethylene Glycol
In addition to PG and glycerol, studies have also identified ethylene glycol as a solvent used in e-liquids. Ethylene glycol is an odorless, clear, slightly viscous liquid that is commonly used as antifreeze in cooling and heating systems, in hydraulic brake fluids, and as an industrial solvent. Hahn and colleagues (2014) identified ethylene glycol in samples even though it was not listed on any labels. Hutzler and colleagues (2014) found that ethylene glycol replaced PG and glycerol as the dominant compound in five products. In an e-liquid from one particular manufacturer, the ethylene glycol content was as high as 76 percent. Four out of five products from this particular manufacturer revealed more than 70 percent ethylene glycol, whereas only 2 percent was detectable in the fifth. Seven products from three manufacturers contained 1 to 6 percent ethylene glycol, and in one additional sample again more than 30 percent was detected. Conversely, altogether 15 samples produced by three other manufacturers tested negative. Most e-cigarette liquids do not contain ethylene glycol and, where present, it is at levels that are not likely to contribute significantly to adverse health effects. Nonetheless, ethylene glycol is a respiratory irritant and is associated with markedly enhanced toxicological hazards when compared with conventionally used glycerol and PG (Gomes et al., 2002).
FLAVORINGS
There are more than 7,000 unique e-liquid flavors available to e-cigarette users (Zhu et al., 2014), and yet, little is known about them as there are few studies examining exposure to flavorings. Furthermore, flavoring components are often not included in e-cigarette products' ingredient lists. For example, one study of 54 e-liquids found many products labeled with “natural or artificial flavors,” and just four samples listed specific flavoring substances (Hahn et al., 2014).
While the Flavor and Extracts Manufacturers Association considers many flavors to be GRAS in food products, at their levels of intended use, these chemicals could still be harmful when they are aerosolized and inhaled, as such ingredients are not safety tested for exposure routes other than ingestion (Barrington-Trimis et al., 2016; FEMA, 2015). For instance, saccharides, which are used to make sweet e-liquid flavors that can appeal to children (Farley et al., 2014; King et al., 2014; Villanti et al., 2013), degrade and produce furans and aldehydes when heated (Soussy et al., 2016). Aldehydes may cause irritation to the respiratory tract (Tierney et al., 2016). One study of 28 e-liquids identified more than 140 volatile flavoring components at concentrations varying from 1 to 5 percent (10 to 50 mg/ml), and detected the formation of aldehydes (Hutzler et al., 2014). Another study that tested multiple flavors in two brands of single-use cigarettes found a similar concentration of flavor chemicals and identified aldehydes such as vanillin and ethyl vanillin (Tierney et al., 2016). Hahn and colleagues (2014) analyzed 54 e-liquids and distinguished ethyl vanillin in 13 samples and thujone in 2 samples.
Other flavoring chemicals have been measured in e-liquids as well. For example, pulegone and eucalyptol were identified in menthol-flavored e-cigarettes (Lisko et al., 2015). Similar to combustible tobacco cigarettes, concentrations of menthol in this study varied from 3,700 to 12,000 µg/g. Additionally, 40 percent of non-menthol products tested in the study had low levels of menthol (Lisko et al., 2015). Menthol's properties include cooling and local anesthesia, as well as effects on drug absorption and metabolism, bronchodilation and respiration changes, and electrophysiology (Ahijevych and Garrett, 2004). Although little is known about the role of menthol in e-cigarettes, the effects of menthol on increasing the reinforcing effects of nicotine on tobacco smoking behavior were evidenced in both qualitative and quantitative empirical studies (Ahijevych and Garrett, 2010). For the menthol smokers, a greater exposure to nicotine and the particulate matter (tar) of the smoked cigarette was observed and can result in increased nicotine dependence and a greater chance of tobacco-attributable disease (Garten and Falkner, 2004).
Exposure to Flavorings
Broadly speaking, flavored tobacco use is associated with younger age; consumers perceive flavored tobacco products more favorably. Flavoring in tobacco products is considered an attractive characteristic and is associated with temporary experimentation and/or initiation of tobacco product use (Feirman et al., 2016; Kowitt et al., 2017). Flavors are extremely common among e-cigarette users, and are often named as a primary reason for e-cigarette use. For example, about 75 percent of regular e-cigarette users report using some non-tobacco flavor (Wang et al., 2015; Yingst et al., 2017). Despite the increasing popularity of e-cigarettes, little is known about users' preferences, selection, and switching among various flavors. Farsalinos and colleagues (2013) conducted an online survey of more than 4,000 e-cigarette users and found that flavors, especially flavor variety, were an important factor in the maintenance of e-cigarette use by current and former smokers. Specifically, nearly half of the study subjects reported that limiting the range of available e-cigarette flavors would increase cravings for combustible tobacco cigarettes and would decrease their likelihood of reducing or quitting smoking. The results also indicated that smokers tended to start with tobacco-flavored products, and then would switch to multiple flavors as they transitioned from dual use to complete (or nearly complete) substitution of e-cigarettes for their usual combustible tobacco cigarettes. Berg (2016) recruited 1,567 adults, ages 18 to 34 years, through Facebook ads targeting tobacco users and non-users. Fruity e-cigarette flavors were the most preferred among both smokers and non-smokers.
Flavors appear to hold value to users. In a willingness-to-pay (WTP) study, removing flavors resulted in an 18 percent drop in WTP among exclusive e-cigarette users, compared with a 1 percent drop for dual users (Nonnemaker et al., 2016). In a discrete-choice experiment context, flavor (cherry in particular) significantly increased intentions to purchase (Czoli et al., 2016). In the laboratory, participants worked harder for flavored puffs, meaning that flavors appear to enhance the reward/reinforcement value of nicotine (Audrain-McGovern et al., 2016). In a concept mapping study among vapers, five statement clusters around flavor use were identified: increased satisfaction and enjoyment, variety and customization, better feel and taste than cigarettes, food craving suppression, and social impacts (Soule et al., 2016). At the same time, data from novice users indicated that non-menthol flavorings were not associated with decreased cigarette consumption over 6 weeks of use (Litt et al., 2016).
In e-liquids, flavor combinations are common and their classification is not straightforward. This has been a limitation in determining preferred flavors among e-cigarette users, as common measures have not been used, resulting in widely divergent estimates across studies. A classification system with transparent decision rules that can be applied across product classes may yield more consistent findings to inform regulatory science (Yingst et al., 2017).
The role of menthol in e-cigarette users has not been studied. However, for combustible tobacco cigarettes, African American smokers report substantially greater preference for menthol cigarettes relative to smokers of European ancestry. This had led some to speculate that menthol may contribute to the greater incidence and severity of certain smoking-related diseases among African Americans. Certain studies also suggest that menthol may influence the rates of smoking initiation and cessation (TPSAC, 2011).
Although studies have dealt with flavoring chemicals in e-cigarette products, there is little information on how these chemicals affect health during long-term exposures by inhalation. Studies have shown that users switch among flavors frequently. Additionally, the choice of flavor may change over the course of a smoker's substitution of combustible tobacco with e-cigarettes, such that tobacco flavors are more popular when users start using e-cigarettes (Farsalinos et al., 2013). Flavoring compounds might also include substances of sensitizing, toxic, or irritating potency. Although few studies have examined the effects of flavoring substances administered by inhalation, there are some chemicals that, although approved for ingestion, have established adverse health effects when inhaled. Table 5-4 presents an overview of common flavorings and their inhalation toxicity. Examples of such chemicals include diacetyl, acetylpropionyl, acetoin, cinnamaldehyde, and benzaldehyde; these are reviewed in details below.
TABLE 5-4
Overview of Common Flavorings and Their Inhalation Toxicity.
Diacetyl, acetylpropionyl (also known as 2,3-pentanedione), and acetoin are chemicals used by food manufactures to add creamy flavors like butter, caramel, butterscotch, piña colada, and strawberry to food products. Acetylpropionyl is structurally similar to diacetyl and therefore can be used as a flavoring substitute. However, these ingredients have been associated with adverse respiratory health outcomes. For example, investigations in microwave popcorn manufacturing plants found increased incidences of chronic cough and bronchitis, asthma, and bronchiolitis obliterans, a severe lung condition that can result in permanent pulmonary scarring and obstruction (Kreiss et al., 2002; NIOSH, 2016). Workers in these facilities inhaled diacetyl and acetoin when butter flavoring containing these chemicals was heated and became aerosolized (Kreiss et al., 2002; NIOSH, 2016). Workers with bronchiolitis obliterans have also been found in flavoring production companies (NIOSH, 2016).
These flavoring ingredients have also been measured in e-cigarette liquids. For instance, a study of flavored e-cigarettes available in the United States identified at least one of these three chemicals in more than 90 percent of the tested e-cigarettes (Allen et al., 2016). Of the 51 samples, 46 flavors had acetoin (concentration ranging up to 529 µg per e-cigarette), 39 contained diacetyl (up to 239 µg per e-cigarette), and 23 flavors included acetylpropionyl (up to 64 µg per e-cigarette) (Allen et al., 2016). Another study of 159 sweet-flavored liquids from 36 American and European manufacturers found diacetyl and/or acetylpropionyl in nearly three-quarters of sampled liquids and their aerosols (Farsalinos et al., 2015c). These samples indicated a median daily exposure of 56 µg of diacetyl per day (interquartile range [IQR] = 26–278 µg/day); the median daily exposure to acetylpropionyl was 91 µg/day (IQR = 20–432 µg/day) (Farsalinos et al., 2015c).
Several studies examined the cinnamaldehyde-containing e-liquids and e-cigarette aerosols. Cinnamaldehyde is the major chemical in cinnamon-flavored e-cigarette products, but also has been found in tobacco-, sweet- (including caramel), and fruit-flavored e-liquids (Behar et al., 2016). Behar and colleagues (2016) evaluated the distribution, concentration, and toxicity of cinnamaldehyde in 39 e-liquids and aerosols generated from e-cigarettes. The study used the gas chromatography–mass spectrometry method and found that 20 of the 39 refill fluids contained cinnamaldehyde at concentrations that were cytotoxic to human embryonic and lung cells in the cell viability assay. The study also revealed that aerosol generated from a single product (cinnamon Ceylon) from a cartomizer-style e-cigarette was cytotoxic. The same product has been shown to be more cytotoxic when aerosol is generated with battery output voltage settings of 5 V than with 3 V, potentially due to additional chemicals released at higher voltage settings, including 2,3-butandione (diacetyl) as confirmed in the study. Cinnamaldehyde depolymerized microtubules in human pulmonary fibroblasts. At concentrations that produced no effect in the cytotoxicity assay, cinnamaldehyde decreased cell growth, attachment, and spreading; altered cell morphology and motility; increased DNA strand breaks; and increased cell death. In general, studies described above have shown that, even at low concentrations, cinnamaldehyde in e-cigarette products is cytotoxic and genotoxic and adversely affects cell processes and survival. These studies also indicate that cinnamaldehyde in e-cigarettes may impair homeostasis in the respiratory system.
Benzaldehyde, which imparts a fruity taste, is an aromatic aldehyde commonly used in food and cosmetics. Studies suggest that oral and dermal exposure to benzaldehyde produces little to no toxicity; however, occupational exposure has been linked to irritation of the eyes and mucous membranes of the respiratory passages (MAK Commission, 2002). One study measured benzaldehyde in aerosol generated from an e-cigarette refilled with 145 flavored nicotine-containing solutions purchased from international online retailers (Kosmider et al., 2016). The solutions were classified into groups according to labeled flavor characteristics: berry/tropical fruit (n = 40), tobacco (n = 37), alcohol-related/drink (n = 15), chocolate/sweet flavor (n = 11), coffee/tea (n = 11), mint/menthol (n = 10), cherry (n = 10), and other, non-identifiable flavor varieties (e.g, Indian summer and cosmopolitan) (n = 11). Benzaldehyde was present in 75 percent of 145 e-cigarette refill fluids, with the highest concentrations in cherry flavors. The benzaldehyde doses inhaled using 30 puffs from flavored e-cigarettes were often higher than doses inhaled from a combustible tobacco cigarette. The estimated median daily inhaled dose of benzaldehyde from cherry-flavored e-cigarettes was 70.3 µg, a level of exposure more than 1,000 times lower than the permissible exposure limit (PEL) of benzaldehyde as defined by the workplace environmental exposure level guides.
CARBONYL COMPOUNDS
It is important to evaluate the health effects of e-cigarettes when e-liquid is heated and aerosolized; under such conditions, chemical reactions may result in the formation of new compounds. For example, although refill liquids can contain carbonyl compounds such as reactive aldehydes, heating can enhance the concentrations of these compounds in the aerosol.
Several studies have shown that e-cigarettes emit toxic carbonyl compounds, generated from thermal decomposition of e-liquid ingredients. Carbonyl compounds such as formaldehyde, acetaldehyde, acrolein, and glyoxal, which have been found in e-cigarette aerosols, are potentially hazardous and may induce various health effects in users. Formaldehyde is classified as a human carcinogen (Group 1) by the International Agency for Research on Cancer (IARC), and acetaldehyde is classified as possibly carcinogenic to humans (Group 2B) (Bekki et al., 2014). Glycidol is a probable carcinogen and acrolein causes irritation of the nasal cavity and damages the lining of the lungs (ATSDR, 2007; NTP, 2007). How formaldehyde-releasing agents (hemiacetals) behave in the respiratory tract is currently unknown. Glyoxal and methylglyoxal show mutagenicity. The amount of carbonyl compounds in e-cigarettes varied significantly not only among different brands but also among different samples of the same products. Although, in most cases, detected levels of carbonyl compounds were lower than those in combustible tobacco cigarette smoke, very high levels of formaldehyde were also reported in e-cigarette aerosols (a comparison of toxicants from combustible tobacco cigarette smoke and e-cigarette aerosols is discussed in Chapter 18) (Canistro et al., 2017; Gillman et al., 2016).
Uchiyama and colleagues (2010, 2013) measured carbonyl compounds in e-cigarette aerosols using high-performance liquid chromatography (see also Bekki et al., 2014; Ohta et al., 2011). The authors tested 13 brands of Japanese e-cigarettes and detected several derivative peaks of carbonyl compounds, including formaldehyde, acetaldehyde, acetone, acrolein, propanal, crotonaldehyde, butanal, glyoxal, and methylglyoxal (Bekki et al., 2014; Ohta et al., 2011; Uchiyama et al., 2013). Four out of the 13 e-cigarette brands did not generate any carbonyl compounds. The other nine e-cigarette brands generated various carbonyl compounds. The maximum concentrations of formaldehyde, acetaldehyde, acrolein, propanal, glyoxal, and methylglyoxal were 140, 120, 40, 46, 23, and 21 µg/10 puffs, respectively.
Goniewicz and colleagues (2014) measured 15 carbonyl compounds in aerosol generated from 12 e-cigarette brands. Only four carbonyl compounds (formaldehyde, acetaldehyde, acrolein, and o-methylbenzaldehyde) were found in aerosols and these compounds were identified in nearly all examined e-cigarettes. The content of formaldehyde ranged from 2.0 mg to 56.1 mg, acetaldehyde from 1.1 mg to 13.6 mg, and acrolein from 0.7 mg to 41.9 mg per e-cigarette (150 puffs).
Kosmider and colleagues (2014) tested 13 samples of aerosol generated from Polish e-cigarettes and detected formaldehyde and acetaldehyde in 8 of them. The amounts of formaldehyde and acetaldehyde in e-cigarette aerosols at a lower voltage were on average 13- and 807-fold lower than those in combustible tobacco cigarette smoke, respectively. E-cigarette aerosols generated from PG-based e-liquids were found to have the highest levels of carbonyls. Furthermore, different e-cigarettes showed large variations in carbonyl levels.
Hutzler and colleagues (2014) measured formaldehyde in e-cigarette aerosol and estimated that exposure to formaldehyde can be comparable with combustible tobacco cigarettes. They measured 20 to 50 µg of formaldehyde per 10 puffs in the final fractions, which roughly corresponds to the expected exposure from smoking one combustible tobacco cigarette.
Flora and colleagues (2016) tested the aerosols of four MarkTen® e-cigarettes (rechargeable with disposable cartridges) for potential degradation products. They found formaldehyde levels that varied from 0.09 to 0.33 µg/puff. The same research team found formaldehyde residues in both the gas (approximately 30 percent) and liquid (approximately 70 percent) phases of an aerosol (Flora et al., 2017).
Blair and colleagues (2015) measured acrolein in aerosol from e-cigarettes and tobacco smoke and found that five puffs of an e-cigarette emitted 0.290 ± 0.018 µg of acrolein while nine puffs on a combustible tobacco cigarette emitted 2.61 ± 0.16 µg of this toxicant. There was a substantial range in the relative standard deviations reported for all mean value measurements, suggesting inconsistencies across products in the release of these chemicals.
Papousek and colleagues (2014) measured acrylamide and acrolein in tobacco smoke and three e-cigarette aerosol samples. The e-cigarette aerosol samples contained no detectable levels of acrylamide. Acrolein levels in combustible tobacco cigarette smoke varied from 4.48 to 8.27 µg per cigarette while levels detected in an equivalent sample of e-cigarette aerosol varied from 0.17 to 3.70 µg.
Sleiman and colleagues (2016) detected up to 31 compounds, including formaldehyde, acetaldehyde, glycidol, acrolein, acetol, and diacetyl, in e-cigarette aerosols from different devices. Emission rates were significantly higher for a single-coil versus a double-coil device, ranging from tens to thousands of nanograms of toxicants per milligram of e-liquid aerosol.
Tayyarah and Long (2014) tested 55 harmful and potentially harmful constituents in e-cigarette aerosol (blu and SKYCIG brands) and quantified three carbonyls (acrolein, acetaldehyde, and propionaldehyde) at levels 86 to 544 times lower than combustible tobacco cigarette smoke.
Table 5-5 summarizes experimental studies to determine carbonyl compounds in e-cigarette aerosols, their setups (i.e., methods to trap and analyze carbonyls, e-liquids used), and results. Because carbonyl compounds were primarily detected in aerosol and only traces have been reported in e-liquids, it has been suggested that these compounds are generated when e-liquid ingredients are heated. Figure 5-1 illustrates the pathways and by-products formed during thermal dehydration of PG and glycerol as postulated by Sleiman and colleagues (2016). Hutzler and colleagues (2014) incubated e-cigarette liquids at various temperatures and found levels of acetaldehyde and formaldehyde from 10-fold to 20-fold higher at the temperature of 150°C compared with ambient temperatures for samples containing PG. They did not observe this effect at 100°C.
TABLE 5-5
Summary of Experimental Studies Determining Carbonyl Compounds in E-Cigarette Aerosols.

FIGURE 5-1
Postulated pathways and by-products formed during thermal dehydration of propylene glycol and glycerol. SOURCE: Sleiman et al., 2016.
Several studies looked at the potential mechanisms for generating carbonyl compounds in e-cigarettes. In addition to temperature and effects from potential overheating, airflow and catalytic properties of metal heating coils may influence the occurrence of decomposition products. As described in the section on humectants, PG and glycerol can be a source of carbonyl compounds. It has been shown that the oxidation and fragmentation of PG and glycerol contained in e-liquids when they come in contact with the heating coil generates carbonyl compounds (Bekki et al., 2014; Geiss et al., 2016; Goniewicz et al., 2014; Ohta et al., 2011; Uchiyama et al., 2013). Lower liquid levels within the cartridges or tanks also seem to be associated with the occurrence of carbonyls, because low liquid levels may increase airflow and could therefore promote overheating of the wire if no safety features are incorporated to maintain a constant and lower temperature. Results reported by Geiss and colleagues (2016) confirmed that the PG oxidation is involved primarily in the formation of acetaldehyde, while the oxidation of glycerol typically generates acrolein. Oxidation of both PG and glycerol can generate formaldehyde, although a predominance of glycerol can be observed. Glycerol forms acrolein and acetaldehyde as oxidation by-products only at higher coil temperatures.
Gillman and colleagues (2016) demonstrated that glycerol can undergo thermal decomposition to form reactive aldehydes, including formaldehyde, acetaldehyde, and acrolein. The extent of formation is dependent upon both the power (watts) of the coil and the design of the device itself. Estimated exposures to total aldehydes from daily consumption of 3 grams of e-liquid ranged from less than 0.1 to 41 mg/day. Formaldehyde was the predominant aldehyde present, with the highest estimated exposure to be 22 mg/day. The authors reported a 750-fold difference in total aldehyde production between different devices, using the same e-liquid. For the device that generated the highest levels of aldehydes, the estimated daily doses exceed the OSHA occupational health PEL for formaldehyde by 10-fold.
Canistro and colleagues (2017) also found that heating of glycerol produces temperature-dependent amounts of formaldehyde, acetaldehyde, and acrolein (see Table 5-6). When rats were exposed via inhalation to e-cigarette aerosols (11 cycles/day, 5 days/week for 4 weeks), a statistically significant fourfold increase in the formation of 8-hydroxy-deoxy-guanosine was found in the lungs, along with other evidence of oxidative stress in these animals. Thus, it is likely that glycerol in e-liquids, under some circumstances that are both device- and power (watt)-dependent, can undergo thermal decomposition to generate reactive aldehydes capable of contributing to oxidative tissue injury, including potential DNA damage. However, for other devices, the levels of aldehyde were very low, relative to both typical indoor air and the levels found in combustible tobacco cigarette smoke. It should be noted that the conditions that resulted in very high levels of aldehydes were extreme and not typically attained during normal consumer use. Nevertheless, the potential exists for e-cigarette devices to form very high levels of aldehydes under extreme conditions.
TABLE 5-6
Volatile Compounds Detected in E-Cigarette Aerosol.
Some e-cigarette devices allow users to change the power of the device or output voltage of the battery to increase aerosol production and nicotine delivery. The battery output voltage, and consequently the heat generated on the coil, has been reported to affect the quantity of carbonyls formed. Kosmider and colleagues (2014) showed that increasing the voltage from 3.2 V to 4.8 V resulted in an increase from 4 to more than 200 times in the levels of formaldehyde, acetaldehyde, and acetone. The levels of formaldehyde in aerosol generated from high-voltage devices were nearly identical to those in combustible tobacco cigarette smoke (1.6–52 µg per cigarette) (see Figure 5-2).

FIGURE 5-2
Effects of nicotine solvent and battery output voltage on levels of carbonyl compounds released from e-cigarettes (µg/15 puffs; n = 3; puff duration = 1.8 seconds, puff volume = 70 ml, puff intervals = 17 seconds). NOTES: * = statistically significant (more...)
Increasing levels of carbonyl compounds were observed for a voltage over 3 V (Bekki et al., 2014; Ohta et al., 2011). Thus, commercial e-cigarettes with 4- to 5-V batteries may generate carbonyl compounds. The battery output voltage significantly affects the concentration of carbonyl compounds in the e-cigarette aerosol, and high-voltage e-cigarettes may expose users to high levels of carbonyl compounds.
Formaldehyde also reacts with PG and glycerol during aerosolization to produce hemiacetals. Jensen and colleagues (2015) analyzed commercial e-liquid aerosolized with the use of a tank system e-cigarette featuring a variable-voltage battery. They detected no formation of any formaldehyde-releasing agents at 3.3 V. However, at 5.0 V, they detected a mean ± SE of 380 ± 90 µg/sample (10 puffs) of formaldehyde as hemiacetals. Similarly, Sleiman and colleagues (2016) found that when they increased the voltage applied to a single-coil device from 3.3 to 4.8 V, the mass of e-liquid consumed doubled from 3.7 to 7.5 mg/puff and the total aldehyde emission rates tripled from 53 to 165 µg/puff, with acrolein rates growing by a factor of 10.
Flora and colleagues (2017) evaluated the effect of e-cigarette heating coil temperature on formaldehyde formation. Using an infrared camera to measure the maximum heat coil temperature and Fourier-transform infrared spectrometer to measure gas-phase formaldehyde, the authors found that, in some of the commercial e-cigarettes tested, the levels of formaldehyde were greater than those detected in combustible tobacco cigarettes, and as high as 14.1 µg/puff. The study found that e-cigarettes produce low amounts of formaldehyde at temperatures below 350°C, but as the temperature increases, the levels of formaldehyde also rise steeply. The authors concluded that the high levels of formaldehyde observed in some e-cigarettes tested in the study were likely due to heating coil temperatures above 350°C.
Geiss and colleagues (2016) also reported correlation between the amounts of carbonyl compounds emitted by e-cigarettes with the temperature of the heating coil. The authors used infrared thermography to determine the temperature of the heating coil and had an experienced e-cigarette user conduct a subjective sensorial quality evaluation of the aerosol generated at each temperature. The study found a steep increase in the generated carbonyls when applying a battery output of at least 15 W corresponding to 200°–250°C on the heating coil. At 20 W, the e-cigarette user provided a negative sensorial quality evaluation, suggesting that an e-cigarette user would be unlikely to apply such wattage in real-world use.
Wang and colleagues (2017) investigated how PG and glycerol influence carbonyl compound formation under precisely controlled temperatures in the absence of nicotine and flavor additives. At reactor temperatures equal to or greater than 215°C for both PG and glycerol, the authors detected significant amounts of formaldehyde and acetaldehyde. Only e-liquids containing glycerol at temperatures exceeding 270°C produced acrolein. At 318°C, 2.03 ± 0.80 µg of formaldehyde, 2.35 ± 0.87 µg of acetaldehyde, and a trace amount of acetone were generated per milligram of PG; at the same temperature, 21.1 ± 3.80 µg of formaldehyde, 2.40 ± 0.99 µg of acetaldehyde, and 0.80 ± 0.50 µg of acrolein were detected per milligram of glycerol.
Other factors causing elevated carbonyl levels should also be considered. It is expected that both the heating element and wicking material will deteriorate with use, which could lead to more thermal degradation (Guthery, 2016). Sleiman and colleagues (2016) found that, after an e-cigarette device was used several times, carbonyl emissions increased by more than 60 percent, and they attributed this effect to the buildup of polymerization by-products that degraded upon heating. Flavoring compounds may also play a role. Using three popular brands of e-cigarettes filled with both flavored and unflavored e-liquids, Khlystov and Samburova (2016) measured several toxic aldehydes and showed that the formation of aldehydes during e-cigarette use comes primarily from thermal decomposition of flavoring compounds. They also found that the production of aldehydes was exponentially dependent on concentration of flavoring compounds. Sucrose, a sweetener and flavor enhancer detected in e-liquids in concentrations from 0.76 to 72.93 µg/g, also has been suggested as a potential ingredient that may thermally degrade to produce carbonyl compounds (Kubica et al., 2014).
Several studies have examined the potential exposure to carbonyl compounds from e-cigarettes. Using American Conference of Governmental Industrial Hygienists (ACGIH) standards, Khlystov and Samburova (2016) assessed e-cigarette users' carbonyls exposure risk from e-cigarettes. ACGIH defines the threshold limit value–ceiling (TLV–C) as the concentration that should not be exceeded during any part of the working exposure; the TLV-C for formaldehyde is 0.3 mg m3, and, for acrolein, is 0.23 mg/m3. To compare exposure to these aldehydes from one puff, the authors divided the amount per puff by 500 ml, the average tidal volume of a healthy adult, and found that all flavored products of a single brand exceeded the ACGIH formaldehyde ceiling level by factors of 190–270 and the acrolein ceiling level by factors of 11–24, depending on the flavor used. Three of five liquids of the second brand tested exceeded the formaldehyde ceiling level by 2.0-fold to 13-fold, depending on the liquid flavor. No acrolein was detected in the second brand tested. All flavored products of the third brand tested exceeded the formaldehyde ceiling level by 2.9-fold to 66-fold and four products of the same brand exceeded the acrolein ceiling by 1.5-fold to 6.0-fold. The authors concluded that one puff of any of the tested flavored e-cigarette liquids exposes the smoker to dangerous levels of these two aldehydes.
Jensen and colleagues (2015) extrapolated the formaldehyde dose from levels of formaldehyde-releasing agents (hemiacetals) detected in aerosol generated at high voltage. The high battery output voltage setting (5 V) used by Jensen and colleagues resulted in excessive breakdown of PG to formaldehyde. The estimated daily dose of formaldehyde-releasing agent for an e-cigarette user vaping at a rate of 3 ml/day would be as high as 14.4 ± 3.3 mg. This dose is much higher than the estimated daily dose of formaldehyde from combustible tobacco cigarettes, which is approximately 3 mg/pack of 20 combustible tobacco cigarettes (150 µg/cigarette). Under the assumption that the risk per unit associated with inhaling formaldehyde-releasing agents is the same as the risk associated with inhaling gaseous formaldehyde, the authors estimated that long-term e-cigarette use is associated with an incremental lifetime cancer risk from inhaling formaldehyde of 4.2 × 10−3. This risk is from 5 to 15 times higher than the risk associated with inhaling formaldehyde during long-term combustible tobacco smoking.
Wang and colleagues (2017) estimated that the daily exposure to formaldehyde and acetaldehyde for an e-cigarette user vaping at 215°C could reach 105 ± 117 µg and 36 ± 42 µg, respectively. This estimated daily formaldehyde exposure is above the “no significant risk level” of 40 µg/day from the California Office of Environmental Health Hazard Assessment (OEHHA, 2013). Same authors estimated that if the e-cigarette heating temperature exceeds 270°C, the formaldehyde generated from 10 50-ml puffs could reach levels similar to those from combustible tobacco smoking (comparisons of exposure to potentially toxic substances from e-cigarettes with combustible tobacco cigarette smoking are described in more detail in Chapter 18 on harm reduction).
In summary, when e-liquids are heated and aerosolized, they can produce chemical reactions that could form carbonyl compounds such as reactive aldehydes, which are considered to have toxic effects on human health. At temperatures within the range of most e-cigarette products (150°–350°C), formaldehyde, acetaldehyde, and acrolein have been detected at levels that have raised concerns about chronic health endpoints (Jensen et al., 2015).
MINOR TOBACCO ALKALOIDS
Although the main alkaloid found in tobacco-derived products, including e-liquids, is nicotine, several minor tobacco alkaloids have been identified. The process by which nicotine in e-liquids is extracted from tobacco may produce some impurities including minor alkaloids: nornicotine, anatabine, anabasine, cotinine, nicotine N-oxides, myosmine, β-nicotyrine, and β-nornicotyrine. These minor alkaloids may arise from biosynthetic processes in the living plant or by bacterial action or oxidation during tobacco processing (Gorrod and Jacob, 1999).
Etter and colleagues (2013) analyzed samples of e-liquids from 20 bottles of 10 different brands using ultra-high-performance liquid chromatography, and found that minor tobacco alkaloids constituted 1–2 percent of the nicotine content in most samples. The most common substances found were cis-N-oxide, trans-N-oxide, myosmine, anatabine, and anabasine. The authors hypothesized that oxidative degradation of nicotine during the manufacturing of the ingredient or of the final liquids, interactions with packaging material, inadequate handling and storage, or an unstable formulation could have resulted in the high amounts of nicotine-related impurities measured.
Testing nicotine-containing e-liquids, Lisko and colleagues (2015) found minor tobacco alkaloids in all samples, and observed that their relative concentrations varied widely among manufacturers. eSmoke brand e-liquids had the highest concentrations of the minor tobacco alkaloids (6.3–48.2 µg/g nornicotine, 8.7–62.7 µg/g myosmine, 21.2–152 µg/g anabasine, 63.1–485 µg/g anatabine, and 2.4–20.7 µg/g isonicoteine). Other products tested contained considerably lower concentrations of minor tobacco alkaloids. These variations could be due to use of purer nicotine extract or minimization of nicotine oxidation. These minor tobacco alkaloid concentrations in e-liquids are much lower when compared with combustible tobacco cigarettes, which have minor tobacco alkaloid concentrations in the range of 659–986 µg/g for nornicotine, 8.6–17.3 µg/g for myosmine, 127–185 µg/g for anabasine, 927–1,390 µg/g for anatabine and 23.4–45.5 µg/g for isonicoteine (comparisons between e-cigarettes and combustible tobacco cigarettes are described in more detail in Chapter 18 on harm reduction).
Flora and colleagues (2016) tested the liquids and aerosols of four MarkTen® e-cigarettes (rechargeable with disposable cartridges) for potential impurities and degradation products. They found that liquids contained 11–19 µg/g of nicotine N-oxides, undetectable levels to 9.4 µg/g of cotinine, 14–31 µg/g of nornicotine, and 7.4–13.0 µg/g of myosmine. Regueiro and colleagues (2016) tested 12 e-cigarette liquids purchased from different vendors in the European Union. Among the nicotine-related compounds studied, the authors detected only anatabine, cotinine, myosmine, and nornicotine in any of the samples, and at concentrations in the microgram-per-milliliter level.
Nicotine dehydrogenation also results in another alkaloid: nicotyrine. Considerable quantities of this nicotine analogue have been measured in an analysis of various e-cigarette aerosols (Martinez et al., 2014). Nicotyrine has been shown to hinder nicotine metabolism in mice (Stålhandske and Slanina, 1982). Therefore, its presence in e-cigarette aerosols could diminish smoking cravings by aiding nicotine absorption in the lungs, restraining metabolism, and consequently maintaining nicotine levels (Martinez et al., 2014).
Nicotine purity varies by grade and manufacturer. The American E-Liquid Manufacturing Standards Association requires members to use U.S. Pharmacopeia (USP)-certified nicotine in e-liquids, although the group does not have regulatory authority (AEMSA, 2014). According to USP standards, nicotine solutions cannot exceed 0.5 percent (5 mg/g) of a single impurity or 1 percent (10 mg/g) of total impurities (U.S. Pharmacopeia, n.d.). Nicotine-related impurities are less toxic than nicotine, but the health effects of these minor tobacco alkaloids to e-cigarette users, especially at high levels, is unknown.
TOBACCO-SPECIFIC NITROSAMINES
TSNAs are potent carcinogenic chemicals (Hecht, 1998; Hecht and Hoffmann, 1988), which are derived from tobacco leaves and formed during the curing process via nitrosation of amines. Low levels of TSNAs have been reported in e-cigarette liquids and aerosol, typically at levels similar to those found in pharmaceutical nicotine products. This is probably attributed to the use of pharmaceutical-grade nicotine that most manufacturers claim to use. This grade of nicotine is highly purified to remove the majority of impurities, including TSNAs.
Using liquid chromatography–tandem mass spectrometry, Kim and Shin (2013) detected TSNAs in 105 refill liquid brands purchased from 11 e-cigarette companies in the Korean market. They measured TSNAs in concentration ranges of 0.34–60.08 µg/L (64.8 percent detection frequency) for N'-nitrosonornicotine (NNN), 0.22–9.84 µg/L (88.6 percent detection frequency) for 4-(methylnitrosamino)-1-(3-pyridyl)-1-butanone (NNK), 0.11–11.11 µg/L (54.3 percent detection frequency) for N'-nitrosoanabasine (NAB), and 0.09–62.19 µg/L (75.2 percent detection frequency) for N'-nitrosoanatabine. Farsalinos and colleagues (2015b) evaluated the presence of selected tobacco-derived chemicals in liquids produced by extracting flavor from cured tobacco leaves and found that total nitrosamine concentrations varied from 2.5 to 38.5 ng/ml. In another study, Farsalinos and colleagues (2015a) also compared the levels of TSNAs in three commercial e-liquids and the aerosol from three 100-puff sets from each liquid trapped in filter pads. In two of the liquids, NAB was found at trace levels (1.2 and 2.3 ng/g); the third contained 1.5 ng/g NAB and 7.7 ng/g NNN (Farsalinos et al., 2015a). The authors found no TSNAs in the aerosol from the 100-puff sets. Finally, Goniewicz and colleagues (2014) analyzed aerosol generated from 12 brands of e-cigarette and identified two nitrosamines (NNN and NNK) in all but three products. The NNN yields ranged from 0.8 ng to 4.3 ng and the NNK yields from 1.1 ng to 28.3 ng per 150 puffs.
FREE RADICALS AND REACTIVE OXYGEN SPECIES
Reactive oxygen species (ROS), including free radicals, can stem from normal biological processes as well as from external sources, such as tobacco smoke. ROS cause oxidative stress, which damages cellular proliferation, metabolism, and health, and can be involved in the development of several cardiovascular (e.g., atherosclerosis), respiratory (e.g., chronic obstructive pulmonary disease, asthma), and neurodegenerative disorders (e.g., Parkinson's disease, multiple sclerosis) as well as diabetes, rheumatoid arthritis, and some types of cancers (e.g., lung, colorectal) (Domej et al., 2014; HHS, 2010; Kehrer and Klotz, 2015; Kirkham and Rahman, 2006; Messner and Bernhard, 2014; Phaniendra et al., 2015; Prescott and Bottle, 2017; Pryor, 1997).
E-cigarette users may be exposed to both highly reactive and more stable ROS during use. Activating the e-cigarette's heating element and aerosolizing the e-liquid produce ROS; these species are drawn into the lungs directly from the device (Lerner et al., 2015b). This process is affected by the age of the heating element (Lerner et al., 2015a). Oxidants are also derived from a device's lithium ion battery, similar to that used in combustible tobacco cigarette filters and e-cigarette cartomizers (Lerner et al., 2015a). Goel and colleagues (2015) identified free radicals from all e-cigarettes and e-liquids tested (at 3.3 V, 2.5 × 1013 to 10.3 × 1013 radicals per puff), as well from glycerol and PG and during dry-puff scenarios. Sussan and colleagues (2015) found 7 × 1011 free radicals per puff. In their mouse model, these free radicals caused oxidative stress and airway inflammation and disrupted antibacterial and antiviral responses. Lerner and colleagues (2015b) similarly detected free radicals in a popular e-cigarette brand. In examining unaerosolized e-liquids, the authors found tobacco flavors were weaker oxidizers than sweet or fruity flavors (Lerner et al., 2015b).
OTHER TOXICANTS
Volatile Organic Compounds and Phenols
Lim and Shin (2017) tested flavored e-liquids (n = 283), nicotine liquids (n = 21), and disposable cartridges (n = 12) and detected 14 VOCs, including alcohols. Specifically, they detected VOCs in the following concentration ranges: benzene (0.008–2.28 mg/L), toluene (0.006–0.687 mg/L), ethylbenzene (0.01–1.21 mg/L), m-xylene (0.002–1.13 mg/L), p-xylene (0.007–2.8 mg/L), o-xylene (0.004–2.27 mg/L), styrene (0.011–0.339 mg/L), ethyl acetate (0.3–669.9 mg/L), ethanol (16–38,742 mg/L), methanol (66–3,375 mg/L), pyridine (0.077–99.7 mg/L), acetylpyrazine (0.077–147 mg/L), 2,3,5-trimethylpyrazine (0.008–96.8 mg/L), and octamethylcyclotetrasiloxane (0.1–57.2 mg/L). According to the authors, the use of petrogenic hydrocarbons as a solvent in the extraction of flavor compounds and nicotine from natural plants may have produced benzene (classified as a Group 1 carcinogen by IARC), toluene, ethylbenzene, m-xylene, p-xylene, and o-xylene. The maximum detected concentrations of benzene, methanol, and ethanol in the samples were higher than their authorized maximum limits as residual solvents in pharmaceutical products. Farsalinos and colleagues (2015b) evaluated the presence of selected tobacco-derived chemicals in liquids produced by extracting flavor from cured tobacco leaves and found nitrate (levels varied from undetectable to 317.9 µg/ml) and small amounts of phenols (total average 1.5 µg/ml), including catechol, m-cresol and o-cresol, and phenol. Goniewicz and colleagues (2014) measured 11 VOCs in aerosol generated from 12 brands of e-cigarettes. Among 11 VOCs analyzed, only two (toluene and m- and p-xylene) were found in almost all examined e-cigarettes. The yields of toluene ranged from 0.2 mg to 6.3 mg per one e-cigarette (150 puffs). Although the m- and p-xylene levels found in analyzed samples of e-cigarette aerosol ranged from 0.1 mg to 0.2 mg/150 puffs, it was also found at the same level in blank samples.
Microorganisms and Residual Solvents
Varlet and colleagues (2015) analyzed 42 models from 14 brands of refill liquids for e-cigarettes for the presence of microorganisms, diethylene glycol, ethylene glycol, hydrocarbons, ethanol, and solvents. All of the products tested contained some potentially toxic compounds. The authors detected diethylene glycol, ethylene glycol, and ethanol at levels within limits permitted for food and pharmaceutical products. The authors also found terpenic compounds and residual solvents such as 1,3-butadiene, cyclohexane, and acetone in some products. In compliance with norms, none of the liquids contained yeast, mold, aerobic microbes, Staphylococcus aureus, or Pseudomonas aeruginosa.
Furans
The thermal degradation of sugars can produce toxic furans, such as 5-hydroxymethylfurfural and furfural. Furfural is known to cause irritation to the upper respiratory tract in humans (Arts et al., 2004), and both furanic compounds show tumorigenic activity in mice (Irwin, 1990; Surh and Tannenbaum, 1994; Surh et al., 1994). Soussy and colleagues (2016) investigated the formation of furanic compounds in e-cigarette aerosols using e-liquids of varying sweetener concentrations and devices under different power settings and puff durations. The authors detected both 5-hydroxymethylfurfural and furfural in the aerosols of sweet-flavored e-liquids. Levels of furans in the e-cigarette emissions were significantly correlated with power of the device and sweetener concentration, but not puff duration. The formation of furanic compounds from a sugar alcohol was negligible.
Phthalates
A recent study found diethyl phthalate (DEP) and diethylhexyl phthalate (DEHP) in e-liquids, although the quantified levels in the study's sample were below phthalate exposure limits (Oh and Shin, 2015). DEP can be a solvent or plasticizer and is found in variety of consumer products, including fragrances, cosmetics, and detergent bases. DEHP is a plasticizer often used in making polyvinyl chloride products. These antiandrogenic, estrogen-like compounds have been shown to initiate early breast development; IARC classifies DEHP as “possibly carcinogenic to humans” (IARC, 2000, p. 529). Researchers hypothesize that DEP and DEHP originated from the e-liquid packaging or during the e-liquid production process (Oh and Shin, 2015).
Caffeine
E-liquid flavors like coffee, tea, chocolate, and energy drinks, which are associated with having caffeine, often contain caffeine at concentrations significantly lower than their dietary counterparts. Lisko and colleagues (2015) measured caffeine concentrations in 44 flavored e-liquids from cartridges, disposables, and refill solutions. The researchers chose flavors traditionally associated with caffeine, marketed as energy boosters, or labeled as containing caffeine by the manufacturer. They detected caffeine in 42 percent of coffee-flavored products, 66 percent of tea-flavored products, and 50 percent of chocolate-flavored e-liquids in concentrations ranging from 3.3 µg/g to 703 µg/g. They did not detect caffeine in energy drink–flavored e-liquids. Eleven of 12 products marketed as energy enhancers contained caffeine in concentrations that varied substantially, ranging from 31.7 µg/g to 9,290 µg/g. Although the estimated caffeine exposures from e-cigarettes are at levels significantly lower than those from drinking caffeinated beverages, very little is known about the effects of caffeine inhalation, and health risks cannot be estimated.
Pharmaceutical Drugs
In addition to the toxicants described above, although rare, e-cigarette users may also be exposed to pharmacological components in their devices' e-liquids. For example, one study found evidence of a weight-loss medication (rimonabant), originally approved in Europe, in an analysis of e-liquids (Hadwiger et al., 2010). This treatment has been associated with adverse neurological events such as seizures and suicide, and is not approved by FDA (2007). Furthermore, this study also found e-liquid can contain an analogue (amino tadalafil) to the active ingredient in Cialis, an erectile dysfunction drug (Hadwiger et al., 2010). The potential exposure to medicinal compounds in some e-liquids places users at risk of experiencing undetermined or harmful health effects.
SYNTHESIS
- Many chemicals other than nicotine have been identified in liquids and aerosols generated from e-cigarettes.
- Compounds not listed on labels also have been identified in e-liquids.
- Several hazardous compounds have been found in liquids and in the heated aerosol produced by e-cigarettes, including formaldehyde, acetaldehyde, and acrolein, which are known carcinogenic toxicants.
- Of greater concern are the added flavorings that are considered safe for use in food, but have not been widely tested for sensitizing, toxic, or irritating potency.
- E-cigarettes are a source of extremely high particulate doses in the human respiratory system. Fine particles are emitted when humectants (mostly PG and glycerol) are aerosolized.4
Conclusion 5-1. There is conclusive evidence that in addition to nicotine, most e-cigarette products contain and emit numerous potentially toxic substances.
Conclusion 5-2. There is conclusive evidence that, other than nicotine, the number, quantity, and characteristics of potentially toxic substances emitted from e-cigarettes are highly variable and depend on product characteristics (including device and e-liquid characteristics) and how the device is operated.
Conclusion 5-3. There is substantial evidence that except for nicotine, under typical conditions of use, exposure to potentially toxic substances from e-cigarettes is significantly lower compared with combustible tobacco cigarettes.
METALS
As discussed above, research on the chemical constituents of e-cigarettes has generally focused on nicotine, the carcinogens formaldehyde and acetaldehyde, flavoring compounds, and particles. An increasing number of studies have also found toxic metals such as lead, nickel, and chromium in e-liquid emissions (Aherrera et al., 2017; Farsalinos et al., 2015b; Goniewicz et al., 2014; Hess et al., 2017; Lerner et al., 2015a; Mikheev et al., 2016; Williams et al., 2013, 2017). Metal exposure may originate from several parts of the device, including the metallic coil, a complex alloy that heats the e-liquid to produce the aerosol that is inhaled by the user (Aherrera et al., 2017; Hess et al., 2017; Olmedo et al., 2018; Williams et al., 2017). Other parts of the device, such as the joints and wires, could also contribute. For example, Kanthal, an alloy frequently used in e-cigarettes, contains aluminum, chromium, and iron. Other common alloys are Ni-200, which is made of nickel, and nichrome, which includes chromium and nickel. Furthermore, metals such as tin have been found in the joints (Williams et al., 2017). E-liquids may also contain metals at varying concentrations. For instance, some e-liquid solutions contain arsenic (Beauval et al., 2016; Mikheev et al., 2016).
A small number of studies have investigated the role of e-cigarette aerosols in metal exposure. Most of these studies have evaluated one or two devices to measure metals in e-cigarette emissions and assess which metals are in higher concentrations compared with other metals, as well as to compare metals found in e-cigarette emissions and tobacco smoke. For example, Saffari and colleagues (2014) used quartz filters to study emission rates of a European tank-style device and found evidence of several metals. The authors detected boron (mean emission rate, ng/h: 964), cadmium (0.480), chromium (28.1), lanthanum (3.21), lead (96.2), nickel (131), potassium (7,765), silver (20.9), titanium (50.2), and zinc (1,142), but did not identify aluminum, copper, iron, or tin. However, the particle-sampling method the authors used in this study could have failed to distinguish metals during the aerosol phase. A study by Goniewicz and colleagues (2014) assessed metal concentrations in aerosols from a pharmaceutical nicotine inhaler and 12 e-cigarettes. Metals, including cadmium (concentrations varied from undetectable to 0.22 µg/150 puffs), lead (0.03 to 0.57 µg/150 puffs), and nickel (0.11 to 0.29 µg/150 puffs), were found in most of the samples tested. Mikheev and colleagues (2016) used quartz filters and inductively coupled plasma mass spectrometry to study metals in aerosols from a tank-style device and cigalike products. The authors measured antimony (0.05 to 0.50 ng/mg), arsenic (0.01 to 0.70 ng/mg), chromium (0.40 to 5.0 ng/mg), copper (0.05 to 5.0 ng/mg), nickel (0.05 to 5.0 ng/mg), tin (0.02 to 0.50 ng/mg), and zinc (1.50 to 50.0 ng/mg) in most samples, but did not measure lead. In another study, Williams and colleagues (2013) detected aluminum, iron, nickel, silver, and tin in particles greater than 1 µm from one brand's 22 cigalike cartomizers; nanoparticles (less than 100 nm) had chromium, nickel, and tin. The authors also used inductively coupled plasma optical emission spectrometry to identify lead (0.017 µg/10 puffs).
One of the key hypotheses is that metals in the coil leach during the heating process into the generated aerosol. For instance, Williams and colleagues (2013) describe the coil in their study of 22 cartomizers as a nickel-chromium filament soldered with tin to a thicker, silver-coated copper wire. The thick, copper-silver wire was also attached to the air tube and mouthpiece at tin solder joints. The same study group detected 35 of 36 selected elements in electronic hookahs and disposable e-cigarettes; in comparison, the authors found 15 of these elements in combustible tobacco cigarette smoke (Williams et al., 2017). Some metals, like copper, lead, nickel, and tin, were quantified at significantly higher concentrations in e-cigarette aerosols than combustible tobacco smoke, while levels of cadmium were lower. In an analysis of disposable e-cigarette wires and joints using electron microscopy and energy-dispersive X-ray spectroscopy, nickel, chromium, copper, silver, zinc, iron, aluminum, tin, calcium, and lead were clearly detected in different parts of the device (see Figure 5-3).

FIGURE 5-3
Scanning electron microscopy and energy dispersive X-ray spectroscopy analysis of disposable e-cigarette/e-hookah wires and joints. NOTES: (A) Scanning electron micrograph of the clamp joining thick and thin wires (red arrow) in BluCig. The filaments (more...)
While many of the studies on e-cigarettes and metals have been done with first- or second-generation devices, a recent study has compared metal concentrations in e-liquid before being in contact with the device to metal concentrations in the aerosol generated after heating the coil of 56 modified e-cigarette devices from daily e-cigarette users (Olmedo et al., 2018). In the study, major increases in metal concentrations were found in aerosol samples compared with e-liquid samples for lead and zinc (increases greater than 2,000 percent) and chromium, nickel, and tin (increases greater than 600 percent). The finding of lead in e-cigarette aerosol samples, a metal not listed among the components of heating coils but that can be present in metal alloys or may be in some other parts of the device, can be of concern. Aerosol mass concentrations for the detected metals (nickel, chromium, lead, and manganese) spanned several orders of magnitude and exceeded current occupational or environmental standards for 50 percent of samples or more. In that study, 10 percent of the e-liquid samples had detectable arsenic concentrations, and the levels remained similar in the aerosol (Olmedo et al., 2018).
E-liquids may also acquire metals after they come in contact with e-cigarette coils. For example, one study found cadmium (mean concentration varied from 0.42 to 205 µg/L), chromium (53.9 to 2,110 µg/L), lead (4.89 to 1,970 µg/L), manganese (28.7 to 6,910 µg/L), and nickel (0.059 to 22.6 µg/L) in e-liquids touching unused cartomizer coils from five different cigalike brands (Hess et al., 2017). By measuring five devices of each of five brands, this study illustrates the substantial variability within and across brands, especially for chromium, manganese, nickel, and lead (see Figure 5-4). Beauval and colleagues (2016) found generally low concentrations of metals in a study of e-liquids, with the exceptions of copper, nickel, and zinc (20, 16, and 200 µ/L, respectively). Furthermore, arsenic was measured in 57 percent of samples (mean concentration of 1.57 µg/L).

FIGURE 5-4
Distribution of metal concentrations within and across brands of disposable e-cigalike devices. NOTES: Horizontal lines within boxes indicate medians; boxes, interquartile ranges; error bars, values within 1.5 times the interquartile range; solid circles, (more...)
So far, only one published study has compared metal concentrations in e-cigarette emissions to metal biomarker concentrations in an e-cigarette study. In that study, conducted among 64 daily e-cigarette users (59 using second- and third-generation devices and 5 using first-generation cigalikes), the levels of chromium and nickel were measured in several samples collected from the e-cigarette device (dispenser, aerosol, and tank) used by the participant, as well as in several biomarkers collected non-invasively: urine, saliva, and exhaled breath condensate (EBC); and data on e-cigarette use (Aherrera et al., 2017). Median nickel and chromium levels were 0.73 and 0.39 µg/g creatinine, respectively, in urine; 2.25 and 1.53 µg/L in saliva; and 1.25 and 0.29 µg/L in EBC. In adjusted models, tertiles 2 and 3 of aerosol nickel concentrations were associated with 16 percent and 72 percent higher urine nickel and 202 percent and 321 percent higher saliva nickel compared with the lowest tertile. Tertile 3 of aerosol chromium levels was associated with 193 percent higher saliva chromium. An earlier time to first vape in the morning and more frequent coil change were associated with higher urine nickel. Tertile 2 of e-liquid consumption per week and voltage were associated with higher saliva nickel levels than tertile 1. Therefore, this study presents evidence that participants' internal doses of chromium and nickel were positively associated with e-cigarette aerosol concentrations. Additional research is needed to evaluate the association between metal levels in e-cigarette emissions and metal biomarkers, as well as comparing metal biomarker levels in e-cigarette users and a comparable group of non-users.
Exposure to metals through e-cigarettes is relevant as certain metals can cause serious health effects. For example, lead exposure is associated with neurotoxicity (Garza et al., 2006) and cardiovascular disease (Navas-Acien et al., 2007), and chromium(VI) and nickel have been associated with respiratory diseases such as lung cancer (IARC, 2012, 2017; Jaishankar et al., 2014). Nickel can also induce an allergic response in some individuals. Several cases of nickel-induced allergic dermatitis have been related to e-cigarette use (Maridet et al., 2015; Ormerod and Stone, 2017). Another concern is that metal absorption is markedly higher through inhalation, as compared with ingestion, and that while some of the metals found in e-cigarette aerosol are essential elements when ingested (zinc, manganese, copper, and chromium[III]), exposure to these metals through inhalation tends to be toxic (Goyer and Lavoie, 2001; Tchounwou et al., 2012). For chromium, no study has measured the valence state and it is currently unknown if the form of chromium in the aerosol is chromium(III) or chromium(VI). The implications could be major as chromium(VI) is an established carcinogen.
Few studies have measured the toxic characteristics of metals in e-cigarette aerosols, although in principle, metal toxicity would not necessarily change compared with metal exposure from other sources. In one of the few studies testing this metal e-cigarette toxicity, an in vitro study of copper nanoparticles from e-cigarette aerosols, it was found that copper nanoparticles increased mitochondrial oxidative stress and DNA fragmentation, supporting their critical toxic role (Lerner et al., 2016). Metals have also been involved as one possible reason explaining cellular damage, generation of ROS, and activation of global defense systems observed in vitro experiments (Bharadwaj et al., 2017; Lerner et al., 2015a).
Limitations of the current research include the small number of studies, the small sample size, the evaluation mostly of first-generation devices, the limited investigation on which characteristics of the device and patterns of use (e.g., wattage, temperature) could be major contributors of exposure, and the evaluation of devices that are selected by the investigators but which do not necessarily reflect what the consumers are using. Only one study has measured metal biomarkers in e-cigarette users, comparing chromium and nickel concentrations in e-liquid and e-cigarettes aerosols obtained from the participant's devices (mostly tank-style and mod devices) to the corresponding metal biomarker (Aherrera et al., 2017).
Synthesis
An increasing but still limited number of studies have detected metals in e-liquid and aerosol samples generated by e-cigarette devices. Some of the key metals include chromium, nickel, lead, manganese, aluminum, tin, and iron. The coils and other parts of the device could be a source of metals, which could be leaking to the aerosol. Cadmium, which is a metal typically found in e-cigarettes, is found at a markedly lower level than in combustible tobacco cigarettes. However, the number of metals appears to be large, even larger than for combustible tobacco cigarettes. There is also substantial variability in metal levels, which can be substantially high in some instances. Overall the number of studies is small and the relevance for tank-style and mod devices is limited, as most studies have assessed first- and second-generation devices. One biomarker study evaluating e-cigarette devices actually used by the users supports that metals can be inhaled, contributing to metal internal dose, at least for chromium and nickel. While it is well established that metals are highly toxic for multiple organs and systems through inhalation, no studies have evaluated the specific health effects of metals in e-cigarettes, except in the study of copper nanoparticles from e-cigarettes and mitochondrial oxidative stress and DNA fragmentation.
Conclusion 5-4. There is substantial evidence that e-cigarette aerosol contains metals. The origin of the metals could be the metallic coil used to heat the e-liquid, other parts of the e-cigarette device, or e-liquids. Product characteristics and use patterns may contribute to differences in the actual metals and metal concentrations measured in e-cigarette aerosol.
Conclusion 5-5. There is limited evidence that the number of metals in e-cigarette aerosol could be greater than the number of metals in combustible tobacco cigarettes, except for cadmium, which is markedly lower in e-cigarettes compared with combustible tobacco cigarettes.
REFERENCES
- Aberer W, Fuchs T, Peters KP, Frosch PJ. Propylene glycol: Cutaneous side effects and test methods. Literature and results of a multicenter study of the German contact allergy group (DKG). Dermatosen in Beruf und Unwelt. 1993;41:25–27.
- AEMSA (American E-Liquid Manufacturing Standards Association). E-liquid manufacturing standards. 2014. [October 9, 2017]. http://www
.aemsa.org /wp-content/uploads/2014 /02/AEMSA-Standards_Version-1-8.pdf. - Aherrera A, Olmedo P, Grau-Perez M, Tanda S, Goessler W, Jarmul S, Chen R, Cohen JE, Rule AM, Navas-Acien A. The association of e-cigarette use with exposure to nickel and chromium: A preliminary study of non-invasive biomarkers. Environmental Research. 2017;159:313–320. [PubMed: 28837903]
- Ahijevych K, Garrett BE. Menthol pharmacology and its potential impact on cigarette smoking behavior. Nicotine & Tobacco Research. 2004;6(Supplement 1):S17–S28. [February 6, 2018]; https://doi
.org/10.1080 /14622200310001649469. [PubMed: 14982706] - Ahijevych K, Garrett BE. The role of menthol in cigarettes as a reinforcer of smoking behavior. Nicotine & Tobacco Research. 2010;12(Supplement 2):S110–S116. [February 6, 2018]; https://doi
.org/10.1093/ntr/ntq203. [PMC free article: PMC3636955] [PubMed: 21177367] - Allen JG, Flanigan SS, LeBlanc M, Vallarino J, MacNaughton P, Stewart JH, Christiani DC. Flavoring chemicals in e-cigarettes: Diacetyl, 2,3-pentanedione, and acetoin in a sample of 51 products, including fruit-, candy-, and cocktail-flavored e-cigarettes. Environmental Health Perspectives. 2016;124(6):733–739. [PMC free article: PMC4892929] [PubMed: 26642857]
- Arbour R, Esparis B. Osmolar gap metabolic acidosis in a 60-year-old man treated for hypoxemic respiratory failure. Chest. 2000;118(2):545–546. [PubMed: 10936154]
- Arts JH, Muijser H, Appel MJ, Frieke Kuper C, Bessems JG, Woutersen RA. Subacute (28-day) toxicity of furfural in Fischer 344 rats: A comparison of the oral and inhalation route. Food and Chemical Toxicology. 2004;42(9):1389–1399. [PubMed: 15234069]
- Arulanantham K, Genel M. Central nervous system toxicity associated with ingestion of propylene glycol. Journal of Pediatrics. 1978;93(3):515–516. [PubMed: 690781]
- ATSDR (Agency for Toxic Substances and Disease Registry). Acrolein. 2007. [December 22, 2017]. https://www
.atsdr.cdc .gov/toxfaqs/tfacts124.pdf. - Audrain-McGovern J, Strasser AA, Wileyto EP. The impact of flavoring on the rewarding and reinforcing value of e-cigarettes with nicotine among young adult smokers. Drug and Alcohol Dependence. 2016;166:263–267. [PMC free article: PMC4995771] [PubMed: 27426010]
- Barrington-Trimis JL, Urman R, Leventhal AM, Gauderman WJ, Cruz TB, Gilreath TD, Howland S, Unger JB, Berhane K, Samet JM, McConnell R. E-cigarettes, cigarettes, and the prevalence of adolescent tobacco use. Pediatrics. 2016;138(2):e20153983. [February 6, 2018]; http://pediatrics
.aappublications .org/content /138/2/e20153983. [PMC free article: PMC4960723] [PubMed: 27401102] - Beauval N, Howsam M, Anthérieu S, Allorge D, Soyez M, Garçon G, Goossens JF, Lo-Guidice JM, Garat A. Trace elements in e-liquids—Development and validation of an ICP-MS method for the analysis of electronic cigarette refills. Regulatory Toxicology and Pharmacology. 2016;79:144–148. [PubMed: 27058761]
- Behar RZ, Luo WT, Lin SC, Wang YH, Valle J, Pankow JF, Talbot P. Distribution, quantification and toxicity of cinnamaldehyde in electronic cigarette refill fluids and aerosols. Tobacco Control. 2016;25:ii94–ii102. [February 6, 2018]; http://dx
.doi.org/10 .1136/tobacco-control-2016-053224. [PMC free article: PMC5503843] [PubMed: 27633763] - Bekki K, Uchiyama S, Ohta K, Inaba Y, Nakagome H, Kunugita N. Carbonyl compounds generated from electronic cigarettes. International Journal of Environmental Research and Public Health. 2014;11(11):11192–11200. [PMC free article: PMC4245608] [PubMed: 25353061]
- Berg CJ. Preferred flavors and reasons for e-cigarette use and discontinued use among never, current, and former smokers. International Journal of Public Health. 2016;61(2):225–236. [PMC free article: PMC4808473] [PubMed: 26582009]
- Bharadwaj S, Mitchell RJ, Qureshi A, Niazi JH. Toxicity evaluation of e-juice and its soluble aerosols generated by electronic cigarettes using recombinant bioluminescent bacteria responsive to specific cellular damages. Biosensors and Bioelectronics. 2017;90:53–60. [PubMed: 27875752]
- Blair SL, Epstein SA, Nizkorodov SA, Staimer N. A real-time fast-flow tube study of VOC and particulate emissions from electronic, potentially reduced-harm, conventional, and reference cigarettes. Aerosol Science and Technology. 2015;49(9):816–827. [PMC free article: PMC4696598] [PubMed: 26726281]
- Bornmann G. Grundwirkungen der glykile und ihre bedeutung für die toxizatät. Arzneimittelforschung. 1954;4:643–646. [PubMed: 13229791]
- Bortz WM, Paul P, Haff AC, Holmes WL. Glycerol turnover and oxidation in man. Journal of Clinical Investigation. 1972;51(6):1537–1546. [PMC free article: PMC292291] [PubMed: 5024045]
- Braun HA, Cartland GF. The toxicity of propylene glycol. Journal of the American Pharmacists Association. 1936;25(9):746–749.
- Burstyn I. Peering through the mist: Systematic review of what the chemistry of contaminants in electronic cigarettes tells us about health risks. BMC Public Health. 2014;14(18) [February 6, 2018]; https://doi
.org/10.1186/1471-2458-14-18. [PMC free article: PMC3937158] [PubMed: 24406205] - Canistro D, Vivarelli F, Cirillo S, Babot Marquillas C, Buschini A, Lazzaretti M, Marchi L, Cardenia V, Rodriguez-Estrada MT, Lodovici M, Cipriani C, Lorenzini A, Croco E, Marchionni S, Franchi P, Lucarini M, Longo V, Della Croce CM, Vornoli A, Colacci A, Vaccari M, Sapone A, Paolini M. E-cigarettes induce toxicological effects that can raise the cancer risk. Scientific Reports. 2017;7(1):2028. [PMC free article: PMC5435699] [PubMed: 28515485]
- Carmines EL, Gaworski CL. Toxicological evaluation of glycerin as a cigarette ingredient. Food and Chemical Toxicology. 2005;43(10):1521–1539. [PubMed: 15967561]
- Catanzaro JM, Smith JG Jr. Propylene glycol dermatitis. Journal of the American Academy of Dermatology. 1991;24(1):90–95. [PubMed: 1999538]
- Cheng TR. Chemical evaluation of electronic cigarettes. Tobacco Control. 2014;23:11–17. [PMC free article: PMC3995255] [PubMed: 24732157]
- Choi H, Schmidbauer N, Spengler J, Bornehag CG. Sources of propylene glycol and glycol ethers in air at home. International Journal of Environmental Research and Public Health. 2010;7(12):4213–4237. [PMC free article: PMC3037050] [PubMed: 21318004]
- CIR (Cosmetic Ingredient Review). Safety assessment of glycerin as used in cosmetics: Draft report for panel review. 2014. [January 2, 2018]. https://www
.cir-safety .org/sites/default/files/glycerin.pdf. - CIR. Safety assessment of glycerin as used in cosmetics: Final report. 2015. [September 29, 2017]. http://www
.cir-safety .org/sites/default/files /glycer_122014_FR.pdf. - Clark CR, Marshall TC, Merickel BS, Sanchez A, Brownstein DG, Hobbs CH. Toxicological assessment of heat transfer fluids proposed for use in solar energy applications. Toxicology and Applied Pharmacology. 1979;51(3):529–535. [PubMed: 538763]
- Clayton GE, Clayton FE, editors. Patty's industrial hygiene and toxicology. Volume 2E: Toxicology. 4th ed. New York: Wiley; 1995.
- Czoli CD, Goniewicz M, Islam T, Kotnowski K, Hammond D. Consumer preferences for electronic cigarettes: Results from a discrete choice experiment. Tobacco Control. 2016;25(e1):e30–e36. [February 6, 2018]; http:
//tobaccocontrol .bmj.com/content/25/e1/e30. [PubMed: 26490845] - Domej W, Oettl K, Renner W. Oxidative stress and free radicals in COPD—Implications and relevance for treatment. International Journal of Chronic Obstructive Pulmonary Disease. 2014;9:1207–1224. [PMC free article: PMC4207545] [PubMed: 25378921]
- Doolittle DJ, Lee DA, Lee CK. The genotoxic activity of glycerol in an in vitro test battery. Food and Chemical Toxicology. 1988;26(7):631–635. [PubMed: 3053367]
- Etter JF. Throat hit in users of the electronic cigarette: An exploratory study. Psychology of Addictive Behaviors. 2016;30(1):93–100. [PubMed: 26653150]
- Etter JF, Zather E, Svensson S. Analysis of refill liquids for electronic cigarettes. Addiction. 2013;108(9):1671–1679. [PubMed: 23701634]
- Farley SM, Seoh H, Sacks R, Johns M. Teen use of flavored tobacco products in New York City. Nicotine & Tobacco Research. 2014;16(11):1518–1521. [PubMed: 25082831]
- Farsalinos KE, Romagna G, Tsiapras D, Kyrzopoulos S, Spyrou A, Voudris V. Impact of flavour variability on electronic cigarette use experience: An Internet survey. International Journal of Environmental Research and Public Health. 2013;10(12):7272–7282. [PMC free article: PMC3881166] [PubMed: 24351746]
- Farsalinos EK, Gillman G, Poulas K, Voudris V. Tobacco-specific nitrosamines in electronic cigarettes: Comparison between liquid and aerosol levels. International Journal of Environmental Research and Public Health. 2015a;12(8):9046–9053. [PMC free article: PMC4555263] [PubMed: 26264016]
- Farsalinos KE, Gillman IG, Melvin MS, Paolantonio AR, Gardow WJ, Humphries KE, Brown SE, Poulas K, Voudris V. Nicotine levels and presence of selected tobacco-derived toxins in tobacco flavoured electronic cigarette refill liquids. International Journal of Environmental Research and Public Health. 2015b;12(4):3439–3452. [PMC free article: PMC4410195] [PubMed: 25811768]
- Farsalinos KE, Kistler KA, Gillman G, Voudris V. Evaluation of electronic cigarette liquids and aerosol for the presence of selected inhalation toxins. Nicotine & Tobacco Research. 2015c;17(2):168–174. [PMC free article: PMC4892705] [PubMed: 25180080]
- FDA (Food and Drug Administration). FDA briefing document: NDA 21-888 Zimulti (rimonabant) tablets, 20 mg. 2007. [October 9, 2017]. https://www
.fda.gov/ohrms /dockets/ac/07/briefing /2007-4306b1-fda-backgrounder .pdf. - FDRL (Food and Drug Research Laboratories, Inc.). Teratologic evaluation of FDA 71-56 (propylene glycol) in mice, rats, hamsters and rabbits. Waverly, NY: FDRL; 1973.
- Feirman SP, Lock D, Cohen JE, Holtgrave DR, Li TJ. Flavored tobacco products in the United States: A systematic review assessing use and attitudes. Nicotine & Tobacco Research. 2016;18(5):739–749. [PubMed: 26315475]
- FEMA (Flavor and Extract Manufacturers Association of the United States). The safety assessment and regulatory authority to use flavors—Focus on e-cigarettes. 2015. [October 4, 2017]. https://www
.femaflavor .org/sites/default /files/FEMAGRAS_Ecig_March_3_2015.pdf. - Fligner CL, Jack R, Twiggs GA, Raisys VA. Hyperosmolality induced by propylene glycol. A complication of silver sulfadiazine therapy. Journal of the American Medical Association. 1985;253(11):1606–1609. [PubMed: 3974044]
- Flora JW, Meruva N, Huang CB, Wilkinson CT, Ballentine R, Smith DC, Werley MS, McKinney WJ. Characterization of potential impurities and degradation products in electronic cigarette formulations and aerosols. Regulatory Toxicology and Pharmacology. 2016;74:1–11. [PubMed: 26617410]
- Flora JW, Wilkinson CT, Wilkinson JW, Lipowicz PJ, Skapars JA, Anderson A, Miller JH. Method for the determination of carbonyl compounds in e-cigarette aerosols. Journal of Chromatographic Science. 2017;55(2):142–148. [PMC free article: PMC5253970] [PubMed: 28087758]
- Fransway AF, Zug KA, Belsito DV, Deleo VA, Fowler JF Jr., Maibach HI, Marks JG, Mathias CG, Pratt MD, Rietschel RL, Sasseville D, Storrs FJ, Taylor JS, Warshaw EM, Dekoven J, Zirwas M. North American Contact Dermatitis Group patch test results for 2007-2008. Dermatitis. 2013;24(1):10–21. [PubMed: 23340394]
- Funk JO, Maibach HI. Propylene glycol dermatitis: Re-evaluation of an old problem. Contact Dermatitis. 1994;31(4):236–241. [PubMed: 7842679]
- Gad SC, Cassidy CD, Aubert N, Spainhour B, Robbe H. Nonclinical vehicle use in studies by multiple routes in multiple species. International Journal of Toxicology. 2006;25(6):499–521. [PubMed: 17132609]
- Garten S, Falkner RV. Role of mentholated cigarettes in increased nicotine dependence and greater risk of tobacco-attributable disease. Preventive Medicine. 2004;38(6):793–798. [PubMed: 15193900]
- Garza A, Vega R, Soto E. Cellular mechanisms of lead neurotoxicity. Medical Science Monitor. 2006;12(3):RA57–RA65. [PubMed: 16501435]
- Geiss O, Bianchi I, Barrero-Moreno J. Correlation of volatile carbonyl yields emitted by e-cigarettes with the temperature of the heating coil and the perceived sensorial quality of the generated vapours. International Journal of Hygiene and Environmental Health. 2016;219(3):268–277. [PubMed: 26847410]
- Gillman IG, Kistler KA, Stewart EW, Paolantonio AR. Effect of variable power levels on the yield of total aerosol mass and formation of aldehydes in e-cigarette aerosols. Regulatory Toxicology and Pharmacology. 2016;75:58–65. [PubMed: 26743740]
- Goel R, Durand E, Trushin N, Prokopczyk B, Foulds J, Elias RJ, Richie JP Jr. Highly reactive free radicals in electronic cigarette aerosols. Chemical Research in Toxicology. 2015;28(9):1675–1677. [PMC free article: PMC4961046] [PubMed: 26244921]
- Gomes R, Liteplo R, Meek ME. Concise International Chemical Assessment Document #45: Ethylene glycol: Human health aspects. Geneva, Switzerland: World Health Organization; 2002.
- Goniewicz ML, Knysak J, Gawron M, Kosmider L, Sobczak A, Kurek J, Prokopowicz A, Jablonska-Czapla M, Rosik-Dulewska C, Havel C, Jacob IP, Benowitz N. Levels of selected carcinogens and toxicants in vapour from electronic cigarettes. Tobacco Control. 2014;23(2):133–139. [PMC free article: PMC4154473] [PubMed: 23467656]
- Gorrod JW, Jacob P. Analytical determination of nicotine and related compounds and their metabolites. 1st ed. Amsterdam, The Netherlands, and New York: Elsevier; 1999.
- Goyer N, Lavoie J. Emissions of chemical compounds and bioaerosols during the secondary treatment of paper mill effluents. American Industrial Hygiene Association Journal. 2001;62(3):330–341. [PubMed: 11434439]
- Guthery W. Emissions of toxic carbonyls in an electronic cigarette. Beitrage zur Tabakforschung International/Contributions to Tobacco Research. 2016;27(1):30–37.
- Hadwiger ME, Trehy ML, Ye W, Moore T, Allgire J, Westenberger B. Identification of amino-tadalafil and rimonabant in electronic cigarette products using high pressure liquid chromatography with diode array and tandem mass spectrometric detection. Journal of Chromatography A. 2010;1217(48):7547–7555. [PubMed: 20980012]
- Hahn J, Monakhova YB, Hengen J, Kohl-Himmelseher M, Schössler J, Hahn H, Kuballa T, Lachenmeier DW. Electronic cigarettes: Overview of chemical composition and exposure estimation. Tobacco Induced Diseases. 2014;12(1):23. [PMC free article: PMC4304610] [PubMed: 25620905]
- Hansen L, Lange R, Gupta S. Development and evaluation of a guideline for monitoring propylene glycol toxicity in pediatric intensive care unit patients receiving continuous infusion lorazepam. Journal of Pediatric Pharmacology and Therapeutics. 2015;20(5):367–372. [PMC free article: PMC4596122] [PubMed: 26472950]
- Haworth S, Lawlor T, Mortelmans K, Speck W, Zeiger E. Salmonella mutagenicity test results for 250 chemicals. Environmental Mutagenesis. 1983;5(Supplement 1):1–142. [February 6, 2018]; https://doi
.org/10.1002/em.2860050703. [PubMed: 6365529] - Health Council of the Netherlands. Propylene glycol (1,2-propanediol): Health-based recommended occupational exposure limit. 2007. [October 3, 2017]. https://www
.gezondheidsraad .nl/sites/default /files/200702osh_propylene_glycol .pdf. - Hecht SS. Biochemistry, biology, and carcinogenicity of tobacco-specific N-nitrosamines. Chemical Research in Toxicology. 1998;11(6):559–603. [PubMed: 9625726]
- Hecht SS, Hoffmann D. Tobacco-specific nitrosamines, an important group of carcinogens in tobacco and tobacco smoke. Carcinogenesis. 1988;9(6):875–884. [PubMed: 3286030]
- Herrington JS, Myers C. Electronic cigarette solutions and resultant aerosol profiles. Journal of Chromatography A. 2015;1418:192–199. [PubMed: 26422308]
- Hess CA, Olmedo P, Navas-Acien A, Goessler W, Cohen JE, Rule AM. E-cigarettes as a source of toxic and potentially carcinogenic metals. Environmental Research. 2017;152:221–225. [PMC free article: PMC5135636] [PubMed: 27810679]
- HHS (U.S. Department of Health and Human Services). How tobacco smoke causes disease: The biology and behavioral basis for smoking-attributable disease: A report of the Surgeon General. Atlanta, GA: U.S. Department of Health and Human Services, Centers for Disease Control and Prevention, National Center for Chronic Disease Prevention and Health Promotion, Office on Smoking and Health; 2010. [PubMed: 21452462]
- HHS. Select committee on GRAS substances (SCOGS) opinion: Propylene glycol and propylene glycol monostearate. 2015. [September 28, 2017]. https://www
.fda.gov/food /ingredientspackaginglabeling /gras/scogs/ucm261045.htm. - Hutzler C, Paschke M, Kruschinski S, Henkler F, Hahn J, Luch A. Chemical hazards present in liquids and vapors of electronic cigarettes. Archives of Toxicology. 2014;88(7):1295–1308. [PubMed: 24958024]
- IARC (International Agency for Research on Cancer). IARC monographs on the evaluation of carcinogenic risks to humans, Volume 77: Some industrial chemicals. 2000. [October 19, 2017]. http://publications
.iarc .fr/Book-And-Report-Series /Iarc-Monographs-On-The-Evaluation-Of-Carcinogenic-Risks-To-Humans /Some-Industrial-Chemicals-2000. - IARC. Chromium(VI) compounds. 2012. [September 29, 2017]. https://monographs
.iarc .fr/ENG/Monographs /vol100C/mono100C-9.pdf. - IARC. Nickel and nickel compounds. 2017. [September 29, 2017]. http://monographs
.iarc .fr/ENG/Monographs /vol100C/mono100C-10.pdf. - Irwin R. Toxicology and carcinogenesis: Studies of furfural (CAS no. 98-01-1) in F344/N rats and B6C3F1 mice (gavage studies). Research Triangle Park, NC: National Toxicology Program; 1990. [February 6, 2018]. https://ntp
.niehs.nih .gov/ntp/htdocs/lt_rpts/tr382.pdf. [PubMed: 12692654] - Ishidate M Jr., Sofuni T, Yoshikawa K, Hayashi M, Nohmi T, Sawada M, Matsuoka A. Primary mutagenicity screening of food additives currently used in Japan. Food and Chemical Toxicology. 1984;22(8):623–636. [PubMed: 6381265]
- Jaishankar M, Tseten T, Anbalagan N, Mathew BB, Beeregowda KN. Toxicity, mechanism and health effects of some heavy metals. Interdisciplinary Toxicology. 2014;7(2):60–72. [PMC free article: PMC4427717] [PubMed: 26109881]
- Jensen RP, Luo W, Pankow JF, Strongin RM, Peyton DH. Hidden formaldehyde in e-cigarette aerosols. New England Journal of Medicine. 2015;372(4):392–394. [PubMed: 25607446]
- Kavlock RJ, Short RD Jr., Chernoff N. Further evaluation of an in vivo teratology screen. Teratogenesis, Carcinogenesis, and Mutagenesis. 1987;7(1):7–16. [PubMed: 2884745]
- Kehrer JP, Klotz LO. Free radicals and related reactive species as mediators of tissue injury and disease: Implications for health. Critical Reviews in Toxicology. 2015;45(9):765–798. [PubMed: 26610815]
- Kelner MJ, Bailey DN. Propylene glycol as a cause of lactic acidosis. Journal of Analytical Toxicology. 1985;9(1):40–42. [PubMed: 3981973]
- Khlystov A, Samburova V. Flavoring compounds dominate toxic aldehyde production during e-cigarette vaping. Environmental Science & Technology. 2016;50(23):13080–13085. [PubMed: 27934275]
- Kienhuis AS, Soeteman-Hernandez LG, Bos PM, Cremers HW, Klerx WN, Talhout R. Potential harmful health effects of inhaling nicotine-free shisha-pen vapor: A chemical risk assessment of the main components propylene glycol and glycerol. Tobacco Induced Diseases. 2015;13(1):15. [March 1, 2018]; https://doi
.org/10.1186 /s12971-015-0038-7. [PMC free article: PMC4482188] [PubMed: 26120296] - Kim HJ, Shin HS. Determination of tobacco-specific nitrosamines in replacement liquids of electronic cigarettes by liquid chromatography-tandem mass spectrometry. Journal of Chromatography A. 2013;1291:48–55. [PubMed: 23602640]
- King BA, Tynan MA, Dube SR, Arrazola R. Flavored-little-cigar and flavored-cigarette use among U.S. middle and high school students. Journal of Adolescent Health. 2014;54(1):40–46. [PMC free article: PMC4572463] [PubMed: 24161587]
- Kirkham P, Rahman I. Oxidative stress in asthma and COPD: Antioxidants as a therapeutic strategy. Pharmacology & Therapeutics. 2006;111(2):476–494. [PubMed: 16458359]
- Konradova V, Vavrova V, Janota J. Effect of the inhalation of a surface tension-reducing substance (propylene glycol) on the ultrastructure of epithelium of the respiratory passages in rabbits. Folia Morphologica (Praha). 1978;26(1):28–34. [PubMed: 631649]
- Kosmider L, Sobczak A, Fik M, Knysak J, Zaciera M, Kurek J, Goniewicz ML. Carbonyl compounds in electronic cigarette vapors: Effects of nicotine solvent and battery output voltage. Nicotine & Tobacco Research. 2014;16(10):1319–1326. [PMC free article: PMC4838028] [PubMed: 24832759]
- Kosmider L, Sobczak A, Prokopowicz A, Kurek J, Zaciera M, Knysak J, Smith D, Goniewicz ML. Cherry-flavoured electronic cigarettes expose users to the inhalation irritant, benzaldehyde. Thorax. 2016;71(4):376–377. [PMC free article: PMC4937616] [PubMed: 26822067]
- Kowitt SD, Meernik C, Baker HM, Osman A, Huang LL, Goldstein AO. Perceptions and experiences with flavored non-menthol tobacco products: A systematic review of qualitative studies. International Journal of Environmental Research and Public Health. 2017;14(4):338. [PMC free article: PMC5409539] [PubMed: 28333107]
- Kreiss K, Gomaa A, Kullman G, Fedan K, Simoes EJ, Enright PL. Clinical bronchiolitis obliterans in workers at a microwave-popcorn plant. New England Journal of Medicine. 2002;347(5):330–338. [PubMed: 12151470]
- Kubica P, Wasik A, Kot-Wasik A, Namiesnik J. An evaluation of sucrose as a possible contaminant in e-liquids for electronic cigarettes by hydrophilic interaction liquid chromatography-tandem mass spectrometry. Analytical and Bioanalytical Chemistry. 2014;406(13):3013–3018. [PMC free article: PMC3992225] [PubMed: 24664404]
- Kucharska M, Wesołowski W, Czerczak S, Soćko R. Testing of the composition of e-cigarette liquids—Manufacturer-declared vs. true contents in a selected series of products. Medycyna Pracy. 2016;67(2):239–253. [PubMed: 27221300]
- LaKind JS, McKenna EA, Hubner RP, Tardiff RG. A review of the comparative mammalian toxicity of ethylene glycol and propylene glycol. Critical Reviews in Toxicolology. 1999;29(4):331–365. [PubMed: 10451263]
- Latven AR, Molitor H. Comparison of the toxic, hypnotic and irritating properties of eight organic solvents. Journal of Pharmacology and Experimental Therapeutics. 1939;65(1):89.
- Laug EP, Calvery HO, Morris HJ, Woodard G. The toxicology of some glycols and derivatives. Journal of Industrial Hygiene and Toxicology. 1939;21(6):173–201.
- Lerner CA, Sundar IK, Watson RM, Elder A, Jones R, Done D, Kurtzman R, Ossip DJ, Robinson R, McIntosh S, Rahman I. Environmental health hazards of e-cigarettes and their components: Oxidants and copper in e-cigarette aerosols. Environmental Pollution. 2015a;198:100–107. [PMC free article: PMC4323666] [PubMed: 25577651]
- Lerner CA, Sundar IK, Yao H, Gerloff J, Ossip DJ, McIntosh S, Robinson R, Rahman I. Vapors produced by electronic cigarettes and e-juices with flavorings induce toxicity, oxidative stress, and inflammatory response in lung epithelial cells and in mouse lung. PLoS ONE. 2015b;10(2):e0116732. [February 6, 2018]; https://doi
.org/10.1371/journal .pone.0116732. [PMC free article: PMC4319729] [PubMed: 25658421] - Lerner CA, Rutagarama P, Ahmad T, Sundar IK, Elder A, Rahman I. Electronic cigarette aerosols and copper nanoparticles induce mitochondrial stress and promote DNA fragmentation in lung fibroblasts. Biochemical and Biophysical Research Communications. 2016;477(4):620–625. [PMC free article: PMC4967027] [PubMed: 27343559]
- Lessmann H, Schnuch A, Geier J, Uter W. Skin-sensitizing and irritant properties of propylene glycol. Contact Dermatitis. 2005;53(5):247–259. [PubMed: 16283903]
- Li QD, Zhan YC, Wang L, Leischow SJ, Zeng DD. Analysis of symptoms and their potential associations with e-liquids' components: A social media study. BMC Public Health. 2016;16(674) [February 6, 2018]; https://doi
.org/10.1186 /s12889-016-3326-0. [PMC free article: PMC4967297] [PubMed: 27475060] - Lim HH, Shin HS. Determination of volatile organic compounds including alcohols in refill fluids and cartridges of electronic cigarettes by headspace solid-phase micro extraction and gas chromatography-mass spectrometry. Analytical and Bioanalytical Chemistry. 2017;409(5):1247–1256. [PubMed: 27826631]
- Lim TY, Poole RL, Pageler NM. Propylene glycol toxicity in children. Journal of Pediatric Pharmacology and Therapeutics. 2014;19(4):277–282. [PMC free article: PMC4341412] [PubMed: 25762872]
- Lin EC. Glycerol utilization and its regulation in mammals. Annual Review of Biochemistry. 1977;46:765–795. [PubMed: 197882]
- Lisko JG, Tran H, Stanfill SB, Blount BC, Watson CH. Chemical composition and evaluation of nicotine, tobacco alkaloids, pH, and selected flavors in e-cigarette cartridges and refill solutions. Nicotine & Tobacco Research. 2015;17(10):1270–1278. [PMC free article: PMC4573955] [PubMed: 25636907]
- Litt MD, Duffy V, Oncken C. Cigarette smoking and electronic cigarette vaping patterns as a function of e-cigarette flavourings. Tobacco Control. 2016;25:ii67–ii72. [February 6, 2018]; http:
//tobaccocontrol .bmj.com/content/25/Suppl_2/ii67. [PMC free article: PMC5479061] [PubMed: 27633766] - MacCannell KL. Hemodynamic responses to glycols and to hemolysis. Canadian Journal of Physiology and Pharmacology. 1969;47(6):563–569. [PubMed: 5797397]
- MAK Commission. The MAK collection for occupational health and safety. Weinheim, Germany: Wiley-VCH Verlag GmbH & Co. KGaA; 2002. Benzaldehyde [MAK value documentation, 2002] pp. 14–36.
- Maridet C, Atge B, Amici JM, Taieb A, Milpied B. The electronic cigarette: The new source of nickel contact allergy of the 21st century? Contact Dermatitis. 2015;73(1):49–50. [PubMed: 25801540]
- Martin G, Finberg L. Propylene glycol: A potentially toxic vehicle in liquid dosage form. Journal of Pediatrics. 1970;77(5):877–888. [PubMed: 5504082]
- Martinez RE, Dhawan S, Sumner W, Williams B. On-line chemical composition analysis of refillable electronic cigarette aerosol—Measurement of nicotine and nicotyrine. Nicotine & Tobacco Research. 2014;17(10):1263–1269. [PubMed: 25542921]
- McCauley L, Markin C, Hosmer D. An unexpected consequence of electronic cigarette use. Chest. 2012;141(4):1110–1113. [PubMed: 22474155]
- Messner B, Bernhard D. Smoking and cardiovascular disease: Mechanisms of endothelial dysfunction and early atherogenesis. Arteriosclerosis, Thrombosis, and Vascular Biology. 2014;34(3):509–515. [PubMed: 24554606]
- Mikheev VB, Brinkman MC, Granville CA, Gordon SM, Clark PI. Real-time measurement of electronic cigarette aerosol size distribution and metals content analysis. Nicotine & Tobacco Research. 2016;18(9):1895–1902. [PMC free article: PMC4978987] [PubMed: 27146638]
- Moline JM, Golden AL, Highland JH, Wilmarth KR, Kao AS. Health effects evaluation of theatrical smoke, haze, and pyrotechnics. 2000. [September 28, 2017]. http://www
.actorsequity .org/docs/safesan/finalreport.pdf. - Morris HJ, Nelson AA, Calvery HO. Observations on the chronic toxicities of propylene glycol, ethylene glycol, diethylene glycol, ethylene glycol mono-ethyl-ether, and diethylene glycol mono-ethyl-ether. Journal of Pharmacology and Experimental Therapeutics. 1942;74(3):266–273.
- Morrissey RE, Lamb JC 4th, Morris RW, Chapin RE, Gulati DK, Heindel JJ. Results and evaluations of 48 continuous breeding reproduction studies conducted in mice. Fundamental and Applied Toxicology. 1989;13(4):747–777. [PubMed: 2620795]
- Navas-Acien A, Guallar E, Silbergeld EK, Rothenberg SJ. Lead exposure and cardiovascular disease—A systematic review. Environmental Health Perspectives. 2007;115(3):472–482. [PMC free article: PMC1849948] [PubMed: 17431501]
- Nelson JL, Harmon ME, Robergs RA. Identifying plasma glycerol concentration associated with urinary glycerol excretion in trained humans. Journal of Analytical Toxicology. 2011;35(9):617–623. [PubMed: 22080901]
- NIOSH (National Institute for Occupational Safety and Health). Exposures to flavoring chemicals: How and where exposures may occur. 2016. [October 11, 2017]. https://www
.cdc.gov/niosh /topics/flavorings/exposure.html. - Nonnemaker J, Kim AE, Lee YO, MacMonegle A. Quantifying how smokers value attributes of electronic cigarettes. Tobacco Control. 2016;25(e1):e37–e43. [February 6, 2018]; http://dx
.doi.org/10 .1136/tobaccocontrol-2015-052511. [PubMed: 26546152] - NTIS (National Technical Information Service). Teratological evaluation of glycerin in mice, rats and rabbits. Rockville, MD: U.S. Department of Commerce; 1974.
- NTP (National Toxicology Program). Study of glycidol in genetically modified haploinsufficient p16Ink4a/p19Arf mice. 2007. [December 22, 2017]. https://ntp
.niehs.nih .gov/ntp/htdocs/gmm_rpts/gmm13.pdf. [PMC free article: PMC8950432] [PubMed: 18784757] - OECD (Organisation for Economic Co-operation and Development). Dipropylene glycol (mixed isomers and dominant isomer): CAS no: 25265-71-8 & 110-98-5. 2001. [December 22, 2017]. http://www
.inchem.org /documents/sids/sids/25265-71-8.pdf. - OECD. Screening information data set (SIDS): SIDS initial assessment report for SIAM 14; glycerol: CAS no: 56-81-5. 2002. [February 6, 2018]. http://www
.inchem.org /documents/sids/sids/56815.pdf. - OEHHA (Office of Environmental Health Hazard Assessment). Proposition 65: No significant risk levels (NSRLs) for carcinogens and maximum allowable dose levels (MADLs) for chemicals causing reproductive toxicity. 2013. [August 18, 2017]. http://oehha
.ca.gov/media /downloads/proposition-65 /chemicals/safeharbor081513_0 .pdf. - Oh JA, Shin HS. Identification and quantification of several contaminated compounds in replacement liquids of electronic cigarettes by gas chromatography-mass spectrometry. Journal of Chromatography B. 2015;53(6):841–848. [PubMed: 25404560]
- Ohta K, Uchiyama S, Inaba Y, Nakagome H, Kunugita N. Determination of carbonyl compounds generated from the electronic cigarette using coupled silica cartridges impregnated with hydroquinone and 2,4-dinitrophenylhydrazine. Bunseki Kagaku. 2011;60(10):791–797.
- Olmedo P, Goessler W, Tanda S, Grau-Perez M, Jarmul S, Aherrera A, Chen R, Hilpert M, Cohen JE, Navas-Acien A, Rule AM. Metal concentrations in e-cigarette liquid and aerosol samples: The contribution of metallic coils. Environmental Health Perspectives. 2018;126(2):027010. [March 1, 2018]; https://doi
.org/10.1289/EHP2175. [PMC free article: PMC6066345] [PubMed: 29467105] - Ormerod E, Stone N. Contact allergy and electronic cigarettes (and eyelash curlers). Clinical and Experimental Dermatology. 2017;42(6):682–683. [PubMed: 28543750]
- OSHA (Occupational Safety and Health Administration). Glycerin mist (total dust). 2006. [September 7, 2017]. https://www
.osha.gov /dts/chemicalsampling/data/CH_243600 .html. - Ostwald R. Glycerol intake, blood cholesterol level and anemia in the guinea pig and rabbit. Proceedings of the Society for Experimental Biology and Medicine. 1962;111:632–634. [PubMed: 13940619]
- Papousek R, Pataj Z, Novakova P, Lemr K, Bartak P. Determination of acrylamide and acrolein in smoke from tobacco and e-cigarettes. Chromatographia. 2014;77(17-18):1145–1151.
- Phaniendra A, Jestadi DB, Periyasamy L. Free radicals: Properties, sources, targets, and their implication in various diseases. Indian Journal of Clinical Biochemistry. 2015;30(1):11–26. [PMC free article: PMC4310837] [PubMed: 25646037]
- Prescott C, Bottle SE. Biological relevance of free radicals and nitroxides. Cell Biochemistry and Biophysics. 2017;75(2):227–240. [PubMed: 27709467]
- Pryor WA. Cigarette smoke radicals and the role of free radicals in chemical carcinogenicity. Environmental Health Perspectives. 1997;105(Supplement 4):875–882. [PMC free article: PMC1470037] [PubMed: 9255574]
- Regueiro J, Giri A, Wenzl T. Optimization of a differential ion mobility spectrometry-tandem mass spectrometry method for high-throughput analysis of nicotine and related compounds: Application to electronic cigarette refill liquids. Analytical Chemistry. 2016;88(12):6500–6508. [PubMed: 27173877]
- Renne RA, Wehner AP, Greenspan BJ, Deford HS, Ragan HA, Westerberg RB, Buschbom RL, Burger GT, Hayes AW, Suber RL, Mosberg AT. 2-Week and 13-week inhalation studies of aerosolized glycerol in rats. Inhalation Toxicology. 1992;4(2):95–111.
- Robertson OH, Loosli CG, Puck TT, Wise H, Lemon HM, Lester W. Tests for the chronic toxicity of propylene glycol and triethylene glycol on monkeys and rats by vapor inhalation and oral administration. Journal of Pharmacology and Experimental Therapeutics. 1947;91(1):52–76. [PubMed: 20265820]
- Saffari A, Daher N, Ruprecht A, De Marco C, Pozzi P, Boffi R, Hamad SH, Shafer MM, Schauer JJ, Westerdahl D, Sioutas C. Particulate metals and organic compounds from electronic and tobacco-containing cigarettes: Comparison of emission rates and secondhand exposure. Environmental Sciences: Processes and Impacts. 2014;16(10):2259–2267. [PubMed: 25180481]
- Sleiman M, Logue JM, Montesinos VN, Russell ML, Litter MI, Gundel LA, Destaillats H. Emissions from electronic cigarettes: Key parameters affecting the release of harmful chemicals. Environmental Science & Technology. 2016;50(17):9644–9651. [PubMed: 27461870]
- Smyth HF Jr., Seaton J, Fischer L. The single dose toxicity of some glycols and derivatives. Journal of Industrial Hygiene and Toxicology. 1941;23:259–268.
- Soule EK, Nasim A, Rosas S. Adverse effects of electronic cigarette use: A concept mapping approach. Nicotine & Tobacco Research. 2016;18(5):678–685. [PMC free article: PMC5896831] [PubMed: 26563262]
- Soussy S, El-Hellani A, Baalbaki R, Salman R, Shihadeh A, Saliba NA. Detection of 5-hydroxymethylfurfural and furfural in the aerosol of electronic cigarettes. Tobacco Control. 2016;25(Supplement 2):ii88–ii93. [February 6, 2018]; http:
//tobaccocontrol .bmj.com/content/25/Suppl_2/ii88.long. [PubMed: 27798321] - Speth PA, Vree TB, Neilen NF, de Mulder PH, Newell DR, Gore ME, de Pauw BE. Propylene glycol pharmacokinetics and effects after intravenous infusion in humans. Therapeutic Drug Monitoring. 1987;9(3):255–258. [PubMed: 3672566]
- Stålhandske T, Slanina P. Nicotyrine inhibits in vivo metabolism of nicotine without increasing its toxicity. Toxicology and Applied Pharmacology. 1982;65(3):366–372. [PubMed: 7157369]
- Stolzenberg SJ, Hine CH. Mutagenicity of halogenated and oxygenated three-carbon compounds. Journal of Toxicology and Environmental Health. 1979;5(6):1149–1158. [PubMed: 393836]
- Suber RL, Deskin R, Nikiforov I, Fouillet X, Coggins CR. Subchronic nose-only inhalation study of propylene glycol in Sprague-Dawley rats. Food & Chemical Toxicology. 1989;27(9):573–583. [PubMed: 2807102]
- Surh YJ, Tannenbaum SR. Activation of the Maillard reaction product 5-(hydroxymethyl)furfural to strong mutagens via allylic sulfonation and chlorination. Chemical Research in Toxicology. 1994;7(3):313–318. [PubMed: 8075362]
- Surh YJ, Liem A, Miller JA, Tannenbaum SR. 5-sulfooxymethylfurfural as a possible ultimate mutagenic and carcinogenic metabolite of the Maillard reaction product, 5-hydroxymethylfurfural. Carcinogenesis. 1994;15(10):2375–2377. [PubMed: 7955080]
- Sussan TE, Gajghate S, Thimmulappa RK, Ma J, Kim JH, Sudini K, Consolini N, Cormier SA, Lomnicki S, Hasan F, Pekosz A, Biswal S. Exposure to electronic cigarettes impairs pulmonary anti-bacterial and anti-viral defenses in a mouse model. PLoS ONE. 2015;10(2):e0116861. [February 6, 2018]; https://doi
.org/10.1371/journal .pone.0116861. [PMC free article: PMC4317176] [PubMed: 25651083] - Tayyarah R, Long GA. Comparison of select analytes in aerosol from e-cigarettes with smoke from conventional cigarettes and with ambient air. Regulatory Toxicology and Pharmacology. 2014;70(3):704–710. [PubMed: 25444997]
- Tchounwou PB, Yedjou CG, Patlolla AK, Sutton DJ. Heavy metals toxicity and the environment. EXS. 2012;101:133–164. [PMC free article: PMC4144270] [PubMed: 22945569]
- Tierney PA, Karpinski CD, Brown JE, Luo W, Pankow JF. Flavour chemicals in electronic cigarette fluids. Tobacco Control. 2016;25(e1):e10–e15. [February 6, 2018]; http://dx
.doi.org/10 .1136/tobaccocontrol-2014-052175. [PMC free article: PMC4853541] [PubMed: 25877377] - Tourtellotte WW, Reinglass JL, Newkirk TA. Cerebral dehydration action of glycerol. I. Historical aspects with emphasis on the toxicity and intravenous administration. Clinical Pharmacology & Therapeutics. 1972;13(2):159–171. [PubMed: 4552818]
- TPSAC (Tobacco Products Scientific Advisory Committee). Menthol cigarettes and public health: Review of the scientific evidence and recommendations. Silver Spring, MD: Center for Tobacco Products, Food and Drug Administration; 2011.
- Uchiyama S, Inaba Y, Kunugita N. Determination of acrolein and other carbonyls in cigarette smoke using coupled silica cartridges impregnated with hydroquinone and 2,4-dinitrophenylhydrazine. Journal of Chromatography A. 2010;1217(26):4383–4388. [PubMed: 20483418]
- Uchiyama S, Ohta K, Inaba Y, Kunugita N. Determination of carbonyl compounds generated from the e-cigarette using coupled silica cartridges impregnated with hydroquinone and 2,4-dinitrophenylhydrazine, followed by high-performance liquid chromatography. Analytical Sciences. 2013;29(12):1219–1222. [PubMed: 24334991]
- Uryupin AB, Peregudov AS, Kochetkov KA, Bulatnikova LN, Kiselev SS, Nekrasov YS. Qualitative and quantitative compositions of fluids for electronic cigarettes. Pharmaceutical Chemistry Journal. 2013;46(11):687–692.
- USP (U.S. Pharmacopeia). Nicotine. n.d. [October 9, 2017]. http://www
.pharmacopeia .cn/v29240/usp29nf24s0_m56620.html. - Van Winkle W Jr., Newman HW. Further results of continued administration of propylene glycol. Journal of Food Science. 1941;6(5):509–516.
- Varlet V, Farsalinos K, Augsburger M, Thomas A, Etter JF. Toxicity assessment of refill liquids for electronic cigarettes. International Journal of Environmental Research and Public Health. 2015;12(5):4796–4815. [PMC free article: PMC4454939] [PubMed: 25941845]
- Varughese S, Teschke K, Brauer M, Chow Y, van Netten C, Kennedy SM. Effects of theatrical smokes and fogs on respiratory health in the entertainment industry. American Journal of Industrial Medicine. 2005;47(5):411–418. [PubMed: 15828073]
- Venable JR, McClimans CD, Flake RE, Dimick DB. A fertility study of male employees engaged in the manufacture of glycerine. Journal of Occupational and Environmental Medicine. 1980;22(2):87–91. [PubMed: 7373447]
- Villanti AC, Richardson A, Vallone DM, Rath JM. Flavored tobacco product use among U.S. young adults. American Journal of Preventive Medicine. 2013;44(4):388–391. [PubMed: 23498105]
- Wang L, Zhan YC, Li QD, Zeng DD, Leischow SJ, Okamoto J. An examination of electronic cigarette content on social media: Analysis of e-cigarette flavor content on Reddit. International Journal of Environmental Research and Public Health. 2015;12(11):14916–14935. [PMC free article: PMC4661688] [PubMed: 26610541]
- Wang P, Chen W, Liao J, Matsuo T, Ito K, Fowles J, Shusterman D, Mendell M, Kumagai K. A device-independent evaluation of carbonyl emissions from heated electronic cigarette solvents. PLoS ONE. 2017;12(1):e0169811. [February 6, 2018]; https://doi
.org/10.1371/journal .pone.0169811. [PMC free article: PMC5226727] [PubMed: 28076380] - Warshaw EM, Botto NC, Maibach HI, Fowler JF Jr., Rietschel RL, Zug KA, Belsito DV, Taylor JS, DeLeo VA, Pratt MD, Sasseville D, Storrs FJ, Marks JG Jr., Mathias CG. Positive patch-test reactions to propylene glycol: A retrospective cross-sectional analysis from the North American Contact Dermatitis Group, 1996 to 2006. Dermatitis. 2009;20(1):14–20. [PubMed: 19321115]
- Weatherby JH, Haag HB. Toxicity of propylene glycol. Journal of the American Pharmacists Association. 1938;27(6):466–471.
- Werley MS, McDonald P, Lilly P, Kirkpatrick D, Wallery J, Byron P, Venitz J. Non-clinical safety and pharmacokinetic evaluations of propylene glycol aerosol in Sprague-Dawley rats and beagle dogs. Toxicology. 2011;287(1-3):76–90. [PubMed: 21683116]
- Wiebe JP, Barr KJ. The control of male fertility by 1,2,3-trihydroxypropane (THP; glycerol): Rapid arrest of spermatogenesis without altering libido, accessory organs, gonadal steroidogenesis, and serum testosterone, LH and FSH. Contraception. 1984a;29(3):291–302. [PubMed: 6428807]
- Wiebe JP, Barr KJ. Suppression of spermatogenesis without inhibition of steroidogenesis by a 1,2,3-trihydroxypropane solution. Life Sciences. 1984b;34(18):1747–1754. [PubMed: 6427545]
- Wiebe JP, Barr KJ, Buckingham KD. Sustained azoospermia in squirrel monkey, Saimiri sciureus, resulting from a single intratesticular glycerol injection. Contraception. 1989;39(4):447–457. [PubMed: 2721196]
- Wieslander G, Norback D, Lindgren T. Experimental exposure to propylene glycol mist in aviation emergency training: Acute ocular and respiratory effects. Occupational and Environmental Medicine. 2001;58(10):649–655. [PMC free article: PMC1740047] [PubMed: 11555686]
- Williams M, Villarreal A, Bozhilov K, Lin S, Talbot P. Metal and silicate particles including nanoparticles are present in electronic cigarette cartomizer fluid and aerosol. PLoS ONE. 2013;8(3):e57987. [February 6, 2018]; https://doi
.org/10.1371/journal .pone.0057987. [PMC free article: PMC3603976] [PubMed: 23526962] - Williams M, Bozhilov K, Ghai S, Talbot P. Elements including metals in the atomizer and aerosol of disposable electronic cigarettes and electronic hookahs. PLoS ONE. 2017;12(4):e0175430. [February 6, 2018]; https://doi
.org/10.1371/journal .pone.0175430. [PMC free article: PMC5393578] [PubMed: 28414730] - Yamaguchi T. Mutagenicity of trioses and methyl glyoxal on Salmonella typhimurium. Agricultural and Biological Chemistry. 1982;46(3):849–851.
- Yingst JM, Veldheer S, Hammett E, Hrabovsky S, Foulds J. A method for classifying user-reported electronic cigarette liquid flavors. Nicotine & Tobacco Research. 2017;19(11):1381–1385. [PMC free article: PMC5896500] [PubMed: 28064201]
- Yu DK, Elmquist WF, Sawchuk RJ. Pharmacokinetics of propylene glycol in humans during multiple dosing regimens. Journal of Pharmaceutical Sciences. 1985;74(8):876–879. [PubMed: 4032274]
- Zhu SH, Sun JY, Bonnevie E, Cummins SE, Gamst A, Yin L, Lee M. Four hundred and sixty brands of e-cigarettes and counting: Implications for product regulation. Tobacco Control. 2014;23(Supplement 3):iii3–iii9. [February 6, 2018]; http://dx
.doi.org/10 .1136/tobaccocontrol-2014-051670. [PMC free article: PMC4078673] [PubMed: 24935895]
Footnotes
- 1
See, for example, http://www
.whitecloudelectroniccigarettes .com/blog/vaping-throat-irritation (accessed January 5, 2018); http: //ecigarettereviewed .com/allergies-conditions-and-e-liquid (accessed January 5, 2018); and http://www .ecigarette-politics .com/pgsensitivity.html (accessed January 5, 2018). - 2
See, for example, https://www
.misthub.com /blogs/vape-tutorials /76788613-tutorial-propylene-glycol-pg-vs-vegetable-glycerin-vg-e-juice (accessed January 5, 2018); https://vapingdaily .com /best-vape-juices-and-e-liquids /pg-vs-vg (accessed January 5, 2018). - 3
21 CFR § 182.1320.
- 4
As described in Chapter 3, the particle count in e-cigarette aerosols may not be substantially different than mainstream combustible tobacco smoke. However, whereas e-cigarette aerosol particulate consists largely of aqueous droplets and vapors of humectants, particulate matter in combustible tobacco smoke are complex, largely organic constituents that include known or suspected carcinogens. Thus, it would be incorrect to assume that the long-term health risks of the two aerosols were similar just because particle count was similar.
- Toxicology of E-Cigarette Constituents - Public Health Consequences of E-Cigaret...Toxicology of E-Cigarette Constituents - Public Health Consequences of E-Cigarettes
Your browsing activity is empty.
Activity recording is turned off.
See more...