NCBI Bookshelf. A service of the National Library of Medicine, National Institutes of Health.
IARC Working Group on the Evaluation of Carcinogenic Risks to Humans. Coffee, Tea, Mate, Methylxanthines and Methylglyoxal. Lyon (FR): International Agency for Research on Cancer; 1991. (IARC Monographs on the Evaluation of Carcinogenic Risks to Humans, No. 51.)
1. Chemical and Physical Data
1.1. Synonyms
Chem. Abstr. Services Reg. No.: 58-08-2
Chem. Abstr. Name: 3,7-Dihydro-1,3,7-trimethyl-1H-purine-2,6-dione
Synonyms: Anhydrous caffeine; coffeine; coffeinum; guaranine; methyltheobromine; methyltheophylline; thein; theine; 1,3,7-trimethyl-2,6-dioxopurine; 1,3,7-trimethylxanthine
1.2. Structural and molecular formulae and molecular weight
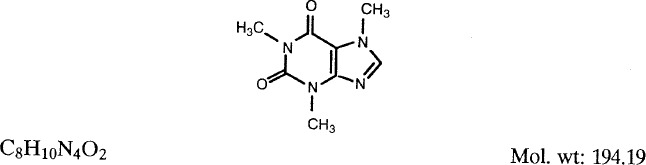
1.3. Chemical and physical properties of the pure substance
- Description: White, odourless powder with a slightly bitter taste (Gennaro, 1985; Macrae, 1985; McElvoy, 1989); glistening white crystals or a white crystalline powder (Moffat, 1986); glistening white needles (National Research Council, 1981; Gennaro, 1985; McElvoy, 1989)
- Sublimation-point: 178°C (Budavari, 1989); about 180°C (Moffat, 1986)
- Melting-point: 234–239°C (Moffat, 1986); 238°C (Budavari, 1989). When crystallized from water, caffeine was thought until recently to contain one molecule of water (monohydrate, CAS No. 5743-12-4); more recent studies indicated that it is in fact a 4/5 hydrate (Macrae, 1985); anhydrous when crystallized from ethanol, chloroform or diethyl ether (Moffat, 1986)
- Density: 1.23 (Budavari, 1989)
- Spectroscopy data: Ultraviolet spectra: aqueous acid; 273 nm ; no alkaline shift; infrared spectra: principal peaks at wave numbers 1658, 1698, 747, 1548, 1242 and 760 nm (potassium bromide disc); mass spectra: principal peaks at m/z 194, 109, 55, 67, 82, 195, 24 and 110 (Moffat, 1986)
- Solubility: Soluble in water (1.0 g/46 ml at 20°C, 1.0 g/5.5 ml at 80°C, 1.0 g/l.5 ml at 100°C (Budavari, 1989); 1.0 g/50 ml water (Gennaro, 1985), ethanol (1.0 g/130 ml; Moffat, 1986; 1.0 g/22 ml at 60°C), acetone (1.0 g/50 ml), chloroform (1.0 g/5.5 ml), diethyl ether (1.0 g/530 ml), benzene (1.0 g/100 ml at 20°C, 1.0 g/22 ml in boiling benzene) and ethyl acetate; slightly soluble in petroleum ether (Budavari, 1989)
- Stability: Decomposed by strong solutions of caustic alkalis (Moffat, 1986); salts decomposed by water
- Equilibrium constants: acidic (Ka), < 1.0×l0−14 at 25°C; basic (Kb), 0.7×10−14 at 19°C (Windholz, 1983)
- Octanol/water partition coefficient (P): log P, 0.0 at pH 7.4 (Moffat, 1986)
1.4. Technical products and impurities
Caffeine (anhydrous or containing one molecule of water of hydration) is available in a USP grade with the following specifications: it contains not less than 98.5% and not more than 101.0% of the above ingredient calculated on an anhydrous basis, not more than 0.1% residue on ignition; 0.5% max weight loss on drying the anhydrous form and not more than 8.5% of its weight when drying the hydrous form (US Pharmacopeial Convention, 1990).
Trade names: Caffeedrine; Dexitac; No Doz (Nodoz); Quick Pep; Tirend; Vivarin (Griffith, 1989; McElvoy, 1989)
2. Production, Use, Occurrence and Analysis
2.1. Production and use
(a) Production
Caffeine is produced commercially by both extraction and synthetic procedures. Extraction procedures involve three methods: direct decaffeination of green coffee beans with solvents, extraction from tea dusts and wastes and fragments of tea leaves, and extraction from cola nuts (McCutheon, 1969; Menthe, 1985; Halsey & Johnston, 1987). Caffeine has been obtained as a by-product from the manufacture of caffeine-free coffee (Budavari, 1989), initially by water and then by solvents, e.g., trichloroethylene (see IARC, 1979a, 1987), dichloromethane (see IARC, 1979b; Anon., 1986; IARC, 1987), ethyl acetate (Anon., 1986, 1987a), water-carbon dioxide processes (Anon., 1987a), and also using oil from spent coffee grounds to remove caffeine from green coffee beans (Anon., 1986). Refining processes are needed to provide the pure caffeine of commerce.
Pressurized carbon dioxide is employed to remove caffeine from tea in the production of decaffeinated tea (Anon., 1986). The extraction yields in the production of natural caffeine have declined significantly in recent years, following the increasing use of water-based as opposed to direct solvent-based extraction procedures.
Synthetic production of caffeine involves the methylation of various xanthines (primarily theobromine) (Halsey & Johnston, 1987) and theophylline (Stanovnik et al., 1982; Nesterov et al., 1985) or the reaction of theophylline with carbon monoxide and methanol (Bott, 1982); total synthesis can be achieved with dimethyl carbamide and malonic acid (Anon., 1987b). Most of the caffeine produced in the USA prior to 1945 was obtained by methylation of theobromine extracted from cocoa; the methylation agents used were dichloromethane and dimethyl sulfate. Contemporary figures for the production of caffeine in the USA could not be obtained; production in 1962 totalled 1 959 000 pounds (889 400 kg), while caffeine imports totalled 1 807 000 pounds (820 400 kg) (Huber, 1964).
The US International Trade Commission did not report domestic production from green coffee beans or of synthetic caffeine for 1986 (US International Trade Commission, 1987). US imports of caffeine in 1988 totalled 6 345 310 pounds (2900 thousand kg), while exports during this period were 753 515 pounds (342.1 thousand kg) (US Bureau of the Census, 1989). US imports of caffeine and its derivatives totalled 6.9 million pounds (3133 thousand kg) in 1987 (Anon., 1988), 5.3 million pounds (2406 thousand kg) in 1986, 5 million pounds (2270 thousand kg) in 1985 (Anon., 1987a) and 6.2 million pounds (2815 thousand kg) in 1980 (Hirsh, 1984). Estimates for the amount of caffeine sold in the USA ranged from 8 to 12 million pounds (3632–5448 thousand kg) in 1986, 80% of which was used in soft drinks (Anon., 1986). Caffeine is produced from green coffee by two companies in the USA (Anon., 1987a) and synthetic caffeine by one (Anon., 1989a). US synthetic production capacity was reported to have been expanded in 1989 by an additional 500 000 pounds (227 000 kg) (Anon., 1989a). It is estimated that 60% of caffeine used in the USA is synthetic (Anon., 1986).
Caffeine is also produced in China, the Federal Republic of Germany, Italy, Japan, the Netherlands and Switzerland (Anon., 1987a, 1988, 1989a; Stabilimento Farmaceutico ‘Cau. G. Testa’, 1989). In the Federal Republic of Germany, approximately 500 tonnes of caffeine are obtained annually from the decaffeination of coffee, while some 3000–3300 tonnes are produced synthetically (Menthe, 1985).
(b) Use
Approximately 80–90% of caffeine extracted from green coffee is used in the beverage industry and most of the remainder and synthetic caffeine are used in pharmaceutical applications (Anon., 1987a, 1988). Caffeine is permitted in the USA in nonalcoholic carbonated cola-type beverages at a content of up to 0.02% by weight of the finished product (Anon., 1987b; US Food and Drug Administration, 1988). It may be used as a flavour enhancer or synergist in foods as served at levels of up to 200 ppm (0.02%) and as a flavouring agent in baked goods, frozen dairy desserts, mixes, gelatins, puddings, fillings and soft candy at levels of up to 400 ppm (Anon., 1987b).
Caffeine is an ingredient in many prescription and nonprescription drugs including stimulant tablets, headache and cold remedies, tablets for the relief of menstrual pain, weight control aids and diuretics (US Food and Drug Administration, 1980). About 1000 prescription drugs and 2000 ‘over-the-counter’ drugs available in the USA contain caffeine (US Food and Drug Administration, 1980; Barone & Roberts, 1984). Caffeine is widely used in a variety of over-the-counter oral drug preparations, often in combination with analgesics such as aspirin, paracetamol (see IARC, 1990), phenacetin (see IARC, 1987) and propoxyphene for the relief of headaches or menstrual tension, with ergotamine tartrate for the treatment of migraine and in combination with some antihistamines to overcome their soporific effects (Gennaro, 1985; Griffith, 1989; McElvoy, 1989; Consumers Union, 1990; US Pharmacopeial Convention, 1990). Caffeine (usually as caffeine citrate) has been used intravenously in the treatment of neonatal apnoea (McElvoy, 1989), to control asthmatic symptoms and to relieve bronchial spasms (Stavric, 1988). Injection of caffeine and sodium benzoate has been used for the symptomatic relief of headache following spinal puncture (McElvoy, 1989). Caffeine has been used in combination with cisplatin (see IARC, 1987) and cytarabine in phase I–II chemotherapy of advanced pancreatic cancer (Dougherty et al., 1989).
Concentrations of caffeine are 100–200 mg/tablet in stimulants (US Food and Drug Administration, 1980; Gennaro, 1985; Huff, 1989a,b), 15–65 mg/tablet in analgesic combinations (US Food and Drug Administration, 1980; Huff, 1989a), 15–33 mg/tablet in cold and allergy relief formulations, 66–200 mg/capsule in weight control aids, 16–200 mg/tablet in diuretics (US Food and Drug Administration, 1980) and 33–65 mg/tablet in menstrual relief products (Huff, 1989a,b). Caffeine levels in over-the-counter drugs vary widely but are typically 15–200 mg/tablet or capsule, depending on both the type of product and the brand (Barone & Roberts, 1984).
2.2. Occurrence
(a) Natural occurrence
Caffeine occurs in more than 60 plant species throughout the world (Barone & Roberts, 1984; Gilbert, 1984). It occurs in dry green beans of arabica and robusta coffees at levels of 0.9–1.4% (average, 1.1%) and 1.5–2.6% (average, 2.2%; Macrae, 1985), respectively. Darkly roasted coffee beans may contain about 20% more caffeine by weight than green beans (Gilbert, 1981; see the monograph on coffee, p. 67). The level of caffeine in tea (Camellia sinensis) is affected by a wide variety of parameters, including seasonal variations, genetic origin and use of nitrogen in fertilizers; thus, only average values can be estimated. The caffeine content of tea can be as high as 5% (Graham, 1984a) but is usually around 3.5% (Gilbert, 1984). The weighted average caffeine level in tea sold in the USA is approximately 3.0% (Graham, 1984a); those in tea sold in the UK range from 2.7 to 3.2% (Kazi, 1985; see also the monograph on tea, p. 223).
Cacao is a major source of theobromine and contains only small amounts of caffeine; significant differences in the caffeine content of dried unfermented and fermented cotyledons have been found, as well as in the bark, beans, leaves, roots and pods of Theobroma cacao. The bean is the main caffeine storage site, and there are only traces in the leaves and pods (Somorin, 1974). Less caffeine (0.066% in original and 0.152% in fat-free material) is found in cotyledons of fermented West African cacao beans than in unfermented cotyledons (0.085 and 0.196%, respectively). The mean concentrations in fat-free samples of Amelonado and Amazonas cacao beans after five days’ fermentation were: green beans, 0.06 and 0.19%; yellow beans, 0.09 and 0.18%; orange beans, 0.08 and 0.23%; and black beans, 0.10 and 0.22%. Concentrations of caffeine in 16 other samples of various origins were 0.07–1.70% (Shively & Tarka, 1984). Cocoa grown in Africa contains as much as 1.7% caffeine (Graham, 1978). The average caffeine content of 22 samples of various chocolate liquors was 0.214% (compared to 1.22% theobromine) (Zoumas et al., 1980).
Caffeine occurs in the Ilex paraguariensis plant from which the South American beverage mate is prepared and in other plants of the holly species (see also the monograph on mate, p. 276). Caffeine levels in mate vary from 0.9 to 2.2%; the age of the leaf is an important determinant of the caffeine content: young, growing leaves, 2.0–2.2%; adult, one-year old leaves, 1.6%; two-year old leaves, 0.68% (Graham, 1984b).
(b) Occupational exposure
No data were available to the Working Group.
(c) Air
Caffeine has been detected in the air of New York City and in New Jersey, USA, mainly due to emissions from coffee roasting plants (Dong et al., 1977).
(d) Water sediments
Caffeine was not found in US industrial effluents (Perry et al., 1979) or drinking-water (National Research Council, 1977a).
(e) Food and beverages
The monographs on coffee, tea and mate contain extensive information on the methylxanthine content of these beverages. The occurrence in and consumption of caffeine in foods and beverages has been reviewed extensively (National Research Council, 1977b; Graham, 1978; National Soft Drink Association, 1982; Pao et al., 1982; Barone & Roberts, 1984; Gilbert, 1984; Graham, 1984a; Hirsh, 1984; Shively & Tarka, 1984; Lelo et al., 1986; National Soft Drink Association, 1986; Stavric & Klassen, 1987; Schrieber et al., 1988; Stavric et al., 1988; Debry, 1989; National Research Council, 1989).
One or more caffeine-containing foods or beverages (coffee, tea, cocoa and chocolate products, soft drinks and mate) is consumed by most adults and children, although 90% of caffeine consumed is in the form of coffee or tea (Gilbert, 1984). A wide variety of values for caffeine content have been reported, especially in coffee (Burg, 1975a; Barone & Roberts, 1984). The caffeine content of natural products varies according to the plant species, growing conditions, the amount used and the method of brewing (e.g., brewing time) and preparation (Barone & Roberts, 1984). Many early values were determined using a variety of analytical methods, often undocumented, and different volumes (‘cup’ size) (Burg, 1975a; Barone & Roberts, 1984; Stavric & Klassen, 1984). The caffeine contents of a variety of food products are given in Table 1.
Table 1.
Caffeine content of various beverages and food products.
In foods and beverages consumed in Australia, reported caffeine levels were 300 mg/100 g in cocoa beans, 6–42 mg/100 g in cocoa drinks, 6 mg/30 g in milk chocolate and 35 mg/30 g in cooking chocolate (Anon., 1983). [The Working Group estimated that the average caffeine level in cola drinks was 120 mg/l.]
In the UK, the average caffeine content per cup is estimated to be 48.2 mg in instant coffee, 100 mg in percolated filter coffee, 55.2 mg in tea (theobromine, 2.3 mg) and 10 mg in colas.
The caffeine content in 12-oz servings of 22 soft drinks in the USA ranged from 30 to 58.8 mg (US Food and Drug Administration, 1984). In some countries, the caffeine content of soft drinks is not indicated on the label, and it thus may be consumed unwittingly (Galasko et al., 1989).
The mean caffeine levels in 39 tinned ‘regular’ soft drinks in New York State, USA, and Ontario, Canada, in 1986 were analysed: those in cola drinks were 34.3 and 22 mg/tin (concentration range, 2.3–133.4 and 0.1–104.9 μg/ml), respectively. The range of caffeine contents in all products was 0.8–50.8 mg/tin (12-oz [355 ml]) in New York State and 0.03–29.4 mg/tin (280-ml) in Ontario. Comparison with earlier reports (47.3 mg/tin in the USA in 1979; 40 mg/tin in Ontario in 1976) indicated a general decrease in the amount of caffeine in all types of cola beverages over the seven-year period (Stavric & Klassen, 1987).
The caffeine content of 14 soft drinks sold in the USA in 1989 ranged from 36 to 54 mg/12-oz serving. Another soft drink contained 72.0 mg/12-oz serving (Anon., 1989b).
Data on human consumption of caffeine are generally based on overall product usage or on a relatively small number of dietary consumption surveys. A gross estimate of consumption of caffeine can be derived by considering per-caput intake (International Coffee Organization, 1981, 1982; Barone & Roberts, 1984; Gilbert, 1984; Hirsh, 1984; International Coffee Organization, 1989). In the USA and Canada, coffee is the most important source of caffeine, accounting for approximately 75 and 60% per-caput intake, respectively; tea accounts for 15 and 30% caffeine intake, respectively (Barone & Roberts, 1984).
Gross estimates of world caffeine consumption (in coffee, tea, cocoa and soft drinks) compared to that in the Canada, Sweden, the UK and the USA for 1981 or 1982 and based on total caffeine consumption and per-caput consumption are shown in Table 2. Estimates of world consumption were derived by taking production data for 1981, converting to caffeine by assuming a 75:25 mixture of arabica coffee at 1.1% and robusta coffee at 2.2%, and tea at 3.5%, and then deducting 20% for spoilage and waste. Estimates of ‘other’ consumption include cocoa (approximately 1350 tonnes of caffeine), mate (approximately 1250 tonnes), chewed cola nuts and miscellaneous sources. Total world caffeine consumption in 1981 was estimated to be approximately 120 000 tonnes, equivalent to 70 mg per day for each inhabitant. Approximately 95% of all caffeine consumed is contained in coffee and tea. The per-caput rate of caffeine use in the USA and Canada is approximately three times that in the world as a whole, but only half that of countries, such as the UK, with heavy tea consumption (Gilbert, 1984).
Table 2.
Estimates of caffeine consumption: world, North America, Sweden and UK, 1981 or 1982.
Daily caffeine intake in the USA has been estimated at 200 mg on the basis of total US consumption (Graham, 1978; Barone & Roberts, 1984). Specific studies have indicated levels as high as 334 (Barone & Roberts, 1984) and 1022 mg/day (Stavric et al., 1988). In the Nordic countries, nearly all caffeine is derived from coffee, and consumption is estimated at 340 mg per day (Kraft General Foods, 1989). Overall mean daily caffeine intake in the UK was estimated to be 359 mg (smokers, 421 mg; nonsmokers, 329 mg); coffee contributed 55% of the total intake of caffeine, and this percentage was higher in men than in women; tea contributed 44%, with no sex difference. In Australia, the average daily intake of caffeine from all sources was estimated to be 240 mg per person (coffee, 54%; tea, 41%; soft drinks, 5%) (Shizlow, 1983) with a maximal average of 6.8 mg/kg bw for adult men (Lelo et al., 1986). Assuming that the mate leaf yields 0.5% caffeine, the average daily consumption of caffeine in Paraguay and Uruguay from this source was estimated to be 85 mg (Gilbert, 1984).
Per-caput consumption of coffee, tea, cocoa and soft drinks in the USA and Canada in selected years between 1960 and 1982 is shown in Table 3. Total caffeine consumption from coffee in 1982 in the USA was estimated to be 10 300 tonnes, equivalent to a per-caput consumption of 125 mg/day. Per-caput tea-leaf consumption in the USA in 1982 was 0.4 kg, giving an approximate caffeine consumption from tea of 2850 tonnes, which is equivalent to a per-caput consumption of 35 mg/day — the amount of caffeine in one cup of weak-to-medium strength tea. Table 3 shows a 231% increase in per-caput consumption of soft drinks in the USA during the period 1960–82 (Gilbert, 1984).
Table 3.
Per-caput consumption of caffeine-containing beverages, Canada and the USA, in selected years.
Table 4 gives the consumption of coffee, tea and soft drinks in the USA in 1962 and in 1985–89 and shows that coffee is now the second most popular beverage after soft drinks.
Table 4.
Consumption of coffee, tea and soft drinks in the USA, 1962 and 1985–89.
Gilbert (1984) estimated that the total intake of caffeine-containing soft drinks in the USA in 1982 was 28.7 billion litres, giving a total caffeine yield of 2850 tonnes and a daily per-caput consumption of approximately 35 mg (see Table 2). The total soft drink sales for 1988 in the USA were estimated to be 7.5 billion 192-oz cases, equivalent to a per-caput consumption of 45.9 gallons [174 1]. Caffeine-free soft drinks accounted for about 4.5% of the market (Anon., 1989c).
Table 5 gives estimations of the consumption of methylxanthines (caffeine, theophylline and theobromine) from foods and beverages; estimates of per-caput consumption are based on the 1980 US census population of 226.5 million. Coffee accounted for the majority of caffeine consumption (72.3%; 140.7 mg/day), and tea accounted for 11.5%; all other sources combined accounted for 16.2%. Total per-caput intake of methylxanthines was estimated to be 233.79 mg/day, of which 194.6 mg (83.2%) were from caffeine, 39.05 mg (16.7%) from theobromine and 0.14 mg (0.1%) from theophylline (Hirsh, 1984).
Table 5.
Consumption of methylxanthines in foods and beverages, USA, 1980.
Additional data on caffeine consumption come from a national household census covering a 14-day (Barone & Roberts, 1984) period in 1972–73 (Federation of American Societies for Experimental Biology, 1978); Table 6 gives mean daily consumption of caffeine by source, and Table 7 gives mean daily consumption of individuals in the 90th–100th percentiles of caffeine intake from all sources. Another survey (Morgan et al., 1982) gave daily caffeine consumption and that over a seven-day period, by age group (Table 8), and the mean daily caffeine consumption by source (Table 9). From these data, it was estimated that mean daily caffeine intake is approximately 3 mg/kg bw for all adults in the general population and approximately 4 mg/kg bw for consumers of caffeine. Among the 10% of adults who consumed the most caffeine, mean intake was approximately 7 mg/kg bw per day; for children under 18 years of age, the mean daily intake was approximately 1 mg/kg bw; and the 10% of children who consumed the most caffeine had a mean intake of approximately 3–5 mg/kg bw (Barone & Roberts, 1984).
Table 6.
Mean daily consumption of caffeine by source for subjects in a US national household census.
Table 7.
Mean daily consumption of caffeine by subjects in a US national household census in the 90th to 100th percentiles of caffeine intake from all sources.
Table 8.
Mean daily caffeine consumption by respondents in a US survey.
Table 9.
Mean daily caffeine consumption by source for respondents in a US survey and for caffeine consumers on days of consumptiona.
In the US National Food Consumption Survey, 1977–78 (Pao et al., 1982), the average daily caffeine consumption from coffee, tea and cola was estimated for those respondents who consumed the particular beverage for at least three days (Barone & Roberts, 1984; Table 10). Average caffeine consumption among US tea drinkers was 76.2 mg/day. For cocoa and hot chocolate drinkers, caffeine intakes ranged from 0.06 to 0.22 mg/kg bw for children and averaged about 0.05 mg/kg bw for adults. Chocolate milk consumption gave caffeine intakes of 0.02–0.06 mg/kg bw for adults and 0.05–0.19 mg/kg bw for children. Children who consumed coffee and tea had similar caffeine intakes; adult consumers of coffee had a higher intake of caffeine than tea drinkers. Using caffeine contents of 85, 60 and 3 mg per cup for ground roasted, instant and decaffeinated coffee, respectively, the daily caffeine intake for all coffee drinkers was estimated to be 233 mg. From these data, which cover only consumers of caffeine-containing products rather than all individuals, it was estimated that, depending on age and sex, the mean daily caffeine intake from coffee for adult (≥19 years) coffee drinkers ranged from 2.7 to 4.0 mg/kg bw, that from tea, 0.9–1.4 mg/kg bw, and that from colas, 0.23–0.47 mg/kg bw (Barone & Roberts, 1984).
Table 10.
Average daily caffeine consumption from different beverages in the US National Food Consumption Survey.
2.3. Analysis
Analytical procedures for the determination of caffeine and its metabolites and other xanthines in biological fluids (Christensen & Whitsett, 1979; Tobias, 1982; Christensen & Neims, 1984; Hurst et al., 1984) and in foods (Hurst et al., 1984) have been reviewed. Until the mid 1970s, the usual technique for determining caffeine in biological fluids was ultraviolet spectroscopy (Axelrod & Reichenthal, 1953; Routh et al., 1969). Use of thin-layer chromatography (Welch et al., 1977; Riechert, 1978; Bradbrook et al., 1979), gas chromatography (Grab & Reinstein, 1968; Demas & Statland, 1977; Bradbrook et al., 1979) and gas chromatography-mass spectrometry (Merriman et al., 1978) for the analysis of caffeine in plasma has been described. The minimal level of caffeine detected in plasma by thin-layer chromatography was 0.1 µg/ml (Bradbrook et al., 1979) and that in serum, saliva or urine was 1 µg/ml (Riechert, 1978); the level of detection of caffeine in plasma by gas chromatography was 0.05 µg/ml (Bradbrook et al., 1979).
High-performance liquid chromatography is currently the most frequently used procedure for determining caffeine and its metabolites and for separating caffeine from other xanthines and drugs in biological fluids; detection techniques range from fixed-wavelength ultraviolet and variable ultraviolet to electrochemical methods, and separation techniques range from normal and reverse-phase to ion pairing (Sved & Wilson, 1977; Aldridge & Neims, 1979, 1980; Christensen & Whitsett, 1979; Tin et al., 1979; Foenander et al., 1980; Van der Meer & Haas, 1980; Christensen & Isernhagen, 1981; Haughey et al., 1982; Muir et al., 1982; Klassen & Stavric, 1983; O’Connell & Zurzola, 1984; Stavric & Klassen, 1984; Kapke & Franklin, 1987; Papadoyannis & Caddy, 1987; Wong et al., 1987; Meatherall & Ford, 1988).
Additional procedures for the determination of caffeine in biological fluids include chromatography on ion-exchange resins (Walton et al., 1979), radioimmunoassays (Cook et al., 1976) and enzyme immunoassay techniques (Aranda et al., 1987).
Caffeine has been determined and separated from theobromine and theophylline in foods and beverages, including coffee, tea and cocoa, and in drug formulations by a variety of techniques (Hurst et al., 1984). Earlier procedures utilized spectrophotometry (Fincke, 1963; Ferren & Shane, 1968; Somorin, 1973, 1974; Horwitz, 1980a), titrimetry (Mayanna & Jayaram, 1981), column chromatography (Levine, 1962; Johnson, 1967) and Kjeldahl nitrogen determination (Moores & Campbell, 1948). These were followed by paper chromatography (Jalal & Collin, 1976), thin-layer chromatography (Senanayake & Wijesekera, 1968, 1971; Somorin, 1974; Jalal & Collin, 1976) and gas chromatography (Horwitz, 1980b). High-performance liquid chromatography is most widely used at present for determining caffeine and other methylxanthines in foods and beverages (Madison et al., 1976; Kreiser & Martin, 1978; Timbie et al., 1978; Horwitz, 1980c; Zoumas et al., 1980; De Vries et al., 1981; Reid & Good, 1982; Blauch & Tarka, 1983; Craig & Nguyen, 1984; Vergnes & Alary, 1986).
Methods used for determining caffeine in green, roasted and instant coffee have been reviewed by Macrae (1985).
3. Biological Data Relevant to the Evaluation of Carcinogenic Risk to Humans
3.1. Carcinogenicity studies in animals
The Working Group was aware of experiments (e.g., by Macklin & Szot, 1980) that were parts of studies on the modifying effects of caffeine on the activity of known carcinogens, in which a group given caffeine was frequently incorporated as a control group. Only a few of these studies were included here, since the experimental design of most of them was inadequate to reveal a possible carcinogenic effect of caffeine: i.e., short duration of exposure to caffeine and/or the histopathological examination was limited to the target organ of the carcinogen used.
(a) Oral administration
Mouse: In a series of experiments (Welsch et al., 1988a) (see also p. 316), groups of 37–43 female C3H mice, eight weeks of age, received caffeine [purity unspecified] at 0 (control), 250 or 500 mg/l drinking-water for 43 weeks. At termination of the study all mammary tumours were excised, fixed in Bouin’s fluid (Welsch et al., 1988b) and examined histologically. The incidence of mammary carcinomas, mean time to tumour appearance and body weight gain were not significantly affected by caffeine treatment; however, the number of mammary adenocarcinomas per animal had significantly increased (p < 0.05) among those given 500 mg/l caffeine. [The Working Group noted the short treatment period and that histopathological examination was limited to the mammary gland.]
Rat: Three groups each of 50 male and 50 female Wistar rats, eight weeks of age, were maintained on basal diet and given tap-water (controls) or a 0.1% [1000 mg/l] solution of synthetic caffeine (purity 100%; total amount consumed, 14.5 g for males and 13.9 g for females) or a 0.2% caffeine solution (total amount consumed, 26.6 g for males and 21.7 g for females) as the drinking fluid for 78 weeks. Surviving rats were then given tap-water for a further 26 weeks. The numbers of tumour-bearing animals were 24/46 in control males and 41/50 in females; 31/48 in males given 0.1% caffeine and 44/48 in females; and 18/44 in males given 0.2% caffeine and 37/50 in females. The numbers of tumours at specific sites were not significantly different in treated and control rats (Takayama & Kuwabara, 1982).
Groups of 50 male and 50 female Sprague-Dawley rats, 28 days of age, received food-grade natural caffeine (containing less than 0.01% theobromine) at 200, 430, 930 or 2000 mg/l drinking-water for 104 weeks. Mean daily intakes were 12, 26, 49 and 102 mg/kg bw in males and 15, 37, 80 and 170 mg/kg bw in females. Two control groups of 50 male and 50 female rats received tap-water only. No significant increase in the numbers of tumour-bearing rats or of tumours at specific sites was observed in treated as compared to control groups (see Table 11). A slight increase in mortality was seen in males at the highest dose; a decrease in body weight was seen in males and females at the higher doses; decreased numbers of tumour-bearing animals and of tumours per animal were observed in males and females treated with the highest dose (Mohr et al., 1984). [The Working Group noted that the reduced number of tumours per animal might have been due partly to impaired growth.]
Table 11.
Overall tumour response in rats treated with caffeine at different doses in the drinking-water.
A group of 40 female Wistar rats, four weeks of age, was given 0.2% [2000 mg/l] caffeine solution [purity unspecified] as the drinking fluid ad libitum for 12 months (mean total dose of caffeine, 13.5 g per rat). A group of 40 controls (reduced to 30 at the end of the study) was given tap-water only. The number of pituitary adenomas (22/40) in the caffeine-treated group was significantly (p < 0.02) greater than that in controls (8/30). Pituitary hyperplasia was seen in 5/40 rats given caffeine and in 1/30 controls (Yamagami et al., 1983). [The Working Group noted the short duration of the study, that evaluation was limited to the pituitary gland and to one sex, and that ten control rats were not evaluated since they died before the end of the study.]
As part of a study of the carcinogenicity of analgesics, 30 male Sprague-Dawley rats, six weeks of age, were given 0.102% caffeine (purity, 99.6–99.9%) in the diet for up to 117 weeks; caffeine consumption was 21.4 g per rat. A control group of 30 males received basal diet alone. The mean survival times were 78 weeks in the treated group and 94 weeks in the controls. No difference in tumour incidence was found between the treated group (8/28) and controls (6/30) (Johansson, 1981). [The Working Group noted that only one sex was used and the high mortality in the treated group.]
Groups of 40 male and 40 female Sprague-Dawley rats, weighing approximately 100 g, were fed diets containing 6% instant coffee (13 samples of coffee, including seven from which caffeine had been removed by extraction with dichloromethane; in three of these, caffeine has been restored to the coffee) for 24 months, at which time all survivors were killed. A control group of 40 males and 40 females was available. In general, survival was similar in all groups (males given decaffeinated coffees had a slightly lower death rate), but body weights of treated males were lower than those of controls. No increase in the number of tumour-bearing animals or of tumours at specific sites was observed in the group receiving decaffeinated coffee with added caffeine as compared to animals receiving decaffeinated coffee or left untreated. The incidence of tumours (benign and malignant combined) was significantly lowered (p < 0.05) in males in two of the six groups given coffee and in one of the three groups given decaffeinated coffee with added caffeine; in females, the decrease was not significant (Wurzner et al., 1977a,b). [The Working Group noted that comparisons of numbers of tumours per animals were made and that the reduced number of tumours found might have been due partly to impaired growth.]
As part of a study on modifying effects (see p. 314), 32 male and 32 female Sprague-Dawley rats, ten weeks of age, were administered caffeine [purity unspecified] at 100 mg/kg bw (annual dose, 27 g/kg bw) by intragastric instillation five times a week for life. Mean survival time was 102 weeks in treated and 129 weeks in control animals. The number of tumour at distant organ sites was lower in the caffeine-treated group than in the controls; local tumours were seen in six caffeine-treated rats and in three controls (Brune et al., 1981). [The Working Group noted the limited reporting of the data and that the difference in survival times may have influenced the results.]
(b) Intraperitoneal administration
Mouse: In a screening assay based on the accelerated induction of lung adenomas in a strain highly susceptible to development of this neoplasm, groups of 40 male strain A mice, six weeks old, were given intraperitoneal injections of caffeine [purity unspecified] at 8, 20 or 40 mg/kg bw in saline, three times a week for eight weeks. A group of controls was given injections of saline only. Twenty-four weeks after the first injection, the mice were sacrificed. The number of surface adenomas was counted macroscopically. All three doses of caffeine decreased the incidence of lung tumours, but this effect was significant (p < 0.05) only with the highest dose (Theiss & Shimkin, 1978). [The Working Group noted that only lung tumours were examined.]
(c) Administration with known carcinogens
These studies are summarized in Table 12 on p. 318.
Table 12.
Summary of results of studies with caffeine in combination with known carcinogens.
(i) Morpholine plus sodium nitrite
Groups of 33–34 male strain A mice, ten weeks of age, were fed 6.35 g/kg of diet morpholine and received 2.0 g/l sodium nitrite in the drinking-water either alone (controls) or in combination with 1 g/kg of diet caffeine [purity unspecified] on five days a week for 20 weeks. Mice were killed when 40 weeks old. The number of surface adenomas in the lungs of the group treated with caffeine (6.0 ± 0.7) was significantly (p < 0.001) lower than that in the control group (17.1 ± 1.3) (Mirvish et al., 1975).
(ii) N-Nitrosodiethylamine
Groups of 25 and 30 male BDVT and Wistar rats received 0 or 600 mg/l caffeine in the drinking-water followed three days later by weekly intraperitoneal injections of 80 mg/kg bw N-nitrosodiethylamine (NDEA) for 10 weeks. Treatment with caffeine was continued for a further two weeks, and all animals were killed 24 weeks after the beginning of NDEA treatment. There was high mortality (40%) in the caffeine-treated group. Addition of caffeine to the drinking-water decreased the number of liver tumours induced by NDEA (p < 0.05): the average numbers of tumours were 1.17 ± 0.225 in the group treated with NDEA and caffeine and 3.23 ± 0.667 in the group treated with NDEA alone (Balansky et al., 1983). [The Working Group noted that the reduction could be attributed to the high mortality in the caffeine-treated group.]
(iii) 4-Nitroquinoline-1-oxide
A total of 339 male and 285 female ICR/Jcl mice, 21 days of age, were divided into five groups and received a single subcutaneous injection in the right flank of 12.5 µg/g bw 4-nitroquinoline-1-oxide (4NQO) dissolved in propylene glycol and five subcutaneous injections of 100 μg/g bw caffeine [purity unspecified] dissolved in water at intervals of 6–12 h: Groups 1 and 2 received caffeine 0–36 h or 120–156 h after 4NQO treatment; group 3 received caffeine 12–18 h before 4NQO treatment; group 4 received an equal volume of water instead of caffeine solution during the 0–36 h after 4NQO treatment; and group 5 received an equal volume of propylene glycol instead of 4NQO solution and caffeine during the following 0–36 h. The doses were the maximum tolerated doses. The mice were killed 20 weeks after 4NQO treatment and examined for the presence of lung tumours. The numbers of tumours were as follows: group 1, 26/57 males and 24/54 females (p < 0.05); group 2, 8/26 males and 15/28 females; group 3, 10/22 males and 17/28 females; group 4, 41/98 males and 37/57 females (Nomura, 1976). Similar results were obtained by Nomura (1980).
Two groups of 100 female ICR-Jcl mice, six weeks of age, received a single irradiation with a surface dose of 3 krad β-rays on an area of skin 2 cm in diameter followed 10 days later by skin applications of 0.1 mg 4NQO in benzene three times a week for a total of 20 applications. One of these groups received 0.8 mg caffeine [purity unspecified] in benzene painted onto the same site on alternate days from the 4NQO applications. The study was terminated after 94 weeks. Caffeine significantly (p < 0.01) increased the incidence of squamous-cell carcinomas of the skin: 21/96 in the group treated with β-rays and 4NQO; 43/94 in the group treated with β-rays, 4NQO and caffeine (Hoshino & Tanooka, 1979). [The Working Group noted the limited reporting of the experiment.]
(iv) 4-Hydroxyaminoquinoline-1-oxide
Groups of 9–18 male Wistar rats, six weeks of age, received a single intravenous injection of 7 mg/kg bw 4-hydroxyaminoquinoline-1-oxide (4HAQO) in hydrochloric acid three days after partial pancreatectomy; they then received 6 or 12 subcutaneous injections of 120 mg/kg bw caffeine in saline (maximum tolerated dose) at 12-h intervals from 0 to 72 h (group 3), 72 to 132 h (group 4) and 0 to 132 h (group 5). Control rats received hydrochloric acid instead of 4HAQO (group 1) or 4HAQO plus saline instead of caffeine from 0 to 132 h (group 2). The animals were sacrificed 52 weeks after 4HAQO treatment. Growth retardation of 10–20% was observed in groups 3, 4 and 5 compared to groups 1 and 2, but surviving rats recovered growth after the last treatment with caffeine. The numbers of acinar-cell adenomas of the pancreas were not significantly different in the various groups; the total number of macroscopic nodules per pancreas was 16.8 ± 6.5 in group 2 and significantly lower (p < 0.01) in groups 3, 4 and 5 (3.5 ± 2.0, 3.3 ± 1.2 and 2.9 ± 2.3). No tumour was identified as an adenocarcinoma. In another experiment, rats received six subcutaneous injections of 120 mg/kg bw caffeine before the 4HAQO treatment (7 mg/kg) (group 2), and three other groups of rats received 12 subcutaneous injections of caffeine from 0 to 132 h after the 4HAQO treatment at doses of 120 (group 3), 60 (group 4) and 30 mg/kg bw (group 5). The animals were sacrificed at 52 weeks. The incidence of acinar-cell adenomas was 100% in all of the groups, and the numbers of pancreatic nodules in groups 4 and 5 were higher (significant only in group 5) than in group 1, which received saline instead of caffeine (Denda et al., 1983).
(v) Urethane
Groups of 30 female ICR mice, six to eight weeks of age, received a single subcutaneous injection of 25 mg/ml urethane in saline [exact dose unspecified], followed two weeks later by a topical application of anthranil twice a week on clipped dorsal skin. Single subcutaneous injections of 100 µg/g bw (maximum tolerated dose) caffeine [purity unspecified] were given at various times between 24 h before and 6 h after injection of urethane. All animals were sacrificed 45 weeks after the beginning of anthranil treatment. Caffeine significantly (p < 0.05) enhanced the incidence of papillomas of the skin when given 6 h before urethane treatment but had no significant effect when given at any other time tested (Armuth & Berenblum, 1981). [The Working Group noted the use of the inadequate promotor.]
Groups of 20 male strain A mice, six weeks of age, were given a single subcutaneous injection of 0.25 or 1.0 mg/g bw urethane in saline solution. Intraperitoneal injections of 20 or 40 mg/kg bw caffeine [purity unspecified] were given three times a week for eight weeks beginning either seven days before, seven days after or on the same day as (3 h before and 3 h after) urethane injection. All mice were sacrificed 16 weeks after the urethane injection. Caffeine treatment beginning seven days before the high dose of urethane resulted in a significant (p < 0.01) suppression of the lung tumour response within the experimental period. Similarly, when caffeine treatment was given on the same day as the urethane injection, the lung tumour response was significantly (p < 0.01) suppressed at both doses of urethane (Theiss & Shimkin, 1978).
Groups of female ICR/Jcl mice [initial numbers unspecified], 25 days of age, received a single subcutaneous injection of 0.1 mg/g bw urethane followed immediately by seven intraperitoneal injections of caffeine [purity unspecified] at 0.05 μmol[10 μg]/g bw at 6-h intervals up to 36 h after urethane treatment. Mice were killed five months after urethane treatment. The incidence of lung tumours was 7/32 in the group given caffeine and 31/59 in those given only urethane (p < 0.01) (Nomura, 1983). [The Working Group noted that the effective numbers of mice varied considerably among the groups.]
(vi) 2-Acetylaminofluorene
Groups of 15 or 20 male ACI rats, six weeks of age, were given 0.02% 2-acetylaminofluorene (2AAF) (purity, > 95%) in the diet and 0 or 0.2% caffeine (purity, > 98%) in the drinking-water (total caffeine intake, 3.26 ± 0.34 g/rat) for 18 weeks and were maintained on basal diet and caffeine-free water until 33 weeks, at which time the experiment was terminated. The number of tumours per animals but not the incidence of liver tumours was significantly (p < 0.001) lower in the group treated with 2AAF and caffeine (3.8 ± 2.3 tumours per rat) as compared to the group treated with 2AAF alone (13.5 ± 5.3 tumours per rat). The authors noted that the total intake of 2AAF in the group also treated with caffeine (277 ± 13 mg/rat) was significantly (p < 0.01) lower than that of the group treated with 2AAF alone (302 ± 24 mg/rat) (Hosaka et al., 1984). [The Working Group noted that the finding may be due to different intakes of the carcinogen.]
(vii) Benzo[a]pyrene
Groups of 32 male and 32 female Sprague-Dawley rats, ten weeks of age, were either fed benzo[a]pyrene (BP) in the diet (average annual dose, 6 or 39 mg/kg bw) or administered BP by gavage in an aqueous 1.5% solution of caffeine [purity unspecified] (average annual dose, 6, 18 or 39 mg/kg bw) for life. The median survival time of rats given BP in caffeine was slightly shorter than that in the groups given BP in the diet (about 100 versus about 128 weeks). Groups given BP in caffeine developed more papillomas of the forestomach than groups given BP in the diet; this difference was statistically significant (chi-square test modified according to Peto) for the groups given 18 mg/kg bw (p < 0.01) and 39 mg/kg bw (p < 0.05) compared to untreated controls (Brune et al., 1981). [The Working Group noted the difference in routes of administration between the groups.]
(viii) Diethylstilboestrol
Groups of 24–30 ACI female rats, four months of age, received a subcutaneous implantation of pellets containing 5 mg diethylstilboestrol (DES) one week after the start of treament with caffeine [purity not specified] at 0, 1 mg/ml (approximately 60 mg/kg bw per day) or 2 mg/ml (approximately 120 mg/kg bw per day) in the drinking-water for 10.5 months, at which time all animals were killed. The average body weight of treated animals was 157.7 g at the end of the experiment, compared to 179 g in controls. Mammary tumours were excised surgically when 1 cm in diameter [and the animals were put back in the experiment]. Increasing caffeine dosage significantly (p < 0.05) lengthened the time to appearance of mammary tumours and decreased their incidence (DES alone, 12/24; DES and low-dose caffeine, 10/24; DES and high-dose caffeine, 3/30) and the number of tumours per animal (DES alone, 12 rats with 92 tumours; DES and low-dose caffeine, 10 rats with 29 tumours; DES and high-dose caffeine, three rats with three tumours; p < 0.05 for the high-dose group). The histological pattern of the tumours was not influenced by caffeine (Petrek et al., 1985). [The Working Group noted that the decrease in the number of mammary tumours per animal and their incidence in the high-caffeine group may have been related, at least in part, to the decrease in body weight.]
(ix) N-Nitroso-N-butyl(4-hydroxybutyl)amine
Six groups of 36 male Wistar rats, weighing on average 194 g, were given 0.01% or 0.05% N-nitroso-N-butyl(4-hydroxybutyl)amine (NBHBA) in the drinking-water for four weeks. One high-dose and one low-dose group received 0.1% (w/v) caffeine [purity unspecified] in the drinking-water for 32 weeks; a second pair of high-dose and low-dose groups received phenacetin at 2.5% in the diet for 30 weeks. Additional groups of 24 rats received either caffeine or phenacetin alone. Surviving rats were killed 36 weeks after the start of the experiment. Treatment with 0.05% NBHBA and caffeine did not change the incidence of bladder carcinomas (5/31 versus 4/27 with NBHBA alone), papillomas (15/31 versus 8/27) or papillary or nodular hyperplasia (22/31 versus 23/27). Similar results were obtained in animals treated with 0.01% NBHBA and caffeine (carcinomas, 0/28 versus 0/23; papillomas, 6/28 versus 3/23; papillary hyperplasia, 12/28 versus 6/23). Phenacetin, used as a positive control, significantly increased the incidences of tumours and of hyperplasia in the group receiving 0.05% NBHBA and of hyperplasia in those receiving 0.01%. No bladder lesion was found in caffeine controls (Nakanishi et al., 1978).
Groups of 40 male Wistar rats, eight weeks of age, were given 0.01% NBHBA in the drinking-water for four weeks followed by 0.1% caffeine [purity unspecified] for 32 weeks or were treated simultaneously with 0.001% NBHBA and 0.1% caffeine in the drinking-water for 40 weeks; similarly, NBHBA-treated groups received sodium saccharin alone (5.0% in the diet) or saccharin plus caffeine for the same length of time. At the end of the treatment period, all surviving animals were killed. Simultaneous administration of caffeine with 0.001% NBHBA did not modulate urinary bladder carcinogenesis, since no tumour was noted, whereas rats given saccharin or saccharin plus caffeine had papillomas (10/24 or 9/32 versus none in NBHBA controls) and carcinomas (2/24 and 1/32 versus none). When NBHBA (0.01%) and caffeine were given sequentially, only papillomas were seen in the caffeine-treated group (6/28 versus 3/23 in NBHBA controls). Treatment with saccharin or saccharin plus caffeine increased the incidences of hyperplasia significantly, but no increase was noted for papillomas (NBHBA plus saccharin, 9/31; NBHBA plus saccharin plus caffeine, 4/30; NBHBA controls, 3/23) or carcinomas (NBHBA plus saccharin, 1/31; none in the others) (Nakanishi et al., 1980).
Eight groups of 45 female Wistar rats, weighing 180–200 g, received three consecutive administrations of 100 mg/kg bw NBHBA by gavage at 24-h intervals with continuous administration of either 110 mg/kg bw per day caffeine (purity, 100%) in the drinking-water or 500 mg/kg bw phenacetin in the diet. Four groups (those receiving NBHBA, NBHBA plus caffeine, NBHBA plus phenacetin or NBHBA plus caffeine plus phenacetin) were terminated after 15 months and the other four groups after 21 months. Neither caffeine nor phenacetin nor caffeine plus phenacetin influenced the incidence of bladder tumours significantly. At 15 months, the numbers of tumour-bearing rats were: NBHBA, 8/31; NBHBA plus phenacetin, 9/40; NBHBA plus caffeine, 15/39; and NBHBA plus caffeine plus phenacetin, 15/40. After 21 months, these numbers were 16/36, 16/34, 17/39 and 15/31, respectively (Kunze et al., 1987).
(x) 7,12-Dimethylbenz(a)anthracene
Groups of 54–55 female C57B1 × DBA/2fF1 (BD2F1) mice, eight weeks of age, received weekly intragastric intubations of 1 mg 7,12-dimethylbenz[a]anthracene (DMBA) for six weeks. One week after the last intubation the animals received 0 (controls), 250 or 500 mg/l caffeine [purity unspecified] in the drinking-water for 20 weeks, at which time the study was terminated. The number of mammary carcinomas per mouse was significantly (p < 0.05) increased in the group given 500 mg/l caffeine in drinking-water (0.7 versus 0.5 in controls). Caffeine did not significantly affect the number of mice with mammary carcinomas or the time to tumour appearance (Welsch et al., 1988a).
Four groups of 20 female Sprague-Dawley rats, 50 days of age, received a single gastric intubation of 20 mg DMBA. One group was then given standard rat chow and tap-water ad libitum; a second group received standard chow and 10 mg/kg bw caffeine in the drinking-water; a third group received tap-water with a diet of 20% vegetable fat; and a fourth group received vegetable fat diet plus the caffeine solution. Nine months after DMBA administration, the animals were killed and mammary tumours were examined histologically. The mean latency of mammary tumour development was significantly (p < 0.05) reduced in the groups given caffeine and fat, whereas in the group given caffeine alone the latency period was significantly (p < 0.05) lengthened. In the groups maintained on high fat alone, the latency was similar to that of animals on standard chow. The combination of caffeine and fat resulted in a larger number of tumours per rat than in the other three groups (Minton et al., 1983). [The Working Group noted, as did Pike and Bernstein (1985), that the analysis was based only on animals that developed tumours and may have been biased by differential survival in the various groups.]
Three groups of 30 female Sprague-Dawley rats, 30 days of age, were given 0 (control), 250 or 500 mg/l caffeine [purity unspecified] in the drinking-water for 30 consecutive days; at 57 days of age, all rats received single intragastric intubations of 5 mg DMBA in 1 ml sesame oil. Mammary tumours were excised surgically when they reached 2 cm in diameter, and the animals were put back in the experiment. The study was terminated 20 weeks after DMBA treatment. Three other groups of 30 females received single intragastric intubations of 5 mg DMBA in 1 ml sesame oil at 53 days of age followed three days later by 0 (control), 250 or 500 mg/l caffeine in the drinking-water. This study was terminated 21 weeks after DMBA treatment. Caffeine treatment of rats before or during DMBA treatment had no significant effect on the incidence of mammary carcinomas, the number of tumours per animal or the latency; caffeine treatment after DMBA treatment increased the incidence of mammary carcinoma (Welsch et al., 1983).
Groups of 40–41 female Sprague-Dawley rats were administered 20 mg/kg bw DMBA intravenously at 53–55 days of age; treatment with 100–860 mg/l caffeine [purity unspecified] in the drinking-water began 29 days before and ended three days after DMBA treatment. Mammary tumours were excised surgically when they reached 2 cm in diameter, and the animals were put back in the experiment. The study was terminated 12–18 weeks after DMBA treatment. Administration of caffeine before DMBA treatment did not significantly affect the incidence of mammary carcinomas, but the number of mammary tumours per rat was reduced. Further groups of 40–41 females received a single intragastric administration of 5 mg DMBA followed three days later by 100–800 mg/l caffeine in the drinking-water until 12 or 18 weeks after DMBA treatment. An increase in the number of mammary gland carcinomas per animal was observed (p < 0.05) when caffeine was administered after DMBA treatment for 12 weeks but not after treatment for 18 weeks. In neither instance did caffeine influence the incidence of mammary carcinomas or the time to appearance of tumours (Welsch et al., 1988b).
In a subsequent study with the same experimental design, a chemically defined diet containing standard (5%) or high (20%) levels of corn oil was used instead of commercial laboratory animal chow. Caffeine (430–500 mg/l) consumption before and during the DMBA treatment significantly (p < 0.05) reduced the number of mammary carcinomas per animal, whereas no effect was found on the number of carcinomas per animal when caffeine was administered after DMBA treatment. No effect on incidence or latency was seen in either case (Welsch & DeHoog, 1988).
(xi) Ultraviolet light
Groups of 54–57 female, nonhomozygous Swiss mice, 10–12 weeks old, were exposed to light from an Ellipiol mercury vapour lamp (irradiation time, 90 min), five times a week for a total of 133 exposures in 27 weeks (total dose, 1 × 107 ergs/mm2). Before each irradiation, 40 μl of a 0.2% solution of caffeine [purity unspecified] in acetone/chloroform was applied to the right ears. The same amount of solvent was applied to the left ears as a control. The first tumours appeared on the ears five months after and the last 11 months after the onset of irradiation. The incidence of tumours of ears treated with caffeine (47–54%) was significantly (p < 0.0001) lower than that on the left ear (consistently varying from 84–89%) (Zajdela & Latarjet, 1973, 1975, 1978a,b).
(xii) Cigarette-smoke condensate
Groups of 51 mice [strain unspecified], four to six weeks of age, received skin applications of 100 or 200 mg of two different fractions of cigarette-smoke condensate dissolved in isopropanol:acetone (20:80) three times a week alone or in combination with 0.04 and 0.2 mg caffeine or 0.08 and 0.4 mg caffeine, respectively. Reduced incidences of skin tumour-bearing animals were found in all caffeine-treated groups, except with the low dose of one condensate fraction (Rothwell, 1974).
3.2. Other relevant data
(a) Experimental systems
(i) Absorption, distribution, metabolism and excretion
The metabolism and pharmacokinetics of caffeine in animal species have been reviewed (Burg, 1975b; Lachance, 1982; Tarka, 1982; Arnaud, 1984; Bonati & Garattini, 1984; Bonati et al., 1984–85; Arnaud, 1987; Bonati & Garattini, 1988).
Animal experiments using radiolabelled caffeine showed its rapid and complete gastrointestinal absorption and distribution (Bonati & Garattini, 1984; Arnaud, 1985a). Caffeine is distributed to all body fluids (Bonati & Garattini, 1984) and appeared in all tissues within 5 min (Burg & Werner, 1972). There was no accumulation of caffeine or its metabolites in specific organs, even after high doses (Bonati & Garattini, 1984). No blood-brain barrier or placental barrier for caffeine was observed in adult or fetal animals (Maickel & Snodgrass, 1973; Bonati & Garattini, 1984; Kimmel et al., 1984; Tanaka et al., 1984). Using an experimental protocol established to study a single passage through the cerebral circulation, caffeine at very high blood levels (Ki = 9.8 mM) may restrict the availability of circulating purines to the brain (McCall et al., 1982).
The fraction of caffeine bound to plasma albumin varies from 10 to 30%. No significant first-pass effect occurs after oral administration. Caffeine is eliminated by various species by apparent first-order kinetics, described by a one-compartment open model system (Bonati & Garattini, 1984). The half-time for caffeine is 0.7–1.0 h in rats and mice, 1–1.6 h in rabbits, 3–5 h in monkeys, 4–4.3 h in dogs and 11–12 h in baboons (Christensen et al., 1981; Bonati & Garattini, 1984; Bonati et al., 1984–85; Bonati & Garattini, 1988). A mean volume of distribution of 0.81/kg has been reported for different species (Bonati & Garattini, 1984; Bonati et al., 1984–85).
Non-linear kinetics, shown in rats by disproportionate increases in the dose-concentration relationship, indicate a limited capacity to absorb and metabolize caffeine at doses of 10–25 mg/kg bw (Aldridge et al., 1977; Latini et al., 1978).
A decreased half-time was reported when 10 mg/kg bw caffeine were administered to pregnant rats in drinking-water on day 18 of gestation (Nakazawa et al., 1985); however a 25% decrease in mean total demethylation was demonstrated in rats between 19 and 21 days of pregnancy, with a breath test using [14C-1,3,7-methyl]caffeine at a dose of 4 mg/kg, with an immediate return to normal values one day after birth (Arnaud & Getaz, 1986). Rabbits receiving 8–22 mg/kg bw per day caffeine through 29 days of gestation exibited increased plasma concentrations in the last half of gestation, demonstrating that there is an increased half-time (Dorrbecker et al., 1988).
Caffeine is metabolized by liver microsomal mixed-function oxidases (Arnaud & Welsch, 1980a). It can increase drug-metabolizing enzyme activity at high doses (75 mg/kg bw) (Mitoma et al., 1969); however, in-vitro studies showed no induction or inhibition of microsomal enzyme activity after a six-day treatment with oral doses of 37.5 mg/kg bw caffeine (Aeschbacher & Wurzner, 1975). Enzyme induction was observed with doses of 100–150 mg/kg bw (Thithapandha et al., 1974; Aeschbacher & Wurzner, 1975; Govindwar et al., 1984), while, with lower doses (30–50 mg/kg bw), inhibition (Khanna & Cornish, 1973) or the absence of an effect (Ahokas et al., 1981) were reported. Inducers of cytochrome P450, such as 3-methylcholanthrene but not phenobarbital, increased caffeine clearance and shortened its half-time (Aldridge et al., 1977; Welch et al., 1977; Aldridge & Neims, 1979; Wietholtz et al., 1981).
In-vivo and in-vitro experiments showed a progressive increase in the activity of the hepatic microsomal enzymes that metabolize caffeine during neonatal development (Warszawski et al., 1981, 1982). In beagle puppies, change in caffeine clearance was determined by the rate of maturation of caffeine-7-demethylase (Aldridge & Neims, 1980). Caffeine is eliminated in animals by biotransformation in the liver to dimethylxanthines, dimethyl- and monomethyluric acids and uracil derivatives; important quantitative differences have been demonstrated in the formation and elimination of metabolites in rats, mice and Chinese hamsters (Arnaud, 1985b). These differences are even more important in monkeys, where caffeine is almost completely metabolized to theophylline (Gilbert et al., 1985, 1986). In addition to the metabolites shown in Figure 1, some species-dependent metabolites have been identified. Trimethylallantoin was first reported in rats in 1973 (Rao et al., 1973), and its chemical structure has now been reported (Arnaud et al., 1986a). A new derivative of paraxanthine was found in mice and identified as the 3-β-D-glucuronide of paraxanthine (Arnaud, 1985b; Arnaud et al., 1986b). Methylated ureas (Arnaud, 1976) and sulfur-containing derivatives (Kamei et al., 1975; Rafter & Nilsson, 1981) found in urine in trace amounts are produced by the intestinal flora. In contrast, the acetylated uracil derivative, 5-acetylamino-6-formylamino-3-methyluracil, one of the most important caffeine metabolites in humans, has not been identified in rodents or other animal species. Other uracil derivatives produced from caffeine, theobromine and paraxanthine in rats were found in human urine (Arnaud, 1984). In rats, the hepatic demethylation of caffeine shows an age-related decline, resulting in a greatly increased elimination half-time in older adult rats (Latini et al., 1980; Feely et al., 1987).
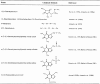
Fig. 1.
Caffeine metabolites found in animal species
The effects of dietary factors on methylxanthine metabolism have been reviewed (Anderson et al., 1982).
Pharmacokinetic differences have been observed in mice after oral administration of caffeine, which may account for interstrain variation in toxicity studies (Arnaud et al., 1989). In rabbits, two subpopulations could be described, with slow or rapid caffeine metabolizing capacity. Animals with slow metabolism exhibited saturation kinetics with high doses of caffeine and inhibition of caffeine metabolism by paraxanthine. Rabbits appear to be the best model to study the inter- and intrasubject variability in caffeine disposition observed in man (Dorrbecker et al., 1987).
Drug interactions with caffeine are known. Co-administration of caffeine was found to increase acetaminophen-induced hepatotoxicity by enhancing the production of a reactive metabolite (Sato & Izumi, 1989).
(ii) Toxic effects
The toxicity of caffeine has been reviewed extensively (Dews, 1982; Lachance, 1982; Tarka, 1982; Arnaud, 1987; Strubelt, 1987).
The acute oral LD50 of caffeine is 200 mg/kg bw in rats, 127 mg/kg bw in mice, 230 mg/kg bw in hamsters and in guinea-pigs and 246 mg/kg bw in rabbits; the intraperitoneal LD50s of caffeine are 200 mg/kg bw in rats and 235 mg/kg bw in guinea-pigs; and the intravenous LD50s of caffeine are 105 mg/kg bw in rats, 100 mg/kg bw in mice and 175 mg/kg bw in dogs. The toxicity of caffeine was determined after daily administration via intragastric cannula to female albino rats over 100 days (equivalent to 1/10 of the animals’ life span). Rats given daily doses slightly above the maximal LD50 (110 mg/kg bw) exhibited a stressor reaction in the form of hypertrophy of the adrenal cortex and atrophy of the adrenal cortex and thymus gland. Some animals manifested a psychotic-like mutilation, gastric ulcers, hypertrophy of the salivary glands, liver, heart, kidneys and lungs, inhibition of oogenesis, minor changes in organ water levels, and an occasional death apparently from bronchopneumonia. Although no major change in growth rates or eating and drinking habits was apparent, some polydypsia and diuresis, thyroiditis, occasional dermatitis, some degree of nephritis, and loss of red pulp in the spleen were seen (Tarka, 1982).
The sensitivity of rats to the lethal effects of caffeine increased with age; caffeine was more toxic in male than in female rats (Tarka, 1982).
The effects of caffeine on the rodent testis are reviewed in detail below and are not covered here. Caffeine also induced thymic atrophy at a dietary level of 0.5% (approximately 150 mg/kg bw) when fed for eight weeks to rats (Gans, 1984).
(iii) Effects on reproduction and prenatal toxicity
The effects of caffeine on reproduction and development in experimental animals have been reviewed (Mulvihill, 1973; Tarka, 1982; Wilson & Scott, 1984; Nash & Persaud, 1988; Nolen, 1988; Al-Hachim, 1989).
Reproductive effects: CD-1 mice were administered 0.012, 0.025 or 0.05% caffeine in the drinking-water (daily caffeine intake, 21.9, 43.8 or 87.5 mg/kg bw) for seven days prior to mating and during a subsequent 100-day cohabitation period. Offspring were removed when one-day old. The last set of litters from the high-dose group and the F1 generation of untreated controls were maintained on caffeine to 90 days of age and mated within their respective groups. Following treatment of the F0 mice, no effect on pregnancy rate was observed but there was a decrease in the number of live pups per litter at the high dose. There was no effect on any parameter in a cross-mated trial between control and high dose animals. Among the F1 males at termination of the study, there was no effect of caffeine on the weight of the testis or epididymus relative to body weight; there was a significant decrease in sperm motility, an increase in sperm density and no change in the proportion of abnormal sperm (Gulati et al., 1984).
In a similar study, no effect on pregnancy rate was observed in F0 and F1 mice, but among F0 groups there was a significant decrease in the number of live pups per litter at the two highest dose levels. There was no significant change in reproductive organ weight, sperm motility or density or in the frequency of sperm abnormalities (Reel et al., 1984).
Groups of male and female Wistar rats were administered 10 mg/kg bw caffeine in the drinking-water daily through five successive sets of litters. Progressively reduced growth and increased neonatal mortality (significant) were observed in the offspring over sequential pregnancies (Dunlop & Court, 1981).
As reported in an abstract, female rats [strain unspecified] in a two-generation reproduction study received daily oral administrations of 4, 20 or 126 mg/kg bw caffeine for seven days before mating and through to 20 days of lactation. The F1 offspring received the same treatment. When mature, F1 offspring were mated with untreated animals. Pregnancy rate and reproduction were normal in F0 females and F1 males; among F1 females, however, the pregnancy rate was normal, but there were decreases in the numbers of corpora lutea, implants and fetuses at the high dose. F2 fetuses of these high-dose females were small and oedematous (Bradford et al., 1983a).
Male and female Sprague-Dawley rats were given cocoa powder (containing 2.50–2.58% theobromine and 0.19% caffeine) in the diet at concentrations of 0, 1.5, 3.5 and 5.0% for three generations (Hostetler et al., 1990, see p. 430 of the monograph on theobromine). No consistent dose-related effect was observed in any reproductive index; nonreproductive toxicity was observed at the two highest dose levels.
Female monkeys (Macaca fascicularis), 12–14 per group, received 0, 10–15 or 25–35 mg/kg bw caffeine in the drinking-water daily on seven days a week for a minimum of eight weeks prior to mating with untreated males. Miscarriages and some stillbirths were reported during two cycles of pregnancy in the caffeine-treated groups, and birthweights of male infants was also significantly lower in these groups in comparison to controls. The effects were dose-related but occurred with both levels of caffeine. No malformation was observed in any of the offspring (Gilbert et al., 1988). [The Working Group noted that the exclusion criteria for stillbirths were not unequivocal.]
Ax et al. (1976) reported that when roosters were fed 0.1% caffeine [about 100 mg/kg bw per day] in a standard ration, hens inseminated with sperm from the roosters had significantly reduced numbers of fertile eggs. Semen and sperm counts were markedly reduced 17–21 days after treatment, and no semen could be collected after 30 days. These effects were reversible on removal of dietary caffeine.
Friedman et al. (1979) found that feeding caffeine in the diet to immature Osborne-Mendel rats at levels of 1% for three weeks and 0.5% [approximately 300 mg/kg bw per day] for 14–75 weeks produced severe testicular atrophy and aspermatogenesis. Analogous results were observed in Holtzman rats. [The Working Group noted the excessive doses used in the study.]
Developmental toxicity: Teratogenicity was reported in SMA mice given single intraperitoneal injections of 250 mg/kg bw caffeine on one of days 7–14 of gestation. Significant increases in the incidence of fetal resorptions, cleft palate and digital defects were observed, depending on the day of treatment (Nishimura & Nakai, 1960).
Subsequently, caffeine was shown to be teratogenic in rats and mice by oral intubation (Bertrand et al., 1965, 1970; Palm et al., 1978), by administration in the diet (Knoche & König, 1964; Fujii & Nishimura, 1972) and by administration in drinking-water (Knoche & König, 1964; Palm et al., 1978; Elmazar et al., 1982). The most common effects observed were digital defects, resorptions and cleft palate. Six out of 64 offspring of rabbits administered 100 mg/kg bw caffeine on days 1–25 of gestation were reported to have ectrodactyly (Bertrand et al., 1970). [The Working Group noted that no control group was used in this study; however, this is the only study in rabbits reported.]
In order to establish a no-effect level, caffeine was administered to Osborne-Mendel rats by gavage; offspring had dose-related increases in the frequency of ectrodactyly and delayed ossification. A no-effect level for terata was 40 mg/kg bw caffeine per day, although a significant increase in the frequency of delayed sternebral ossification was observed with 6 mg/kg bw per day (Collins et al., 1981). When administered in the drinking-water at a wider dose range (10–204 mg/kg bw per day), caffeine did not induce dose-related gross anomalies. Sternebral ossification was seen less frequently in all treated groups than in controls, except with the lowest dose (Collins et al., 1983). [The Working Group concluded that caffeine was less toxic to the developing embryo and fetus when given in drinking-water than by gavage; this pattern of exposure to caffeine — small doses throughout the day — is closely similar to human exposure to caffeine.]
In another study by Collins et al. (1987), the previously reported delay in sternebral ossification was confirmed in day-20 fetuses of rats drinking caffeine-containing water from gestation day 0 to day 20. Among offspring that were raised to postnatal day 6, the delay in ossification was nearly reversed. The authors concluded that the reversal would have been complete if a longer postnatal period had been studied.
Wistar rats received total daily administrations of 10 or 100 mg/kg bw caffeine by gavage, either as a single dose or as four doses every three hours, on days 6–20 of gestation. While a dose-related decrease in fetal weight and an increase in the delay in ossification were observed with both modes of administration, the major malformation, ectrodactyly, was observed only in the group given 100 mg/kg bw as a single dose (Smith et al., 1987).
When CD-COBS rats were administered 80 mg/kg bw caffeine orally as a single dose or as four doses every three hours on day 12 of gestation, the peak blood levels of caffeine and the area under the blood concentration-time curve were doubled with the single-dose as compared to multiple-dose regime (Jiritano et al., 1985). [The Working Group noted that this finding is consistent with that of the preceding study.]
Sprague-Dawley rats were administered 5–75 mg/kg bw caffeine daily by gavage on days 3–19 of gestation and their offspring were observed for behavioural and developmental effects for nine weeks after birth. Dose-related developmental effects included delayed incisor eruption, delayed vaginal opening and decreased body weight. Active avoidance behaviour was also significantly decreased with the highest doses of caffeine (West et al., 1986).
Many other developmental neurotoxicology studies, mostly in rats, have evaluated the effect of prenatal administration of caffeine on behavioural and neurochemical measures in neonates. These studies were reviewed by Sobotka et al. (1979). The effects are not consistent across studies: thus, caffeine may cause subtle changes in discrete neuronal subsystems but is not a neurotoxicant in the sense of disrupting primary neuronal systems.
(iv) Genetic and related effects
The genetic and related effects of caffeine have been reviewed (Bateman, 1969; Adler, 1970; Fishbein et al., 1970; Anon., 1973; Mulvihill, 1973; Kihlman, 1974; Thayer & Palm, 1975; von Kreybig & Czok, 1976; Kihlman, 1977; Timson, 1977; Legator & Zimmering, 1979; Lachance, 1982; Tarka, 1982; Haynes & Collins, 1984; Dalvi, 1986; Grice, 1987; Rosenkranz & Ennever, 1987), as have its antimutagenic effects (Clarke & Shankel, 1975).
The results described in this section are listed in Table 13 on p. 336, with the evaluation of the Working Group, as positive, negative or inconclusive, as defined in the footnotes. The results are tabulated separately for the presence and absence of an exogenous metabolic system. The lowest effective dose (LED), in the case of positive results, or the highest ineffective dose (HID), in the case of negative results, are shown, together with the appropriate reference. The studies are summarized briefly below.
Table 13.
Genetic and related effects of caffeine.
Effects on DNA structure and DNA synthesis: Caffeine interacts in different ways with DNA structure and metabolism. There is some evidence of intercalation of caffeine in double-stranded DNA (Richardson et al., 1981; Tornaletti et al., 1989). Caffeine impairs the helical structure of DNA (Ts’o et al., 1962), causes a slight increase in the rate of its elongation (Bowden et al., 1979) and lowers its melting-point. There may be local unwinding of DNA, as suggested by susceptibility to single-strand-specific nuclease digestion (Chetsanga et al., 1976).
It has been known since 1964 that caffeine interacts with DNA primarily at single-stranded regions; however, in the initial studies very high concentrations of methylxanthine were used (Byfield et al., 1981). In ultraviolet-irradiated DNA treated with low concentrations of caffeine, the caffeine molecules bind to the DNA near the region of the radiation-induced conformational changes. Caffeine binds to single-stranded (denatured) DNA regions, and it seems to bind preferentially to A-T-rich regions. This might be due to costacking, particularly with adenine (Kihlman, 1977).
Co-incubation of caffeine with single-strand-specific endonuclease induced some breakage, whereas no breakage occurred when DNA was incubated with either caffeine or endonuclease alone (Sleigh & Grigg, 1974; Chetsanga et al., 1976). Denatured (single-stranded) DNA has a higher affinity for caffeine than does native (double-stranded) DNA (Ts’o & Lu, 1964). In human lymphocytes, 3H-labelled caffeine did not bind in situ to chromosome preparations after heat or alkali denaturation (Brøgger, 1974).
There are many studies on the effects of caffeine on enzymes involved in DNA metabolism and on nucleotide pools. The RNA-dependent DNA polymerase activity of murine and avian oncogenic viruses was inhibited by caffeine (Srinivasan et al., 1979). There were conflicting reports of inhibition of Escherichia coli polymerase I polymerizing activity (Solberg et al., 1978; Balachandran & Srinivasan, 1982); however, caffeine inhibited nuclease activities of E. coli DNA polymerase (Solberg et al., 1978). DNA polymerase activity in human embryonic lung cells was inhibited by caffeine (Wragg et al., 1967). Caffeine inhibited three different exonucleases of E. coli (Roulland-Dussoix, 1967), thymidine kinase (at high concentrations; Sandlie et al., 1980) and some, but not all, of the purine nucleoside phosphorylases of both the ribose and deoxyribose series (Koch & Lamont, 1956); thymidine phosphorylase was not affected (Sandlie et al., 1980). Effects on nucleotide pools are discussed below (p. 335).
In E. coli, caffeine did not behave like a purine analogue in the purine biosynthesis pathway (Delvaux & Devoret, 1969). The effects of caffeine on DNA synthesis differed according to the assay system used. Caffeine did not inhibit DNA synthesis in vitro (Grigg, 1968), but DNA synthesis was inhibited in cell-free extracts of cultured human embryonic lung cells (Wragg et al., 1967), in Paramoecium aurelia (Smith-Sonneborn, 1974) and in Saccharomyces cerevisiae (Tsuboi & Yanagishima, 1975), but not in Tetrahymena pyriformis (Lakhanisky et al., 1981).
In Drosophila melanogaster larvae, caffeine strongly inhibited semi-conservative DNA synthesis but had no effect on repair replication (Boyd & Presley, 1974). Post-replication repair-deficient mutants were affected only minimally by caffeine (Boyd & Shaw, 1982).
In a study with partially hepatectomized mice in vivo, caffeine (given intraperitonealy at 50 mg/kg per day for four days) depressed the synthesis of DNA (as measured by 3H-thymidine incorporation) but not of RNA in the liver (Mitznegg et al., 1971). 3H-Thymidine incorporation into DNA was also depressed in mouse bone-marrow cells (Singh et al., 1984).
Caffeine increased the number of replication sites in the DNA of Chinese hamster V79 cells and in HeLa cells (Painter, 1980) and slightly increased the rate of DNA elongation in V79 cells, which qualitatively and reproducibly correlated with an increased cloning efficiency (Bowden et al., 1979). The pattern of condensation in DNA in chicken fibroblasts was changed by caffeine (Ghosh & Ghosh, 1972), which also partially inhibited cell-cycle progression from G1 through to M phase in mouse S–180 ascites cells (Boynton et al., 1974). Caffeine inhibited DNA synthesis in Chinese hamster CHO-K1 cells (Waldren & Patterson, 1979), V79 cells, mouse lymphoma L5178Y cells and mouse LS929 cells (Lehmann, 1973). An important element in this inhibition of DNA synthesis is reduced precursor uptake by cells: there were large reductions in the uptake of uridine and thymidine in Chinese hamster ovary (CHO)-K1 cells (Waldren, 1973) and that of thymidine in L5178Y-UK cells (Lehmann & Kirk-Bell, 1974). In CHO-K1 cells treated with caffeine, one complete cell cycle was possible, but in the second cycle there was a block near the G2/S interface (Waldren, 1973).
In a test for differential cytotoxicity using wild-type and DNA repair-deficient strains of CHO cells, it was concluded that caffeine was probably not a DNA damaging agent, because no differential retardation of growth was observed (Hoy et al., 1984).
In human HeLa cells, caffeine inhibited RNA but not DNA synthesis (Kuhlmann et al., 1968). 14C-Caffeine was not incorporated into the DNA of human lymphocytes (Brøgger, 1974), but it reduced the size of DNA segments synthesized by excision repair-defective xeroderma cells (Buhl & Regan, 1974).
Prokaryotes: Evidence of caffeine-induced DNA damage was observed in the Bacillus subtilis rec assay (weak responses) and in the E. coli repair test.
The mutagenic activity of caffeine was first observed in a streptomycin-dependent strain of E. coli in the 1940s (see Table 13); however, other studies in E. coli gave positive and negative results. Its mutagenic activity was confirmed using phage-resistance and a reverse mutation assay. Caffeine was shown to induce frameshift mutations (Clarke & Wade, 1975). In most cases, the mutation rate was directly proportional to the growth rate (Kubitschek & Bendigkeit, 1964), and this is consistent with the hypothesis that a mutational event occurs as a mistake during DNA replication (Webb, 1970). Caffeine may also act as an antimutagen in E. coli (Grigg & Stuckey, 1966), perhaps by reducing growth rate (Barfknecht & Shankel, 1975).
Caffeine was consistently nonmutagenic in many studies in all the Salmonella typhimurium his reversion tester strains and in S. typhimurium forward mutation assays. It was, however, mutagenic to Xanthomonas phaseoli, Klebsiella pneumoniae and Bacillus subtilis.
Lower eukaryotes (including fungi): Caffeine was generally mutagenic in algae (Plectonema boryanum) and fungi (Physarum polycephalum, Dictyostelium discoideum, Ophiostoma multiannulatum). Some negative findings were observed in fungi (Ophiostoma reverse mutation) and yeast (Schizosaccharomyces pombe).
Caffeine induced aneuploidy (monosomics) in Saccharomyces cerevisiae.
Studies in the yeast S. pombe revealed a significant decrease in the frequency of meiotic recombination and an increase in that of mitotic gene conversion between closely linked heteroallelic markers. It was suggested that the reduction of meiotic crossing-over may be caused by an interaction of caffeine with DNA, which inhibits DNA degradation (Ahmad & Leupold, 1973; Loprieno et al., 1974). As a result of this interaction, more stable pairing might occur at the level of mismatched bases, thereby generating an increase in mitotic gene conversion.
Plants: Caffeine increased the rate of point mutations in plants (Glycine max). It also induced chromosomal aberrations in many studies in plants (e.g., Allium, Hordeum and Vicia species), with only a few exceptions. The incidence of aberrations was modified by ATP (Kihlman et al., 1971a) and low temperature (Osiecka, 1976). Sister chromatid exchange was induced in Vicia faba, and mitotic recombination was induced in a number of studies in plants (e.g., Glycine max and Nicotiana tabacum).
Insects: Results of tests for sex-linked recessive lethal mutation in Drosophila melanogaster were equivocal, but chromosomal aberrations were induced when the exposed cells were in G2 or early mitosis, and there was evidence of recombinogenic effects. Predominantly positive responses were induced in tests for aneuploidy in D. melanogaster, although the frequencies were low. Dominant lethal responses were not observed in Bombyx mori.
Mammalian cells in vitro: DNA strand breakage was not induced by caffeine.
In V79 cells, mutation was not induced at the hprt locus, and there was no increase in the frequency of ouabain-resistant mutants. Also, caffeine failed to induce forward mutations either to auxotrophy at a variety of loci in CHO-K1 cells or at the tk locus in mouse lymphoma L5178Y cells. Caffeine has been reported to be antimutagenic to V79 cells, in which it reduces the fractions of both induced and spontaneous mutations.
Sister chromatid exchange was induced in some studies but not in others. Its induction may well be related to an inhibition of the poly(ADP-ribose) polymerase; this inhibition could delay the rejoining of DNA strand breaks induced by bromodeoxyuridine (Natarajan et al., 1981). Inhibition of this enzyme is associated with the induction of sister chromatid exchange (Levi et al., 1978; Morgan & Cleaver, 1982).
Micronuclei have been induced by caffeine in a cell line and in cultured mouse preimplantation embryos. The sensitivity of different cell lines to the induction of chromosomal aberrations by caffeine clearly varies widely. When treated with caffeine, CHO cells responded with large increases in the frequency of chromosomal aberrations that were dependent upon treatment during S-phase (Kihlman et al., 1971a,b; Kihlman, 1977). In mice deficient in folate, caffeine strongly increased the frequency of micronucleated cells (MacGregor, 1990).
Caffeine enhanced the frequency of cell transformation in several virus-induced systems but not in an assay for colony morphology in primary Syrian hamster embryo cells.
Human cells in vitro: The growth of HeLa cells was inhibited by concentrations of caffeine above 300 µg/ml (Ostertag et al., 1965); exposure of these cells for 2 h to 1% caffeine had virtually no effect on cell cycle time (Kuhlmann et al., 1968).
Caffeine did not induce unscheduled DNA synthesis or hprt locus mutations in human cells.
Caffeine weakly induced sister chromatid exchange in most of eight studies with human leukocytes and in all three published studies with leukocytes or lymphoblastoid cells from patients with xeroderma pigmentosum. Dose-dependent increases were obtained in only two of the studies (Ishii & Bender, 1978; Guglielmi et al., 1982).
In contrast, numerous reports have described the induction of chromosomal aberrations in human leukocytes and in HeLa cell lines. In cultured human lymphocytes from people with the heritable fragility condition, caffeine enhanced the expression of fragile sites (Ledbetter et al., 1986; Smeets et al., 1989).
Mammals in vivo: In a large number of studies in mammals in vivo, caffeine usually failed to induce significant responses. While single-strand breaks were induced in mouse liver and kidney, there was no significant effect in host-mediated assays (incubation of bacteria in the intraperitoneal cavity or in-vitro testing against bacteria from the urine of dosed rats), in an assay for specific locus in a mouse germ-line cell or in a mouse spot test.
Variable responses were obtained, however, with respect to sister chromatid exchange: of seven reports, two gave negative results, one gave a weak positive result and four a significant positive response.
In a large number of studies on the possible clastogenic effects of caffeine, almost uniformly negative responses were obtained in tests for micronuclei, bone-marrow metaphases and dominant lethal mutation. Negative results were also seen in a translocation test, and chromosomal aberrations were not induced in metaphase-I cells of mouse spermatogenesis. In addition, no sperm abnormality was induced in mice. Among this wealth of negative data, three significant positive responses were seen in the micronucleus test; in each case, the doses were in the toxic range.
Effects of methylxanthines on relevant targets other than DNA: In this section, we consider the effects of the methylxanthines, caffeine, theophylline and theobromine, on non-DNA targets but which potentially lead indirectly to DNA damage, mutation and modification of the activities of xenobiotics (including carcinogens) co-administered with methylxanthines. These aspects have been reviewed (Kihlman, 1977; Roberts, 1978; Byfield et al., 1981; Haynes & Collins, 1984; Roberts, 1984; Althaus & Richter, 1987; Boothman et al., 1988).
Non-DNA targets that are important to the genotoxic and related effects of methylxanthines are (i) cytochrome P450s (see p. 322 et seq.), (ii) cAMP metabolism, (iii) DNA metabolism, chromatin structure and function and (iv) nucleotide pools.
(1) cAMP metabolism
It is well established that methylxanthines can inhibit the phosphodiesterase involved in the degradation of cyclic nucleotides (Leonard et al., 1987), i.e., the intracellular messengers that control a wide variety of phenomena not related to survival per se. The majority of the studies were performed in vitro with caffeine concentrations higher than levels encountered by humans in vivo.
In mouse B-16 melanoma cells, Kolb and Mansfield (1980) found that theophylline inhibited DNA synthesis, reduced cell growth rate, elevated intracellular cAMP levels and changed cell morphology. Since these effects are also caused by cAMP and its potentiators in other cell lines, the inhibition of DNA synthesis is assumed to be a secondary effect of the increased level of cAMP resulting from inhibition of cAMP phosphodiesterase by theophylline. cAMP is known to inhibit cell growth and the transport of metabolites; it also mediates contact inhibition, the formation of cytoskeletal structures and increases cell adhesiveness (Rajaraman & Faulkner, 1984). Therefore, the reduced uptake of 3H-thymidine by L5178Y cells observed by Lehmann and Kirk-Bell (1974) may also, in part, be mediated by the increased cAMP concentration (Kolb & Mansfield, 1980).
cAMP does not, however, mimic the effects of caffeine on chromosomal structure nor on the gap filling process in radiation-damaged DNA. Furthermore, in some plant cells in which methylxanthines induce chromosome damage, the presence of cAMP is equivocal (Kihlman, 1977). Therefore, the effects of caffeine on cAMP levels appear not to be involved in the induction of chromosomal aberrations.
(2) DNA metabolism, chromatin structure and function
The effects of methylxanthines in cells treated with mutagenic agents can be summarized as follows (Roberts, 1984):
- (i) reversal of agent-induced depression of DNA synthesis;
- (ii) reversal of agent-induced inhibition of replicon initiation;
- (iii) decrease in size of replicons (also in the absence of DNA damage);
- (iv) inhibition of elongation of nascent DNA (to high-molecular-weight, template-sized DNA);
- (v) time-dependent incision of template DNA;
- (vi) time-dependent formation of DNA double-strand breaks;
- (vii) inhibition of excision of base damage;
- (viii) induction of protein synthesis; and
- (ix) prevention of S phase delay and G2 arrest (induction of premature mitosis).
These aspects are considered together because they appear to result from two interrelated actions of methylxanthines affecting chromatin: interaction with single-stranded DNA and inhibition of poly(ADP-ribosyl)ation reactions.
Methylxanthines, in particular caffeine, interact with DNA primarily at single-stranded regions. In living cells, there is only indirect evidence for such interaction (Althaus & Richter, 1987). The finding that the production of chromosomal aberrations by methylxanthines in bean root tips is strongly dependent on temperature, with a sharp maximum around 12°C, led to the suggestion that chromosomal aberrations may be the result of an influence of the methylated oxypurines on macromolecular hydration structures (Kihlman, 1977).
Inhibition of poly(ADP-ribose)polymerase was determined in nucleotide-permeable human lymphocytes following three days of stimulation with 2 μg/ml L-phytohaemagglutinin: theophylline (2 mM) gave 89% inhibition, theobromine (1 mM), 81%, and caffeine (2 mM), 35% (Althaus & Richter, 1987). This is an important finding because poly(ADP-ribosyl)ation reactions are distributed ubiquitously among higher eukaryotes and have been demonstrated in a number of plants and lower eukaryotes. Various lines of evidence indicate an involvement of poly(ADP-ribosyl)ation in the normal cell cycle of mammalian cells and, in particular, in the molecular events occurring during S phase. Distinct changes in the levels of biosynthetic activity of poly(ADP-ribose) were observed in cellular differentiation processes. The presence of poly(ADP-ribosyl)ation in yeast is controversial. No activity has so far been found in prokaryotic organisms. An inhibition of poly(ADP-ribosyl)ation reactions by methylxanthines may result in genetic effects and in the modulation of genetic effects induced by other agents (ionizing radiation, ultraviolet light, and mutagenic and carcinogenic chemicals), because these reactions are involved in all major chromatin functions, i.e., DNA repair, DNA replication and transcriptional activity. They influence the local organization of chromatin and, in particular, the architecture of active chromatin domains as a consequence of altered protein interactions.
Important acceptor proteins for poly(ADP-ribose) are histone H2B and histone H1, which are involved in the nucleosomal organization of chromatin and of polynucleosome structures, respectively. The production of DNA strand breaks, either directly (ionizing radiation) or enzymatically in the process of DNA-excision repair, is required for the stimulation of poly(ADP-ribose) biosynthesis. In excision repair, several steps have been shown to be affected by poly(ADP-ribose)polymerase inhibitors: incision and ligation are inhibited, excision is reduced and repair synthesis is usually stimulated. Inhibition by methylxanthines of poly(ADP-ribose) synthesis usually results in reduced repair. Many unrepaired lesions are lethal, and reduced survival of damaged cells is observed.
There is a positive correlation between the sister chromatid exchange-inducing potential and the inhibitory effects of chemicals that reduce the activity of poly(ADP-ribose)polymerase. There is no concomitant increase in the frequency of chromosomal aberrations or point mutations (e.g., hprt mutants). In contrast, in cells with damaged DNA, ADP-ribosylation inhibitors significantly increased the frequency of chromosomal aberrations induced by alkylating agents and other types of chemical mutagens, and also by ultraviolet or ionizing radiation, and raised the incidence of hprt mutants (which can result from deletions) but not of ouabain-resistant mutants (resulting mostly from amino acid substitutions). Overall, an altered poly(ADP-ribose) metabolism can have specific effects on genetic phenomena such as DNA excision repair (Roberts, 1978), clastogenicity and mutagenicity (Roberts, 1984; Althaus & Richter, 1987), but also on neoplastic transformation (Roberts, 1984; Boothman et al., 1988). In the last case, controversial results have been reported.
(3) Nucleotide pools
It has been established that genetic effects can be produced not only by radiation and chemical attack upon DNA, but also by disturbances in deoxyribonucleotide precursor pools. Some studies indicate that the purine analogue, caffeine, may affect DNA precursor metabolism (Haynes & Collins, 1984). Caffeine is known to inhibit enzymes of purine metabolism and may thereby alter the normal base ratio in the DNA precursor pool, thus causing errors in pairing. Caffeine doses enhance the killing action of ultraviolet light. It inhibits both de-novo synthesis and the utilization of exogenous purines in cultured CHO cells. Furthermore, caffeine inhibited incorporation of thymidine into DNA both in prokaryotic and eukaryotic cells. In E. coli, it has been suggested that this inhibition could be caused by a caffeine-induced inhibition of thymidine kinase or, more likely, an effect of caffeine on the DNA synthesis process itself. It was shown that, although thymidine kinase is inhibited by caffeine in E. coli cells, intracellular concentrations of thymidine triphosphate which one would consequently expect to decrease, actually increased significantly. Thus, the major effect of caffeine on the nucleotide pool appears to be the result of inhibition of processes that involve thymidine triphosphate. In these experiments, intracellular concentrations of the other nucleoside triphosphate pools were only slightly increased by caffeine. The finding that chronic exposure to caffeine led to sister chromatid exchange in human peripheral blood lymphocytes (Guglielmi et al., 1982) was interpreted to be a result of inhibition of DNA synthesis brought about by inhibition of de-novo synthesis of endogenous purines and also the transport and use of exogenous purines.
(b) Humans
(i) Absorption, distribution, metabolism and excretion
Caffeine absorption from the gastrointestinal tract is rapid, virtually complete and directly dependent on pH (Chvasta & Cooke, 1971; Marks & Kelly, 1973; Robertson et al., 1978; Bonati et al., 1982; Blanchard & Sawers, 1983a,b). Plasma concentration curves following oral and intravenous doses were superimposable, suggesting that there is no pronounced first-pass effect (Axelrod & Reichenthal, 1953); after oral doses of 5–8 mg/kg bw, peak plasma concentrations of 8–10 μg/ml were observed (Arnaud & Welsch, 1980b, 1982; Bonati et al., 1982; Blanchard & Sawers, 1983a,b; Arnaud, 1987). After oral ingestion, the time to reach peak plasma concentration exhibits wide variations, ranging from 15 to 120 min (Robertson et al., 1978; Bonati et al., 1982; Arnaud, 1987). These variations can be explained by the effect of gastric emptying (Chvasta & Cooke, 1971; Arnaud, 1987) and also by the presence of dietary constituents (Arnaud, 1987).
After absorption, caffeine is rapidly and uniformly distributed into body fluids (Bonati & Garattini, 1984). The volume of distribution of caffeine in man ranges from 0.5 to 0.8 l/kg bw, but the value most often reported is close to 0.7 l/kg bw (Arnaud, 1987). In newborns, the levels of caffeine in plasma and cerebrospinal fluid are virtually identical (Turmen et al., 1979; Somani et al., 1980). In-vivo and in-vitro studies have shown that caffeine is bound at 10–35% to plasma proteins, mainly albumins, over a wide range of concentrations (1–100 µg/ml) (Bonati & Garattini, 1984; Yesair et al. 1984). The binding capacity of caffeine to breast milk proteins is about 3.2% (Tyrala & Dodson, 1979).
Caffeine is eliminated by apparent first-order kinetics, described by a one-compartment open model system. A study of a limited number of patients with a small range of doses (≤10 mg/kg bw) excluded the existence of dose-dependent kinetics in man at the levels at which people are normally exposed to caffeine (Bonati et al., 1982).
The half-time of caffeine decreases gradually after birth and reaches adult values (2.5–4.5 h) at about the age of six months (Aldridge et al., 1979; Aranda et al., 1979a,b; Parsons & Neims, 1981; Gorodischer & Karplus, 1982). A serum clearance of 31.5 ml/kg bw per h in 1–2.5-month-old infants increased to a mean maximum value of 331.7 ml/kg bw per h in 5–6-month-old infants, while values of 155 and 94 ml/kg bw per h were observed in adult smokers and nonsmokers, respectively (Aranda et al., 1979a). No significant difference in the elimination of caffeine in young and elderly subjects has been found, although a slight decrease in plasma caffeine binding was observed in the older group (Blanchard & Sawers, 1983b).
Caffeine clearance is stimulated by smoking (Parsons & Neims, 1978; Wietholtz et al., 1981; Kotake et al., 1982; May et al., 1982; Arnaud, 1987; Joeres et al., 1988); after stopping smoking for only three or four days, the rate of caffeine metabolism is substantially slower (Brown et al., 1988; Murphy et al., 1988). The metabolic disposition or volume of distribution of caffeine does not differ between men and women (Callahan et al., 1983; Arnaud, 1987). In women, the use of oral contraceptives was shown to double the half-time of caffeine (Patwardhan et al., 1980; Callahan et al., 1983). A gradual prolongation of the half-time was also shown during pregnancy (Neims et al., 1979; Aldridge et al., 1981; Knutti et al., 1981, 1982; Parsons & Pelletier, 1982; Brazier et al., 1983), and caffeine clearance had increased by more than three fold at 2–12 weeks postpartum (Parsons & Pelletier, 1982).
Caffeine concentrations in human and fetal gonads were similar to those in plasma (Goldstein & Warren, 1962). A small percentage (0.5–4%) of an ingested dose of caffeine is excreted unchanged in urine (Arnaud, 1987). Caffeine is also excreted in bile (Arnaud, 1987) and is found in saliva (Cook et al., 1976; Parsons & Neims, 1978; Newton et al., 1981), semen (Beach et al., 1982, 1984) and breast milk (Tyrala & Dodson, 1979; Findlay et al., 1981; Bailey et al., 1982; Ryu, 1985); it was also detected in umbilical cord blood (Parsons et al., 1976; van’t Hoff, 1982). As salivary concentrations correspond to 65–85% of plasma concentrations, they can be used to predict serum concentrations (Khanna et al., 1980; Callahan et al., 1982). An average milk to serum concentration ratio of 0.52 was observed (Tyrala & Dodson, 1979).
The metabolism of caffeine is the rate-limiting factor for its plasma clearance (Arnaud, 1987). It is transformed by hepatic microsomal enzymes (Grant et al., 1987; Berthou et al., 1989), and no significant metabolism occurs in other organs (Arnaud, 1987). The initial major step in caffeine biotransformation in humans is selective catalysis by cytochrome P450PA (P450IA2) in human liver microsomes, which is also responsible for the N-oxidation of aryl amines (Butler et al. 1989). The major role of the liver is demonstrated by the impaired clearance of caffeine in subjects with liver disease, in whom serum half-times of 60–168 h were reported (Statland et al., 1976; Desmond et al., 1980; Statland & Demas, 1980; Renner et al., 1984). Many drug interactions have been reported to lead to impaired caffeine elimination, explained by competitive inhibition at the enzymatic level (Reynolds, 1989). Allopurinol causes dose-dependent inhibition of the conversion of 1-methylxanthine to 1-methyluric acid (Grygiel et al., 1979; Grant et al., 1986). Alcohol has been shown to impair caffeine elimination (Mitchell et al., 1983; George et al., 1986). Ingestion of 480 mg/day of caffeine for one week by healthy male volunteers failed to alter its pharmacokinetics (George et al., 1986).
Caffeine metabolism has been reviewed extensively (Arnaud, 1984, 1987) (see Figure 2). After oral administration of caffeine, plasma concentrations of theobromine and theophylline showed a small and similar increase, while a ten-fold higher paraxanthine concentration was observed. In most of the subjects studied, caffeine plasma concentrations decreased more rapidly than those of paraxanthine, so that paraxanthine concentrations became higher than those of caffeine from 8 to 10 h after administration (Bonati et al., 1982). The amount of urinary caffeine metabolites depends on the rate of each biotransformation step, the body distribution of metabolites, their plasma concentrations and their renal excretion (Arnaud, 1987). All the metabolic transformations shown in Figure 2 include multiple and separate pathways with demethylation, C-8 oxidation and perhaps, associated with this transformation, the formation of uracil derivatives. In contrast to these biotransformations, the C-8 oxidation of 1-methylxanthine into 1-methyluric acid is not a microsomal transformation but was shown to be performed by xanthine oxidase (Bergmann & Dikstein, 1956; Grygiel et al., 1979; Grant et al., 1986; Arnaud, 1987).
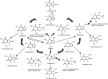
Fig. 2.
Metabolism of caffeine in humansa
Although in women who used oral contraceptives, the half-time of caffeine was doubled, only minor changes in the proportion of the formed metabolites have been reported (Callahan et al., 1983). Compared to controls, pregnant women produced smaller amounts of 1-methylxanthine and 1-methyluric acid (Scott et al., 1986).
The most important metabolic pathway in humans is demethylation of caffeine (95%), only 5% giving trimethyl derivatives, whereas in rodents 40% trimethyl derivatives are found (Arnaud & Welsch, 1980b; Arnaud, 1987). Therefore, collection of labelled carbon dioxide after demethylation of [14C- or 13C-methyl]-caffeine constitutes a breath test used in clinical studies to detect impaired liver function (Wietholtz et al., 1981; Kotake et al., 1982; Renner et al., 1984; Arnaud, 1987).
The 5-acetylamino-6-formylamino-3-methyluracil metabolite of caffeine is found only in humans; paraxanthine was shown to be its precursor (Grant et al., 1983a; Arnaud, 1984). Although its estimated rate of production (3–35%) approximates those of 1-methylxanthine (18%) and 1-methyluric acid (15%) (Callahan et al., 1982; Yesair et al., 1984), however, its metabolic formation is not yet understood. The variability in the production and excretion rates of acetylated urinary metabolites (Callahan et al., 1982; Tang et al., 1983) was related to acetylation polymorphism (Grant et al., 1983b). The demonstration that the general population could be divided into fast and slow acetylators (Evans & White, 1964) explains some of the variability observed in caffeine metabolism. A simple marker for acetylator status in man is the ratio 5-acetylamino-6-formylamino-3-methyl-uracil:1-methylxanthine in urine (El-Yazigi et al., 1989). The ratios of the urinary concentrations of 1-methyluric acid:1-methylxanthine, of 1,7-dimethyluric acid:1,7-dimethylxanthine and of 5-acetylamino-6-formylamino-3-methyluracil plus 1-methyluric acid plus 1-methylxanthine:1,7-dimethylxanthine represent indices of xanthine oxidase, 8-hydroxylation and 7-demethylation activities, respectively (Kalow, 1984).
After oral administration of labelled caffeine, 2–5% was excreted in the faeces. The most important products identified were 1,7-dimethyluric acid at 44%, 1-methyluric acid at 38%, 1,3-dimethyluric acid at 14%, 1,3,7-trimethyluric acid at 6% and caffeine at 2% of faecal radioactivity (Callahan et al., 1982).
(ii) Toxic effects
General toxicity: The toxicity of caffeine in humans has been reviewed (Lachance, 1982; Rall, 1985; Arnaud, 1987; Ashton, 1987; Leonard et al., 1987; Stavric, 1988).
At low doses (up to 2 µg/ml in blood), caffeine stimulates the central nervous system, and this effect is perceived by many caffeine users as beneficial. High blood concentration (10–30 µg/ml) of caffeine may produce restlessness, excitement, tremor, tinnitus, headache and insomnia (Lachance, 1982; Ashton, 1987; Stavric, 1988).
Caffeine can induce alterations in mood and sleep patterns, increase urine production and gastric acid secretion, alter myocardial function, induce hypertension and arrhythmia, and increase plasma catecholamine levels and plasma renin activity, especially when administered to non-users or recent abstainers (Leonard et al., 1987; Stavric, 1988). Excessive consumption of caffeine may lead to an anxiety neurosis known as ‘caffeinism’. Similar symptoms have been described after withdrawal of caffeine (Greden, 1979; Griffiths & Woodson, 1988).
Acute toxicity due to caffeine is not very common, although some adverse effects (e.g., gastric symptoms, insomnia, diuresis) have been observed as a result of overdoses (Lachance, 1982; Rall, 1985; Stavric, 1988). Caffeine poisoning can be especially hazardous to persons with impaired liver function. Patients with liver cirrhosis may accumulate up to 40 times more caffeine and its metabolites than healthy controls (Wahlländer et al., 1985).
The effects of coffee and tea on plasma lipid levels and on morbidity and mortality from cardiovascular disease are discussed in detail in the respective monographs.
The effect of caffeine consumption (86.8% from coffee) on mortality from cardiovascular disease was evaluated in a cohort study of more than 10 000 hypertensive people from 14 communities in the USA over four years. No evidence was found to support an association between increased caffeine consumption and increased mortality due to cardiovascular disease (Martin et al., 1988).
Blood pressure: The effects of caffeine on blood pressure have been reviewed (Myers, 1988; Robertson & Curatolo, 1984).
In volunteers who abstained from caffeine-containing products, a bolus dose of 250 mg led to a 5–10% increase in both systolic and diastolic blood pressure for 1–3 h. Tolerance to this effect developed, however, when caffeine was given three times a day for seven days (Robertson et al., 1978, 1981; Arnaud, 1987). Increases in systolic and diastolic blood pressure (by 5.1 and 11.5 mm Hg, respectively) were observed in 10 volunteers who drank coffee corresponding to about 240 mg caffeine (Smits et al., 1986). In another study, daily use of decaffeinated coffee (40 mg caffeine) instead of five cups of regular coffee (445 mg caffeine) for six weeks led to a small but significant decrease in systolic (by 1.5 mm Hg) and diastolic (by 1.0 mm Hg) blood pressure in 45 healthy volunteers (van Dusseldorp et al., 1989).
Several epidemiological studies have investigated the association between coffee drinking and blood pressure. One showed that only systolic blood pressure was related to coffee intake, and the overall increase before adjustment for other variables was 2.5 mm Hg (Lang et al., 1983). In another study (Birkett & Logan, 1988) caffeine intake was positively related to an increase in diastolic blood pressure, but the effect was small — less than 1 mm Hg at usual caffeine intake. A longitudinal study with more than 51 000 participants found no relationship between either systolic or diastolic blood pressure and the number of cups of coffee consumed (Bertrand et al., 1978). A significant decrease in blood pressure with increased coffee consumption was found in an epidemiological investigation involving 500 persons (Periti et al., 1987).
The available data indicate that consumption of caffeine in moderate amounts does not cause a persistent increase in blood pressure in normotensive subjects. Caffeine can, however, acutely raise blood pressure in non-users of caffeine-containing beverages, but after one to four days of regular consumption a tolerance develops, with blood pressure returning to previous levels.
Cardiac arrhythmias: Caffeine and caffeine-containing beverages have long been implicated in the etiology of cardiac arrhythmias, but only a few well-designed studies have been carried out in humans (for a review, see Dobmeyer et al., 1983). An association between premature ventricular beats and consumption of large amounts of coffee or tea was found in a large cross-sectional study reported by Prineas et al. (1980).
Contrary to several earlier authors (for reviews, see Dobmeyer et al., 1983; Robertson & Curatolo, 1984), Myers et al. (1987) found no increase in the severity or frequency of ventricular arrhythmia after intake of caffeine (300 mg) in a placebo-controlled study of patients with previous myocardial infarction. These results are in contrast to those of another study (Sutherland et al., 1985) in which 200 mg caffeine induced a considerable increase in the frequency of extrasystoles in subjects with a high incidence of spontaneous ventricular ectopic beats. Thus, the traditional clinical view that caffeine induces cardiac arrhythmias in humans still remains open, both in healthy subjects and in patients with existing heart disease.
Benign breast disease:
(1) Case series and intervention trials: A clinical association between fibrocystic breast disease and caffeine consumption was suggested in 1979, when Minton et al. (1979a,b) reported differences in the levels of cAMP and cGMP in normal, fibrotic, fibroadenomatous, fibrocystic and carcinomatous breast tissues. They also found an improvement in signs of the disease among women who eliminated methylxanthines from their diet. Similar findings were reported in a subsequent study by the same group (Minton et al., 1981). Several concerns about the studies have been raised. They were not randomized, information on the extent of disease at baseline was not always provided, caffeine consumption and the course of the disease were not monitored during the study, and outcome was ascertained by people who were aware of the caffeine consumption status of the subject.
Several clinical case series were subsequently studied. Brooks et al. (1981) found an improvement in signs of fibrocystic breast disease after restricting methylxanthine consumption. Heyden and Muhlbaier (1984; reported again by Heyden & Fodor, 1986) found that the mean change in total methylxanthine consumption in women who experienced a total or transient disappearance of nodules was similar to that in women who did not experience such changes. Hindi-Alexander et al. (1985) found that the severity of fibrocystic breast disease was greater with greater intake of caffeine, theophylline and total methylxanthines. Three randomized studies were conducted: Ernster et al. (1982) found a reduction in clinically palpable breast nodes in women on the methylxanthine-free diet but found no relation between changes in caffeine levels in breast fluid and degree of improvement in breast findings score. Parazzini et al. (1986) observed no difference among the methylxanthine-abstention groups in respect of change in size, clinical characteristics or mean scores at follow-up. Data from Allen and Froberg (1987) did not support the hypothesis of decreasing nodularity in the caffeine-free group relative to that in the group with no dietary restriction group or in a placebo group.
[The Working Group noted that studies of case series and intervention trials were concerned mainly with the effect of caffeine consumption on the symptoms of benign breast disease; they have little, if any, bearing on the occurrence of this condition.]
(2) Etiological studies: Lawson et al. (1981) analysed data obtained from the Boston Collaborative Drug Surveillance Program (January-September 1972) and from a collaborative study conducted in the USA, Scotland and New Zealand (from 1977 to present). Coffee and tea drinking were grouped as ‘hot beverage consumption’. When cases of fibrocystic disease were compared to controls who did not drink coffee or tea, the relative risks (RRs) were 1.4, 1.5 and 1.3 for increasing levels of intake.
Marshall et al. (1982) analysed a series of patients admitted to the Roswell Park Memorial Institute, Buffalo, NY, USA, between 1957 and 1965. When data were analysed by number of cups per day, no relation was found between the occurrence of fibrocystic breast disease and coffee consumption (age-adjusted RR, 0.9 for more than three cups per day versus non-coffee drinker); a nonsignificant reduction in risk was associated with tea consumption (RR, 0.8 for more than three cups per day), and no relation was seen when results for the two beverages were combined.
Boyle et al. (1984) conducted a hospital-based case-control study in Connecticut, USA, from 1979 to 1981. The study showed a clear, significant dose-response relationship between the occurrence of fibrocystic breast disease and the amount of caffeine that had been consumed daily five years previously. When patients were compared with women who consumed fewer than 30 mg caffeine per day, the RRs were 1.5, 2.0 and 2.3 for those who consumed 31–250, 251–500 and > 500 mg per day (p < 0.0001 test for trend). The increase in risk was greater among women with those subtypes of benign breast disease that are thought to be most closely related to an increased risk for breast cancer. The data were based on patient recall of caffeine consumption.
Odenheimer et al. (1984) studied female twins from the Kaiser-Permanente Twin Registry (USA) in 1977–79. For the 90 pairs in which one twin had biopsy-confirmed benign breast disease, the RR was 1.6 (95% confidence interval (CI), 1.0–2.4) for each cup of coffee consumed per day. For the 48 pairs in which cases were determined by clinical examination, the RR was 4.2 (1.1–15.6) for different categories of consumption. Fifteen pairs were in both groups.
Lubin et al. (1984, 1985a) conducted a hospital-based case-control study in Israel between 1977 and 1980. No significant association between the occurrence of benign breast disease and coffee consumption was found: in comparison with surgical controls, the adjusted RRs were 1.0, 1.1, 0.95 and 0.80 for four categories of consumption (0, 1, 2–3, > 4 cups/day); compared to neighbourhood controls they were 1.0, 0.85, 0.83 and 0.70. No association between total methylxanthine intake and benign breast disease was found. Similarly, no association was found when women with different histological types or women with different degrees of ductal atypia were examined separately.
La Vecchia et al. (1985) conducted a hospital-based case-control study of benign breast disease in Milan, Italy, between 1981 and 1983. Categorizing coffee consumption in three levels (0, 1–2 and >3 cups/day), the RRs by multivariate analysis were 1.0, 3.0 (95% CI, 1.7–5.3) and 3.8 (2.2–6.7) compared to the inpatient controls, and 1.0, 1.4 (0.8–2.5) and 2.1 (1.2–3.8) compared to outpatient controls for all benign breast disease together. For benign tumours (85 cases), the RRs were 1.0, 2.3 (1.0–4.8) and 1.6 (0.7–3.5) compared to the inpatient controls, and 1.0, 1.0 (0.5–2.2) and 1.0 (0.4–2.1) compared to outpatient controls. For dysplasia (203 cases), the RRs were 1.0, 4.1 (2.0–8.4) and 6.4 (3.1–13.1) compared to inpatient controls and 1.0, 2.0 (1.0–4.1) and 3.7 (1.8–7.7) when compared to outpatient controls.
Schairer et al. (1986) conducted a case-control study on participants in the Breast Cancer Detection Demonstration Project in the USA. For all benign breast disease cases, the RRs were 1.0, 1.0 (95% CI, 0.8–1.2), 1.0 (0.8–1.2), 1.0 (0.7–1.2) and 1.1 (0.8–1.5) for five increasing levels of methylxanthine consumption (<125, 126–250, 251–500, 501–750, > 750 mg/day). When analysed specifically for cases of fibrocystic disease, cases of unknown type and cases of benign neoplasms, the RRs were all near unity. When cases with fibrocystic disease were subdivided by the presence of atypia, hyperplasia, sclerosing adenosis and cyst, RRs near unity were again observed.
Rohan et al. (1989) conducted a case-control study in Adelaide, Australia, between 1983 and 1985 which included both biopsy-confirmed controls (women whose biopsy did not show epithelial proliferation) and community controls. Total methylxanthine intake (caffeine, theobromine and theophylline) was not related to the risk of benign proliferative epithelial disorders: adjusted RRs for increased quintiles of intake (< 173.2, 173.2–270.0, 270.1–344.0, 344.1–429.3, > 429.3 mg/day) were 1.0, 0.8 (0.5–1.3), 0.8 (0.5–1.5), 0.8 (0.4–1.3) and 1.2 (0.7–2.1) using community controls, and 1.0, 0.7 (0.4–1.2), 0.7 (0.5–1.7), 0.7 (0.4–1.3) and 1.0 (0.6–1.9) using biopsy controls. Separate analyses for theophylline, theobromine and caffeine and for degree of atypia generally showed no association; however, theobromine intake was associated with an increased risk when biopsy controls were used as the comparison group. Total methylxanthine intake was associated with an increased risk of severe atypia when community controls were used.
[The Working Group noted that the positive association between coffee intake and benign breast disease found in some etiological studies could be due to the difference in the likelihood of disease detection between consumers and nonconsumers of methylxanthines. The one study in which biopsy-confirmed controls were used found no association.]
(iii) Effects on reproduction and prenatal toxicity
Malformations: A case-control study was carried out in Scotland by Nelson and Forfar (1971) of 458 mothers who gave birth to infants with congenital abnormalities and two control groups; the first was composed of the mothers of the next 500 normal babies delivered in the same maternity units as the patients, and the second were 411 mothers matched to the mothers of cases by age, parity and babies’ sex. Mothers were interviewed on the use of pharmaceuticals during pregnancy. This information was confirmed by doctors’ or pharmacists’ records; 11.3% of the reports were rejected due to lack of confirmation. Caffeine-containing drugs had been used by 2.4% of the mothers of cases and 1.5% of those of controls (nonsignificant). Few data are provided on losses and refusals. [The Working Group noted the restricted use of caffeine-containing drugs; other sources of caffeine were not considered.]
A large North American study followed 5378 women exposed during pregnancy to caffeine-containing drugs for possible malformations in their offspring (Heinonen, 1982). For all malformations considered together (n = 350), the RR was 0.98. For malformations at individual sites, the RRs ranged from 0.70 to 1.2 (nonsignificant). [The Working Group noted that non-medicinal sources of caffeine were not accounted for.]
Several studies have reported congenital malformations in relation to consumption of caffeine in beverages (Borlée et al., 1978; Linn et al., 1982; Rosenberg et al., 1982; Tohnai et al., 1984, Furuhashi et al., 1985). These are discussed in the monograph on coffee (pp. 104–106).
Rosenberg et al. (1982) estimated total caffeine intake from coffee, tea and cola for 2030 mothers of malformed infants and 712 controls. Total caffeine intake was related neither to all malformations nor to those at invididual sites.
Low birthweight and/or preterm birth: Studies relating coffee intake and low birthweight (Mau & Netter, 1974; van den Berg, 1977; Arnandova & Kaculov, 1978; Kuzma & Sokol, 1982; Linn et al., 1982; Berkowitz et al., 1982; Watkinson & Fried, 1985; Furuhashi et al., 1985; Martin & Bracken, 1987; Muñoz et al., 1988; Brooke et al., 1989; Caan & Goldhaber, 1989) are described on pp. 106–112. In several of these studies sources of caffeine other than coffee were considered.
Total caffeine intake, as determined from various sources including coffee, tea, cola and drugs, was positively associated with the proportion of low-birthweight babies after controlling for smoking and other potential confounders (RR, 4.6 for consumption of >300 mg/day versus no consumption; p < 0.001) (Martin & Bracken, 1987). A significant increase in the frequency of low birthweight was found by Caan and Goldhaber (1989) among women with heavy consumption of caffeine from coffee and cola as compared to women with none (odds ratio, 3.5; p < 0.05). [The Working Group was concerned that cola and coffee were considered to be equivalent sources of caffeine per drink.]
Effects on sperm and fertility: The addition of caffeine to human sperm increased sperm mobility (Homonnai et al., 1976; Traub et al., 1982; Aitken et al., 1983). Barkay et al. (1984) reported that women undergoing artificial insemination were twice as likely to become pregnant if their husband’s semen had been treated with caffeine than if it had not. Scanning electron microscopic examination of fresh semen showed no morphological change caused by in-vitro treatment with caffeine.
(iv) Genetic and related effects
Vogel et al. (1966) published results of an epidemiological study in which the sex ratios of children in German families were examined relative to the parents’ coffee-drinking habits. Using multiple regression techniques, no evidence was found that caffeine induced sex-linked recessive lethal mutations in women or sex-linked dominant mutations in men. The authors suggested that since the questionnaires on coffee drinking were completed by the children and their parents, there might be uncontrolled biases in the study. [The Working Group noted that others have pointed out that during the years in which many of the children involved were conceived, ‘coffee’ consumption may not necessarily have been synonymous with caffeine consumption because of wartime substitutions.]
The urine of coffee drinkers was not mutagenic to S. typhimurium TA100 or TA98 (Aeschbacher & Chappuis, 1981) (see the monograph on coffee, p. 112).
Drinking coffee or tea to result in a total caffeine intake corresponding to that in five cups of coffee per day [exact amount not stated] was associated by multiple regression analysis with a roughly two-fold higher frequency of both micronucleated reticulocytes and micronucleated mature erythrocytes in splenectomized but otherwise healthy individuals after adjustment for smoking. Drinking decaffeinated coffee was not associated with an increase in the number of micronucleated cells (Smith et al., 1990).
Cultured human lymphocytes from volunteers on a regime of 800 mg caffeine daily for four weeks, resulting in caffeine blood levels as high as 29.6 µg/ml after four weeks showed no significant increase in the frequency of chromosomal damage (Weinstein et al., 1972, 1973a,b, 1975).
In one study of caffeine ingestion during pregnancy, an elevated frequency of chromosomal anomalies was reported (Furuhashi et al., 1985). [The Working Group noted that the laboratory and the epidemiological methods, as well as the data, were so inadequately reported that the study was not suitable for evaluation.]
3.3. Epidemiological studies of cancer in humans
The epidemiological studies considered are those in which the effect of caffeine was examined as well as those in which the effects of methylxanthines (caffeine, theophylline and theobromine) were looked at.
(a) Cohort studies
(i) All sites
The effect of caffeine consumption on mortality from cancer at all sites among the 10 064 participants in the Hypertension Detection and Follow-up Program in the USA was examined after four years of follow-up (Martin et al., 1988). Exposure to caffeine was estimated on the basis of data obtained at interview concerning coffee and tea consumption and use of caffeine-containing medications; no data were collected on consumption of caffeine-containing soft drinks. The unadjusted RRs showed no association between caffeine consumption and mortality from cancer or any other cause, although in all of these analyses, the confidence intervals were very wide.
(ii) Breast cancer
Phelps and Phelps (1988) conducted an ecological study, which did not distinguish between tea and coffee consumption, and reported a correlation of 0.004 with breast cancer mortality ratios after adjusting for dietary fat intake.
(b) Case-control studies
(i) Breast
Case-control studies of breast cancer and methylxanthine consumption are summarized in Table 14.
Table 14.
Summary of results of case-control studies of breast cancer and methylxanthine consumption.
In the hospital-based case-control study of Lubin et al. (1984, 1985b), described on p. 145, methylxanthine intake was quantified on the basis of caffeine in coffee, tea and cola and theobromine in chocolate and in cocoa. No association was found, even after stratification for ethnic group, age or consumption of total, saturated or polyunsaturated fats.
In the study of Schairer et al. (1987), described on p. 146, on participants in a five-year screening programme for the detection of breast cancer in the USA, information on methylxanthine consumption was derived from information about consumption of brewed coffee, instant coffee, decaffeinated coffee, hot non-herbal tea, hot cocoa, iced tea, chocolate milk, cola soft drinks and diet cola drinks. The results for total caffeine alone were similar to those for total methylxanthines consumed, so only the latter were given. No association was found.
Rohan and McMichael (1988) conducted a population-based case-control study of breast cancer in Adelaide, South Australia. A total of 559 cases were diagnosed between April 1982 and July 1984; 451 were included in the study. Controls were matched to the age of the cases at diagnosis and selected at random from the electoral roll. A total of 648 individuals were approached in order to enroll the 451 controls included in this study. Methylxanthine intake was measured by means of a self-administered, quantitative food frequency questionnaire, and daily intake of caffeine was calculated in instant coffee, decaffeinated coffee, percolated coffee, drip coffee, tea, cocoa, chocolate drink, solid chocolate and cola; daily intake of theobromine in cocoa, chocolate drink and solid chocolate was also calculated. No increased risk for breast cancer was found in postmenopausal women in association with total caffeine and total methylxanthine intake. However, premenopausal women had an increase in risk at mid-third of intake (caffeine: RR, 2.0; 95% CI, 1.0–4.2; methylxanthines: 2.0, 1.0–4.1) and a smaller increase in risk at the upper third of intake (caffeine: 1.4; 0.6–3.0; methylxanthines: 1.6; 0.7–3.3). None of the RRs deviated markedly from unity and none was statistically significant. The patterns of RR provided little support for an interaction between methylxanthine and fat in determining breast cancer risk.
Iscovich et al. (1989) conducted a case-control study in La Plata, Argentina in 1984–85. Of the 153 cases of breast cancer identified, only three were not interviewed. Two matched controls were selected for each case: one from a hospital and the other from the neighbourhood of the case. Caffeine-containing beverages were one of 147 dietary items on the questionnaire, along with questions on demographic and socioeconomic characteristics, reproductive history and smoking. Multivariate analysis, adjusting for known risk factors, showed no effect of caffeine-containing beverages, analysed in quartiles, on breast cancer risk. Smoking was not adjusted for.
In the study by Pozner et al. (1986), described on p. 146, caffeine and coffee intake were examined in women with breast cancer to determine whether their consumption influenced cell differentiation in tumours. Caffeine consumption was calculated for coffee, instant coffee, decaffeinated coffee, tea, cola and cocoa. Women with moderately or well-differentiated tumours had higher intakes of all coffee, caffeinated coffee, decaffeinated coffee, cola and tea.
(ii) Urinary bladder
In a case-control study in Copenhagen, Denmark (Jensen et al., 1986), described on p. 126, a weak association was found between cancer of the urinary bladder and caffeine intake from coffee and tea. This association was significant in men (p < 0.05) after adjustment for consumption of beer and soft drinks, in addition to age and smoking.
4. Summary of Data Reported and Evaluation
4.1. Exposure data
Caffeine is a methylxanthine and occurs naturally in more than 60 plant species throughout the world. It is prepared on an industrial scale by methylation of theobromine.
Global per-caput consumption of caffeine from all sources was estimated to be 70 mg per day in 1981–82.
Caffeine is consumed in beverages such as coffee, tea and mate and in soft drinks to which caffeine is added. Coffee is the main source of dietary caffeine consumption. The caffeine content of beverages varies widely. Caffeine is also used in numerous prescription and non-prescription pharmaceutical preparations.
4.2. Experimental carcinogenicity data
Caffeine was tested for carcinogenicity in five studies in rats by oral administration. In two of these studies, no significant difference in the incidence of tumours at any site was found. The other three studies were found to be inadequate for evaluation.
Studies on oral and intraperitoneal administration of caffeine to mice were found to be inadequate for evaluation.
In one study, decaffeinated coffee to which caffeine was added was tested by oral administration to rats; overall, no increase in the incidence of tumours at any site was observed as compared to appropriate controls.
Administration of caffeine in combination with known carcinogens resulted in decreased incidences of lung tumours in mice treated with urethane, of mammary tumours in rats treated with diethylstilboestrol and of skin tumours in mice treated with either ultraviolet light or cigarette-smoke condensate. Caffeine did not influence the incidence of bladder tumours induced in rats by N-nitroso-N-butyl(4-hydroxybutyl)amine in three experiments or of pancreatic tumours induced in rats by 4-hydroxyaminoquinoline-1-oxide in another study.
4.3. Human carcinogenicity data
A cohort study with a short follow-up period showed no association between caffeine consumption and mortality from cancers at all sites, although there were few deaths on which to base an analysis.
Four case-control studies of breast cancer in which an attempt was made to measure methylxanthine intake showed no association. A slight increase in risk was seen in premenopausal women in one study, but in general the relative risks were below unity.
One case-control study of bladder cancer showed a weak association with caffeine consumption.
Caffeine and coffee consumption are highly correlated in most of the populations studied; thus, it is very difficult to separate the two exposures in epidemiological studies. It was therefore not possible to evaluate adequately the effect of caffeine per se.
4.4. Other relevant data
Caffeine intake from pharmaceutical sources has not been related to teratogenic effects in humans. High levels of either coffee or caffeine consumption were related to an increased frequency of low birthweight.
Quantitative and qualitative differences in the metabolism of caffeine are seen between humans and experimental animals.
On the basis of the available evidence, caffeine consumed in moderate amounts does not cause any persistent increase in blood pressure in normotensive subjects. Whether caffeine consumed in amounts present in coffee or tea causes cardiac arrhythmia in healthy subjects or in patients with heart disease remains an open question.
Caffeine has been shown to cause adverse reproductive and developmental effects in mice, rats, rabbits and monkeys. Testicular atrophy was observed at high dose levels in rats. Reproductive studies in mice showed no effect on pregnancy, but there was a decrease in litter size at birth. Teratogenic effects were usually associated with high, single, daily doses that were also associated with other signs of maternal toxicity. High daily levels given as divided doses were less toxic to the conceptus than when given as a single dose. Reduced fetal body weight was observed in rats. A reversible delay in ossification of the sternum was observed in rats at a relatively low dose given by gavage. With administration in drinking-water, similar effects were seen, but at higher doses.
One epidemiological study revealed no effect of caffeine (in coffee-drinking subjects) on the sex ratio of their children. In lymphocytes of normal, caffeine-exposed people, chromosomal aberrations were not observed. An increased frequency of micronucleated blood cells was observed in otherwise healthy splenectomized people exposed to caffeine. Urine of caffeine-exposed persons was not mutagenic to Salmonella typhimurium.
Although it has been suggested that caffeine may induce gene mutations in mammals and man, direct evidence in vivo is limited and the indirect evidence is based largely on extrapolation from results in lower organisms, in which there is no doubt about the mutagenic action of caffeine, and from cultured mammalian cells, in which caffeine is clastogenic at high concentrations.
Overall, caffeine affects photoreactivation, excision repair and postreplication repair. The antagonistic effect of caffeine on mutations induced by ultraviolet radiation has been explained on the basis of inhibition of an error-prone, postreplicative, recombination repair process. Caffeine can modulate the effects of xenobiotics by acting on (i) cytochrome P450, (ii) cAMP metabolism, (iii) DNA metabolism, chromatin structure and function and (iv) nucleotide pools.
4.5. Evaluation1
There is inadequate evidence for the carcinogenicity in humans of caffeine.
There is inadequate evidence for the carcinogenicity in experimental animals of caffeine.
Overall evaluation
Caffeine is not classifiable as to its carcinogenicity to humans (Group 3).
5. References
- Adler I.-D. Cytogenetic investigations of mutagenic action of caffeine in premeiotic spermiogenesis in mice. Humangenetik. 1966;3:82–83. [PubMed: 5986058]
- Adler I.-D. Does caffeine induce dominant lethal mutations in mice? Humangenetik. 1969;7:137–148. [PubMed: 5799491]
- Adler, I.-D. (1970) The problems of caffeine mutagenicity. In: Vogel, F. & Röhrborn, G., eds, Chemical Mutagenesis in Mammals and Man, Berlin, Springer, pp. 383–403.
- Adler I.-D., Röhrborn G. Cytogenetic investigation of meiotic chromosomes of male mice after chronic caffeine treatment. Humangenetik. 1969;8:81–85. [PubMed: 5365587]
- Adler I.-D., Schöneich J. Mutagenic action of caffeine in an ascites tumour strain of mice: cytogenetic investigation. Humangenetik. 1967;4:374–376. [PubMed: 6065796]
- Aeschbacher H.U., Chappuis C. Non-mutagenicity of urine from coffee drinkers compared with that from cigarette smokers. Mutat. Res. 1981;89:161–177. [PubMed: 7027029]
- Aeschbacher H.U., Wurzner H.R. Effect of methylxanthines on hepatic microsomal enzymes in the rat. Toxicol, appl. Pharmacol. 1975;33:575–581. [PubMed: 1188953]
- Aeschbacher H.U., Milon H., Wurzner H.R. Caffeine concentrations in mice plasma and testicular tissue and the effect of caffeine on the dominant lethal test. Mutat. Res. 1978;57:193–200. [PubMed: 351390]
- Aeschbacher H.U., Chappuis C., Wurzner H.R. Mutagenicity testing of coffee: a study of problems encountered with the Ames Salmonella test system. Food Cosmet. Toxicol. 1980;18:605–613. [PubMed: 7009349]
- Aeschbacher H.U., Meier H., Jaccaud E. The effect of caffeine in the in vivo SCE and micronucleus mutagenicity tests. Mutat. Res. 1986;174:53–58. [PubMed: 3702906]
- Ahmad A., Leupold U. On a possible correlation between fine-structure map expansion and reciprocal recombination based on crossing-over. Mol. gen. Genet. 1973;123:143–158. [PubMed: 4725966]
- Ahnström G., Natarajan A.T. Repair of gamma-ray and neutron-induced lesions in germinating barley seeds. Int. J. Radiat. Biol. 1971;19:433–443. [PubMed: 5314344]
- Ahokas J.T., Pelkonen O., Ravenscroft P.J., Emmerson B.T. Effects of theophylline and caffeine on liver microsomal drug metabolizing mono-oxygenase in genetically AHH-responsive and non-responsive mice. Res. Commun. Subst. Abuse. 1981;2:277–290.
- Aitken R.J., Best F., Richardson D.W, Schats R., Simm G. Influence of caffeine on movement characteristics, fertilizing capacity and ability to penetrate cervical mucus of human spermatozoa. J. Reprod. Fertil. 1983;67:19–27. [PubMed: 6822971]
- Alderson T., Khan A.H. Caffeine-induced mutagenesis in Drosophila. Nature. 1967;215:1080–1081. [PubMed: 6053434]
- Aldridge A., Neims A.H. The effects of phenobarbital and βnaphthoflavone on the elimination kinetics and metabolic pattern of caffeine in the beagle dog. Drug Metab. Disposition. 1979;7:378–382. [PubMed: 43223]
- Aldridge A., Neims A.H. Relationship between the clearance of caffeine and its 7-N-demethylation in developing beagle puppies. Biochem. Pharmacol. 1980;29:1909–1914. [PubMed: 7396998]
- Aldridge A., Parsons W.D., Neims A.H. Stimulation of caffeine metabolism in the rat by 3-methylcholanthrene. Life Sci. 1977;21:967–974. [PubMed: 927023]
- Aldridge A., Aranda J.V., Neims A.H. Caffeine metabolism in the newborn. Clin. Pharmacol. Then. 1979;25:447–453. [PubMed: 428190]
- Aldridge A., Bailey J., Neims A.H. The disposition of caffeine during and after pregnancy. Semin. Perinatol. 1981;5:310–314. [PubMed: 7302604]
- Al-Hachim G.M. Teratogenicity of caffeine: a review. Eur. J. Obstet. Gynecol, reprod. Biol. 1989;31:237–247. [PubMed: 2666182]
- Allen S.S., Froberg D.G. The effect of decreased caffeine consumption on benign proliferative breast disease: a randomized clinical trial. Surgery. 1987;101:720–730. [PubMed: 3296263]
- Althaus, F.R. & Richter, C. (1987) ADP-Ribosylation of Proteins, Berlin, Springer.
- Amacher D.E., Zelljadt I. Mutagenic activity of some clastogenic chemicals at the hypoxanthine guanine phosphoribosyl transferase locus of Chinese hamster ovary cells. Mutat. Res. 1984;136:137–145. [PubMed: 6717480]
- Amacher D.E., Paillet S.C., Turner G.N., Ray V.A., Salsburg D.S. Point mutations at the thymidine kinase locus in L5178Y mouse lymphoma cells II. Test validation and interpretation. Mutat. Res. 1980;72:447–474. [PubMed: 7453708]
- Anderson K.E., Conney A.H., Kappas A. Nutritional influences on chemical biotransformations in humans. Nutr. Rev. 1982;40:161–171. [PubMed: 6750455]
- Andersson H.C. G2 repair and the formation of chromosomal aberrations III. The effect of hydroxyurea, 5-fluorodeoxyuridine and caffeine on X-ray-induced chromosome damage in Vicia faba. Hereditas. 1982;97:193–209.
- Andrew L.E. The mutagenic activity of caffeine in Drosophila. Am. Nat. 1959;43:135–138.
- Andriadzee M.I., Pleskach N.M., Mikhelson V.M., Zhestyanikov V.D. Spontaneous and induced sister chromatid exchanges in blood lymphocytes of healthy donors and a xeroderma pigmentosum patient under the action of inhibitors of DNA synthesis and repair: novobiocin, 3-methoxybenzamide and caffeine (Russ.). Tsitologia. 1986;28:69–85. [PubMed: 3082051]
- Anon. (1973) Caffeine, coffee, and cancer Br. med. J., 281 1031–1032.
- Anon. (1983) Caffeine levels in Australian foods. Food Technol. Austr. 35 87.
- Anon. (1986) Caffeine’s no wide-awake market. Chem. Bus., 8 44.
- Anon. (1987a) Caffeine prices skyrocketing as total supplies tighten. Chem. Mark. Rep., 6 April, 201.
- Anon. (1987b) Caffeine. Food Chem. News Guide, 25 May, 78.1.
- Anon. (1988) Caffeine prices strengthened as coffee consumption slides. Chem. Mark. Rep., 28 November, pp. 17–18.
- Anon. (1989a) Caffeine seen as stable despite Chinese imports. Chem. Mark. Rep., 7 August, 20–21.
- Anon. (1989b) Caffeine content for various sodas. Beverage Ind., June, 57.
- Anon. (1989c) Annual soft drink report. Beverage Ind., March.
- Apfelzweig R.A., Teplitz R.L. A study of the effect of caffeine upon excision repair of damaged DNA. Mutat. Res. 1979;62:151–158. [PubMed: 492195]
- Aranda J.V., Collinge J.M., Zinman R., Watters G. Maturation of caffeine elimination in infancy. Arch. Dis. Child. 1979a;54:946–949. [PMC free article: PMC1545746] [PubMed: 533298]
- Aranda J.V., Cook C.E., Gorman W., Collinge J.M., Loughnan P.M., Outerbridge E.W., Aldridge A., Neims A.H. Pharmacokinetic profile of caffeine in the premature newborn infant with apnea. J. Pediatr. 1979b;94:663–668. [PubMed: 430317]
- Aranda J.V., Beharry K., Rex J., Johannes R.J., Charest-Boule L. Caffeine enzyme immunoassay in neonatal and pediatric drug monitoring. Ther. Drug Monit. 1987;9:97–103. [PubMed: 3554632]
- Arisimova L.E. Comparative study of sex chromosome nondisjunction and loss frequencies in female and male Drosophila melanogaster under the combined action of caffeine and X-rays (Russ.). Vestn. Leningr. Univ. Biol. 1975;2:145–147.
- Atiza R.R., Dorado G., Barbancho M., Pueyo C. Study of the causes of direct-acting mutagenicity in coffee and tea using the ara test in Salmonella typhimurium. Mutat. Res. 1988;201:89–96. [PubMed: 3047575]
- Arlett C.F, Harcourt S.A. The induction of 8-azaguanine-resistant mutants in cultured Chinese hamster cells by ultraviolet light The effect of changes in post-irradiation conditions. Mutat. Res. 1972;14:431–437. [PubMed: 5062850]
- Armuth V., Berenblum I. The effect of caffeine on two-stage skin carcinogenesis and on complete systemic carcinogenesis. Carcinogenesis. 1981;2:977–979. [PubMed: 7296765]
- Arnandova R., Kaculov A. Coffee and pregnancy (Russ.). Akush. Ginekol. (Sofia). 1978;17:57–61.
- Arnaud M.J. Identification, kinetic and quantitative study of [2-14C] and [1-Me14C] caffeine metabolites in rat’s urine by chromatographic separations. Biochem. Med. 1976;16:67–76. [PubMed: 1008870]
- Arnaud, M.J. (1984) Products of metabolism of caffeine. In: Dews, P.B., ed., Caffeine. Perspectives from Recent Research, Berlin, Springer, pp. 3–38.
- Arnaud M.J. Pharmacokinetics in animal chronic toxicological studies. J. Pharm. clin. 1985a;4:259–268.
- Arnaud M.J. Comparative metabolic disposition of [1-Me14C]caffeine in rats, mice, and Chinese hamsters. Drug Metab. Disposition. 1985b;13:471–478. [PubMed: 2863113]
- Arnaud M.J. The pharmacology of caffeine. Progr. Drug Res. 1987;31:273–313. [PubMed: 3326033]
- Arnaud, M.J. & Getaz, F. (1986) Effect of pregnancy on [1,3,7-Me14C]caffeine breath test in the rat. Acta pharmacol. toxicol., Suppl. 5, Abstract 859.
- Arnaud, M.J. & Welsch, C. (1980a) Comparison of caffeine metabolism by perfused rat liver and isolated microsomes. In: Coon, M J., Conney, A.H., Estabrook, R.W., Gelboin, H.V., Gillette, J.R. & O’Brien, P.J., eds, Microsomes, Drug Oxidations and Chemical Carcinogenesis, Vol. II, New York, Academic Press, pp. 813–816.
- Arnaud, M.J. & Welsch, C. (1980b) Caffeine metabolism in human subjects. In: 9e Colloque Scientifique International sur le Café, London, 1980, Paris, Association Scientifique Internationale du Café, pp. 385–396.
- Arnaud, M.J. & Welsch, C. (1982) Theophylline and caffeine metabolism in man. In: Rietbrock, N., Woodcock, B.G. & Staib, A.H., eds, Theophylline and Other Methylxanthines, Braunschweig, Wiesbaden, Friedr. Vieweg and Sohn, pp. 135–148.
- Arnaud M.J., Ben-Zvi Z., Yaari A., Gorodischer R. 1,3,8-Trimethylallantoin: a major caffeine metabolite formed by rat liver. Res. Commun, chem. Pathol. Pharmacol. 1986a;52:407–410. [PubMed: 3738216]
- Arnaud M.J., Richli U., Philippossian G. Isolation and identification of paraxanthine (Px) glucuronide as the major caffeine metabolite in mice (Abstract). Experientia. 1986b;42:696.
- Arnaud, M.J., Bracco, I. & Getaz, F. (1989) Synthesis of ring labelled caffeine for the study of metabolic and pharmacokinetics. Mouse interstrain differences in relation to pharmacologic and toxic effects. In: Baillie, T.A. & Jones, J.R., eds, Synthesis and Applications of Isotopically Labelled Compounds, Amsterdam, Elsevier, pp. 645–648.
- Ashton C.H. Caffeine and health. Br. med. J. 1987;295:1293–1294. [PMC free article: PMC1248372] [PubMed: 3120982]
- Ax R.L., Collier R.J., Lodge J.R. Effects of dietary caffeine on the testis of domestic fowl, Gallus domesticus. J. Reprod. Fertil. 1976;47:235–238. [PubMed: 957321]
- Axelrod J., Reichenthal J. The fate of caffeine in man and a method for its estimation in biological material. J. Pharmacol. exp. Ther. 1953;107:519–523. [PubMed: 13053413]
- Bailey D.N., Weibert R.T., Naylor A.J., Shaw R.F. A study of salicylate and caffeine excretion in the breast milk of two nursing mothers. J. anal. Toxicol. 1982;6:64–68. [PubMed: 7098450]
- Balachandran R., Srinivasan A. Caffeine inhibits DNA polymerase I from Escherichia coli: studies in vitro. Carcinogenesis. 1982;3:151–153. [PubMed: 7039855]
- Balansky R.M., Blagoeva P.M., Mirtcheva Z. The influence of selenium and caffeine on chemical carcinogenesis in rats, mutagenesis in bacteria, and unscheduled DNA synthesis in human lymphocytes. Biol. Trace Element Res. 1983;5:331–343. [PubMed: 24263571]
- Barfknecht T.R., Shankel D.M. The effect of streptomycin resistance, caffeine and acriflavine on ultraviolet light-induced reversion to tryptophan independence in strains of Escherichia coli B/r. Mutat. Res. 1975;30:163–176. [PubMed: 1107829]
- Barkay J., Bartoov B., Ben-Ezra S., Langsam J., Feldman E., Gordon S., Zuckerman H. The influence of in vitro caffeine treatment on human sperm morphology and fertilizing capacity. Fertil Steril. 1984;41:913–918. [PubMed: 6539253]
- Barone, J.J. & Roberts, H. (1984) Human consumption of caffeine. In: Dews, P.B., ed., Caffeìne: Perspectives from Recent Research, Berlin, Springer, pp. 59–73.
- Basler A., Bachmann U., Roszinsky-Köcher G., Röhrborn G. Effects of caffeine on sister-chromatid exchanges (SCE) in vivo. Mutat. Res. 1979;59:209–214. [PubMed: 108595]
- Bateman A.J. A storm in a coffee cup. Mutat. Res. 1969;7:475–478. [PubMed: 5807341]
- Batikjan G.G., Pogosjan V.S. Investigation of caffeine action in different tissues of Coreopsis tinctoria Nutt (Russ.). Tsitol. Genet. 1976;10:240–243. [PubMed: 1014090]
- Beach C.A., Bianchine J.R., Gerber N. The excretion of caffeine in the semen of men: comparison of the concentrations in blood and semen. Proc. west. Pharmacol. Soc. 1982;25:377–380. [PubMed: 7122524]
- Beach C.A., Bianchine J.R., Gerber N. The excretion of caffeine in the semen of men: pharmacokinetics and comparison of the concentrations in blood and semen. J. clin. Pharmacol. 1984;24:120–126. [PubMed: 6715563]
- van den Berg, B.J. (1977) Epidemiologic observations of prematurity: effects of tobacco, coffee and alcohol. In: Reed, D.M. & Stanley, F.J., eds, The Epidemiology of Prematurity, Baltimore, Urban & Schwarzenberg, pp. 157–176.
- Bergmann F., Dikstein S. Studies on uric acid and related compounds III. Observations on the specificity of mammalian xanthine oxidases. J. biol. Chem. 1956;223:765–780. [PubMed: 13385225]
- Berkowitz G.S., Holford T.R., Berkowitz R.L. Effects of cigarette smoking, alcohol, coffee and tea consumption on preterm delivery. Early Hum. Dev. 1982;7:239–250. [PubMed: 7160334]
- Berthou F., Ratanasavanh D., Riche C., Picart D., Voirin T., Guillouzo A. Comparison of caffeine metabolism by slices, microsomes and hepatocyte cultures from adult human liver. Xenobiotica. 1989;19:401–417. [PubMed: 2750203]
- Bertrand M., Schwam E., Frandon A., Vagne A., Alary J. The systematic and specific teratogenic effect of caffeine in rodents (Fr.). C.R. Soc. Biol. (Paris). 1965;159:2199–2202.
- Bertrand M., Girod J., Rigaud M.F. Ectrodactyly induced by caffeine in rodents: role of specific and genetic factors (Fr.). C.R. Soc. Biol. (Paris). 1970;164:1488–1489. [PubMed: 4251927]
- Bertrand C.A., Pomper I., Hillman G., Duffy J.C., Micheli I. No relation between coffee and blood pressure (Letter to the Editor). New Engl. J. Med. 1978;299:315–316. [PubMed: 661940]
- Bień, M., Piatkowski, J. & Lachowicz, T.M. (1989) Inhibition of mating process by caffeine and its effect on antibiotic marker segregation in yeast Saccharomyces cerevisiae. In: Martini, A. & Vaughan Martini, A., eds, Yeast as a Main Protagonist of Biotechnology, Vol. 2, London, John Wiley & Sons, pp. S267–S271. [PubMed: 2665360]
- Birkett N.J., Logan A.G. 1988Caffeine-containing beverages and the prevalence of hypertension J. Hypertension 6(Suppl. 4)S620–S622. [PubMed: 3241268]
- Bishun N., Williams D., Mills J. The cytogenetic effects of caffeine on two tumour cell lines. Mutat. Res. 1974;26:151–155. [PubMed: 4822596]
- Blanchard J., Sawers S.J.A. The absolute bioavailability of caffeine in man. Eur. J. clin. Pharmacol. 1983a;24:93–98. [PubMed: 6832208]
- Blanchard J., Sawers S.J.A. Comparative pharmacokinetics of caffeine in young and elderly men. J. Pharmacokin. Biopharm. 1983b;11:109–126. [PubMed: 6886969]
- Blauch J.L., Tarka S.M. Jr. HPLC determination of caffeine and theobromine in coffee, tea and instant hot cocoa mixes. J. Food Sci. 1983;48:745–750.
- Bonati, M. & Garattini, S. (1984) Interspecies comparison of caffeine disposition. In: Dews, P.B., ed., Caffeine. Perspectives from Recent Research, Berlin, Springer, pp. 48–56.
- Bonati M., Garattini S. Pharmacokinetics of caffeine. ISI Atlas Sci. Pharmacol. 1988;2:33–39.
- Bonati M., Latini R., Galletti F., Young J.F., Tognoni G., Garattini S. Caffeine disposition after oral doses. Clin. Pharmacol. Ther. 1982;32:98–106. [PubMed: 7083737]
- Bonati M., Latini R., Tognoni G., Young J.F., Garattini S. Interspecies comparison of in vivo caffeine pharmacokinetics in man, monkey, rabbit, rat, and mouse. Drug Metab. Rev. 1984–85;15:1355–1383. [PubMed: 6543526]
- Boothman D.A., Schlegel R., Pardee A.B. Anticarcinogenic potential of DNA-repair modulators. Mutat. Res. 1988;202:393–411. [PubMed: 2973563]
- Borlée I., Lechat M.F., Bouckaert A., Misson C. Coffee, risk factor during pregnancy (Fr.). Louvain Méd. 1978;97:279–284.
- Bott, K. (1982) Caffeine from Theophylline, Carbon Monoxide and Methanol, German Patent 3,113,880 (BASF A.-G.) [Chem Abstr. 98 53545m]
- Bowden G.T., Hsu I.C., Harris C.C. The effect of caffeine on cytotoxicity, mutagenesis, and sister-chromatid exchanges in Chinese hamster cells treated with dihydrodiol epoxide derivatives of benzo[a]pyrene. Mutat. Res. 1979;63:361–370. [PubMed: 522876]
- Boyd J.B., Presley J.M. Repair replication and photorepair of DNA in larvae of Drosophila melanogaster. Genetics. 1974;77:687–700. [PMC free article: PMC1213161] [PubMed: 4371150]
- Boyd J.B., Shaw K.E.S. Postreplication repair defects in mutants of Drosophila melanogaster. Mol. gen. Genet. 1982;186:289–294. [PubMed: 6810065]
- Boyle C.A., Berkowitz G.S., LiVolsi V.A., Ort S., Merino M.J., White C., Kelsey J.L. Caffeine consumption and fibrocystic breast disease: a case-control epidemiologic study. J. natl Cancer Inst. 1984;72:1015–1019. [PubMed: 6585579]
- Boynton A.L., Evans T.C., Crouse D.A. Effects of caffeine on radiation-induced mitotic inhibition in S-180 ascites tumor cells. Radiat. Res. 1974;60:89–97. [PubMed: 10881702]
- Bradbrook I.D., James C.A., Morrison P.J., Rogers H.J. Comparison of thin-layer and gas chromatographic assays for caffeine in plasma. J. Chromatogr. 1979;163:118–122. [PubMed: 528623]
- Bradford J.C., Caldwell J.A., Barbolt T.A., Drobeck H.P. 1983aChronic administration of caffeine to two generations of rats (Abstract) Teratology 2732A.
- Bradford J.C., Naismith R.W., Sorg R.M., Godek E.G., Matthews R.J., Caldwell J.A., Drobreck H.P. Micronucleus test and body fluid analysis of rats exposed to caffeine in a reproductive study (Abstract No Cb-10). Environ. Mutagenesis. 1983b;5:402.
- Brazier J.L., Ritter J., Berland M., Khenfer D., Faucon G. Pharmacokinetics of caffeine during and after pregnancy. Dev. Pharmacol. Ther. 1983;6:315–322. [PubMed: 6628163]
- Brøgger A. Caffeine-induced enhancement of chromosome damage in human lymphocytes treated with methyl methanesulfonate, mitomycin C and X-rays. Mutat. Res. 1974;23:353–360. [PubMed: 4366873]
- Brooke O.G., Anderson H.R., Bland J.M., Peacock J.L., Stewart C.M. Effects on birth weight of smoking, alcohol, caffeine, socioeconomic factors, and psychosocial stress. Br. med. J. 1989;298:795–801. [PMC free article: PMC1836053] [PubMed: 2496859]
- Brooks P.G., Gart S., Heldfond A.J., Margolin .M.L., Allen A.S. Measuring the effect of caffeine restriction on fibrocystic breast disease: the role of graphic stress telethermometry as an objective monitor of disease. J. reprod. Med. 1981;26:279–282. [PubMed: 7252948]
- Brown C.R., Jacob P. III, Wilson M., Benowitz N.L. Changes in rate and pattern of caffeine metabolism after cigarette abstinence. Clin. Pharmacol. Ther. 1988;43:488–491. [PubMed: 3365914]
- Brune H., Deutsch-Wenzel R.P., Habs M., Ivankovic S., Schmähl D. Investigation of the tumorigenic response to benzo(a)pyrene in aqueous caffeine solution applied orally to Sprague-Dawley rats. J. Cancer Res. clin. Oncol. 1981;102:153–157. [PubMed: 7338532]
- Budavari, S., ed. (1989) The Merck Index, 11th ed., Rahway, NJ, Merck & Co., p. 248.
- Buhl S.N., Regan J.D. 1974Effect of caffeine on postreplication repair in human cells Biophys. J. 14519–527. [PMC free article: PMC1334515] [PubMed: 4836668]
- Burg A.W. Effects of caffeine on the human system. Tea Coffee Trade J. 1975a;147:40–42.
- Burg A.W. Physiological disposition of caffeine. Drug Metab. Rev. 1975b;4:199–228. [PubMed: 816628]
- Burg A.W., Werner E. Tissue distribution of caffeine and its metabolites in the mouse. Biochem. Pharmacol. 1972;21:923–936. [PubMed: 5039752]
- Butler M.A., Iwasaki M., Guengerich F.P., Kadlubar F.F. Human cytochrome P-450PA (P-450IA2), the phenacetin O-deethylase, is primarily responsible for the hepatic 3-demethylation of caffeine and N-oxidation of carcinogenic arylamines. Proc. natl Acad. Sci. USA. 1989;86:7696–7700. [PMC free article: PMC298137] [PubMed: 2813353]
- Byfield J.E., Murnane J., Ward J.F., Calabro-Jones P., Lynch M., Kulhanian F. Mice, men, mustards and methylated xanthines: the potential role of caffeine and related drugs in the sensitization of human tumours to alkylating agents. Br. J. Cancer. 1981;43:669–683. [PMC free article: PMC2010679] [PubMed: 7248151]
- Caan B.J., Goldhaber M.K. Caffeinated beverages and low birthweight: a case-control study. Am. J. public Health. 1989;79:1299–1300. [PMC free article: PMC1349709] [PubMed: 2764212]
- Callahan M.M., Robertson R.S., Arnaud M.J., Branfman A.R., McComish M.F., Yesair D.W. Human metabolism of [1-methyl-14C]- and [2–14C]caffeine after oral administration. Drug Metab. Disposition. 1982;10:417–423. [PubMed: 6126344]
- Callahan M.M., Robertson R.S., Branfman A.R., McComish M.F., Yesair D.W. Comparison of caffeine metabolism in three nonsmoking populations after oral administration of radiolabeled caffeine. Drug Metab. Disposition. 1983;11:211–217. [PubMed: 6135578]
- Carlson P.S. Mitotic crossing-over in a higher plant. Genet. Res. 1974;24:109–112.
- Casto B.C., Pieczynski W.J., Janosko N., DiPaolo J.A. Significance of treatment interval and DNA repair in the enhancement of viral transformation by chemical carcinogens and mutagens. Chem.-biol. Interact. 1976;13:105–125. [PubMed: 816474]
- Cattanach B.M. Genetical effects of caffeine in mice. Z. Vererbungsl. 1962;93:215–219. [PubMed: 13877380]
- Cattanach, B.M. (1964) A genetical approach to the effects of radiomimetic chemicals on fertility in mice. In: Carlson, W.D. & Gassner, F.X., eds, Effects of Ionizing Radiation on the Reproductive System, Oxford, Pergamon, pp. 415–426.
- Ceccherini I., Loprieno N., Sbrana I. Caffeine post-treatment causes a shift in the chromosome aberration types induced by mitomycin C, suggesting a caffeine-sensitive mechanism of DNA repair in G2. Mutagenesis. 1988;3:39–44. [PubMed: 3128718]
- Cesarone, C.F., Bolognesi, C. & Santi, L. (1982) Evaluation of damage to DNA after in vivo exposure to different classes of chemicals. Arch. Toxicol., Suppl. 5, 355–359.
- Chang C.-C., Philipps C., Trosko J.E., Hart R.W. Mutagenic and epigenetic influence of caffeine on the frequencies of UV-induced ouabain-resistant Chinese hamster cells. Mutat. Res. 1977;45:125–136. [PubMed: 917032]
- Chetsanga C.J., Rushlow K., Boyd V. Caffeine enhancement of digestion of DNA by nuclease S1. Mutat. Res. 1976;34:11–20. [PubMed: 2867]
- Christensen, H.D. & Isernhagen, R. (1981) The application of the radial compression separation system for biological materials. In: Hawk, G.L., ed., Biological/Biomedical Applications of Liquid Chromatography, Vol. 3, New York, Marcel Dekker, pp. 71–93.
- Christensen, H.D. & Neims, A.H. (1984) Measurement of caffeine and its metabolites. In: Dews, P.B., ed., Caffeine. Perspectives from Recent Research, Berlin, Springer, pp. 39–47.
- Christensen H.D., Whitsett T.L. Measurements of xanthines and their metabolites by means of high pressure liquid chromatography. Chromatogr. Sci. 1979;10:507–537.
- Christensen, H.D., Manion, C.V. & Kling, O.R. (1981) Caffeine kinetics during late pregnancy. In: Soyka, L.F. & Redmond, G.R, eds, Drug Metabolism in the Immature Human, New York, Raven Press, pp. 163–181.
- Chvasta T.E., Cooke A.R. Emptying and absorption of caffeine from the human stomach. Gastroenterology. 1971;61:838–843. [PubMed: 5125686]
- Clark A.M., Clark E.G. The genetic effects of caffeine in Drosophila melanogaster. Mutat. Res. 1968;6:227–234. [PubMed: 5714161]
- Clarke C.H., Shankel D.M. Antimutagenesis in microbial systems. Bacteriol. Rev. 1975;39:33–53. [PMC free article: PMC413882] [PubMed: 235256]
- Clarke C.H., Wade M.J. Evidence that caffeine, 8-methoxypsoralene and steroidal diamines are frameshift mutagens for E. coli K-12. Mutat. Res. 1975;28:123–125. [PubMed: 1095915]
- Collins T.F.X., Welsh J.J., Black T.N., Collins E.V. A study of the teratogenic potential of caffeine given by oral intubation to rats. Regul. Toxicol. Pharmacol. 1981;1:355–378.
- Collins T.F.X., Welsch J.J., Black T.N., Ruggles D.I. A study of the teratogenic potential of caffeine ingested in drinking water. Food chem. Toxicol. 1983;21:763–777. [PubMed: 6686582]
- Collins T.F.X., Welsh J.J., Black T.N., Whitby K.E., O’Donnell M.W. Jr. Potential reversibility of skeletal effects in rats exposed in utero to caffeine. Food chem. Toxicol. 1987;25:647–662. [PubMed: 3653820]
- Consumers Union (1990) US Pharmacopeia. Drug Information for the Consumer, Easton, PA, Mack Publishing, pp. 274–277, 287–289.
- Cook C.E., Tallent C.R., Amerson E.W., Myers M.W., Kepler J.A., Taylor G.F., Christensen H.D. Caffeine in plasma and saliva by a radioimmunoassay procedure. J. Pharmacol. exp. Ther. 1976;199:679–686. [PubMed: 1033273]
- Craig W.J., Nguyen T.T. Caffeine and theobromine levels in cocoa and carob products. J. Food Sci. 1984;49:302–305.
- Dalvi R.R. Acute and chronic toxicity of caffeine: a review. Vet. hum. Toxicol. 1986;28:144–150. [PubMed: 2871652]
- Debry, G. (1989) Le Café [Coffee], Nancy, Centre de Nutrition Humaine.
- De Flora S., Zanacchi P., Camoirano A., Bennicelli C., Badolati G.S. Genotoxic activity and potency of 135 compounds in the Ames reversion test and in a bacterial DNA-repair test. Mutat. Res. 1984a;133:161–198. [PubMed: 6374443]
- De Flora S., Camoirano A., Zanacchi P., Bennicelli C. Mutagenicity testing with TA97 and TA102 of 30 DNA-damaging compounds, negative with other Salmonella strains. Mutat. Res. 1984b;134:159–165. [PubMed: 6390191]
- Delvaux A.-M., Devoret R. E. coli K12. Mutat. Res. Vol. 7. 1969. The occurrence of suppressors in caffeine-resistant mutants from; pp. 273–285. [PubMed: 4896920]
- De Marco A., Cozzi R. Chromosomal aberrations induced by caffeine in somatic ganglia of Drosophila melanogaster. Mutat. Res. 1980;69:55–69. [PubMed: 6767180]
- De Marco A., Polani S. Cell-stage-specific enhancement by caffeine of the frequency of chromatid aberrations induced by X-rays in neural ganglia of Drosophila melanogaster. Mutat. Res. 1981;84:91–99. [PubMed: 6799815]
- Demas T., Statland B.E. Prolonged half-life of serum caffeine in patients with hepatic insufficiency (Abstract No 203). Clin. Chem. 1977;23:1156.
- Demerec M., Bertani G., Flint J. A survey of chemicals for mutagenic action on E coli. Am. Naturalist. 1951;85:119–136.
- Denda A., Yokose Y., Emi Y., Murata Y., Ohara T., Sunagawa M., Mikami S., Takahashi S., Konishi Y. Effects of caffeine on pancreatic tumorigenesis by 4-hydroxyaminoquinoline 1-oxide in partially pancreatectomized rats. Carcinogenesis. 1983;4:17–22. [PubMed: 6401601]
- Desmond P.V., Patwardhan R.V., Johnson R.F., Schenker S. Impaired elimination of caffeine in cirrhosis. Dig. Dis. Sci. 1980;25:193–197. [PubMed: 7371463]
- De Vries J.W., Johnson K.D., Heroff J.C. HPLC determination of caffeine and theobromine content of various natural and red dutched cocoas. J. Food Sci. 1981;46:1968–1969.
- Dews P.B. Caffeine. Ann. Rev. Nutr. 1982;2:323–341. [PubMed: 6764734]
- Dobmeyer D.J., Stine R.A., Leier C.V., Greenberg R., Schaal S.F. The arrhythmogenic effects of caffeine in human beings. New Engl. J. Med. 1983;308:814–816. [PubMed: 6835272]
- Dong M., Hoffmann D., Locke D.C., Ferrand E. The occurrence of caffeine in the air of New York City. Atmos. Environ. 1977;11:651–653.
- Dorrbecker S.H., Ferraina R.A., Dorrbecker B.R., Kramer P.A. Caffeine and paraxanthine pharmacokinetics in the rabbit: concentration and product inhibition effects. J. Pharmacokinet. Biopharmacol. 1987;15:117–132. [PubMed: 3612497]
- Dorrbecker S.H., Raye J.R., Dorrbecker B.R., Kramer P.A. Caffeine disposition in the pregnant rabbit I. Pharmacokinetics following administration by intravenous bolus and continuous zero-order infusion. Dev. Pharmacol. Ther. 1988;11:109–117. [PubMed: 3371145]
- Dougherty J.B., Kelsen D., Kemeny N., Magill G., Botet J., Niedzwiecki D. Advanced pancreatic cancer: a phase I–II trial of cisplatin, high-dose cytarabine and caffeine. J. natl Cancer Inst. 1989;81:1735–1738. [PubMed: 2681796]
- Dulout F.N., Larramendy M.L., Olivero O.A. Effect of caffeine on the frequency of chromosome aberrations induced in vivo by triethylenemelamine (TEM) and adriamycin (ADR) in mice. Mutat. Res. 1981;82:295–304. [PubMed: 7266560]
- Dunkel V.C., Zeiger E., Brusick D., McCoy E., McGregor D., Mortelmans K., Rosenkranz H.S., Simmon V.E. 1985Reproducibility of microbial mutagenicity assays: II. Testing of carcinogens and noncarcinogens in Salmonella typhimurium and Escherichia coli Environ. Mutagenesis 7(Suppl.5)1–248. [PubMed: 3905369]
- Dunlop M., Court J.M. Effects of maternal caffeine ingestion on neonatal growth in rats. Biol. Neonat. 1981;39:178–184. [PubMed: 7295838]
- Dunn T.L., Gardiner R.A., Seymour G.J., Lavin M.F. Genotoxicity of analgesic compounds assessed by an in vitro micronucleus assay. Mutat. Res. 1987;189:299–306. [PubMed: 3670333]
- van Dusseldorp M., Smits P., Thien T., Katan M.B. Effect of decaffeinated versus regular coffee on blood pressure. A 12-week, double-blind trial. Hypertension. 1989;14:563–569. [PubMed: 2680964]
- Elmazar M.M.A., McElhatton P.R., Sullivan F.M. Studies on the teratogenic effects of different oral preparations of caffeine in mice. Toxicology. 1982;23:57–71. [PubMed: 7089985]
- El-Yazigi A., Chaleby K., Martin C.R. A simplified and rapid test for acetylator phenotyping by use of the peak height ratio of two urinary caffeine metabolites. Clin. Chem. 1989;35:848–851. [PubMed: 2566399]
- Epstein S.S., Shafner H. Chemical mutagens in the human environment. Nature. 1968;219:385–387. [PubMed: 5662155]
- Epstein S.S., Bass W., Arnold E., Bishop Y. The failure of caffeine to induce mutagenic effects or to synergize the effects of known mutagens in mice. Food Cosmet. Toxicol. 1970;8:381–401. [PubMed: 5530812]
- Ernster V.L., Mason L., Goodson W.H., Sickles E.A., Sacks S.T., Selvin S., Dupuy M.E., Hawkinson J., Hunt T.K. Effects of caffeine-free diet on benign breast disease: a randomized trial. Surgery. 1982;91:263–267. [PubMed: 7058508]
- Evans D.A.P., White T.A. Human acetylation polymorphism. J. Lab. clin. Med. 1964;63:394–403. [PubMed: 14164493]
- Faed M.J.W., Mourelatos D. Enhancement by caffeine of sister-chromatid exchange frequency in lymphocytes from normal subjects after treatment by mutagens. Mutat. Res. 1978;49:437–440. [PubMed: 634309]
- Federation of American Societies for Experimental Biology (1978) Evaluation of the Health Aspects of Caffeine as a Food Ingredient. A Report to the Food and Drug Administration by the Select Committee on GRAS Substances (Contract No. FDA 223-75-2004; SCOGS-89), Bethesda, MD.
- Feely J., Kelleher P., Odumosu A. The effects of ageing on aminopyrine and caffeine breath tests in the rat Fundam, clin. Pharmacol. 1987;1:409–412. [PubMed: 3447929]
- Ferren W.P., Shane N.A. Differential spectrometric determination of caffeine in soluble coffee and in drug combinations. J. Assoc, off. anal. Chem. 1968;51:573–577.
- Fincke A. The occurrence of caffeine and theobromine in chocolate fats (Ger.). Fet. Seif Anstrichmitt. 1963;65:647–650.
- Findlay J.W.A., DeAngelis R.L., Kearney M.E, Welch R.M., Findlay J.M. Analgesic drugs in breast milk and plasma. Clin. Pharmacol. Ther. 1981;29:625–633. [PubMed: 7214793]
- Fishbein, L., Flamm, W.G. & Falk, H.L. (1970) Chemical Mutagens, New York, Academic Press.
- Foenander T., Birkett D.J., Miners J.O., Wing L.M.H. The simultaneous determination of theophylline, theobromine and caffeine in plasma by high performance liquid chromatography. Clin. Biochem. 1980;13:132–134. [PubMed: 7418197]
- Frei J.V., Venitt S. Chromosome damage in the bone marrow of mice treated with the methylating agents methyl methanesulphonate and N-methyl-N-nitrosourea in the presence or absence of caffeine, and its relationship with thymoma induction. Mutat. Res. 1975;29:89–96. [PubMed: 170515]
- Friedman L., Weinberger M.A., Farber T.M., Moreland F.M., Peters E.L., Gilmore C.E., Khan M.A. Testicular atrophy and impaired spermatogenesis in rats fed high levels of the methylxanthines caffeine, theobromine, or theophylline. J. environ. Pathol. Toxicol. 1979;2:687–706. [PubMed: 422930]
- Fries N., Kihlman B. Fungal mutations obtained with methyl xanthines. Nature. 1948;162:573–574. [PubMed: 18933054]
- Fujii T., Nishimura H. Adverse effects of prolonged administration of caffeine on rat fetus. Toxicol. appl. Pharmacol. 1972;22:449–457. [PubMed: 5041373]
- Furth E.E., Thilly W.G. Caffeine is non-mutagenic to Salmonella typhimurium and human cells in culture. J. Food Saf. 1978;1:222–237.
- Furuhashi N., Sato S., Suzuki M., Hiruta M., Tanaka M., Takahashi T. Effects of caffeine ingestion during pregnancy. Gynecol. obstet. Invest. 1985;19:187–191. [PubMed: 4040880]
- Gabridge M.G., Legator M.S. A host-mediated microbial assay for the detection of mutagenic compounds. Proc. Soc. exp. Biol. Med. 1979;130:831–834. [PubMed: 4886528]
- Galasko G.T.F., Furman K.I., Alberts E. Brief communication. The caffeine contents of non-alcoholic beverages. Food chem. Toxicol. 1989;27:49–51. [PubMed: 2703194]
- Gans J.H. Comparative toxicities of dietary caffeine and theobromine in the rat. Food chem. Toxicol. 1984;22:365–369. [PubMed: 6539285]
- Gennaro, A.R., ed. (1985) Remington’s Pharmaceutical Sciences, 17th ed., Easton, PA, Mack Publishing, pp. 1133–1135.
- George J., Murphy T., Roberts R., Cooksley W.G.E., Halliday J.W., Powell L.W. Influence of alcohol and caffeine consumption on caffeine elimination. Clin. exp. Pharmacol. Physiol. 1986;13:731–736. [PubMed: 3802578]
- Georgian L., Morara I., Comisel V. Caffeine effects on the chromosomal aberrations induced in vivo by sarcolysine and methotrexate. Rev. roum. Morphol. Embryol. Physiol. 1980;26:179–183. [PubMed: 6446036]
- Gezelius K., Fries N. Phage resistant mutants induced in Escherichia coli by caffeine. Hereditas. 1952;38:112–114.
- Ghosh S., Ghosh I. Condensation of interphase chromatin in caffeine-treated cells. Naturwissenschaften. 1972;59:277–278. [PubMed: 5065762]
- Gilbert, R.M. (1981) Caffeine: overview and anthology. In: Miller, S.A., ed., Nutrition and Behavior, Philadelphia, The Franklin Institute Press, pp. 145–166.
- Gilbert, R.M. (1984) Caffeine consumption. In: Spiller, G.A., ed., The Methylxanthine Beverages and Foods: Chemistry, Consumption, and Health Effects, New York, Alan R. Liss, pp. 185–213.
- Gilbert S.G., Stavric B., Klassen R.D., Rice D.C. The fate of chronically consumed caffeine in the monkey (Macaca fascicularis). Fundam, appl.Toxicol. 1985;5:578–587. [PubMed: 4007312]
- Gilbert S.G., So Y., Klassen R.D., Geoffroy S., Stavric B., Rice D.C. Elimination of chronically consumed caffeine in the pregnant monkey (Macaca fascicularis). J. Pharmacol. exp. Ther. 1986;239:891–897. [PubMed: 3795047]
- Gilbert S.G., Rice D.C., Reuhl K.R., Stavric B. Adverse pregnancy outcome in the monkey (Macaca fascicularis) after chronic caffeine exposure. J. Pharmacol, exp. Ther. 1988;245:1048–1053. [PubMed: 3385636]
- Glass E.A., Novick A. Induction of mutation in chloramphenicol-inhibited bacteria. J. Bacteriol. 1959;77:10–16. [PMC free article: PMC290309] [PubMed: 13620643]
- Goldstein A., Warren R. Passage of caffeine into human gonadal and fetal tissue. Biochem. Pharmacol. 1962;11:166–168. [PubMed: 13899873]
- González-Fernández A., Hernández R, López-Sáez J.E. Effect of caffeine and adenosine on G2 repair: mitotic delay and chromosome damage. Mutat. Res. 1985;149:275–281. [PubMed: 3982446]
- Gorodischer R., Karplus M. Pharmacokinetic aspects of caffeine in premature infants with apnoea. Eur. J. clin. Pharmacol. 1982;22:47–52. [PubMed: 7094974]
- Govindwar S.P., Kachole M.S., Pawar S.S. In vivo and in vitro effects of caffeine on hepatic mixed-function oxidases in rodents and chicks. Food chem. Toxicol. 1984;22:371–375. [PubMed: 6539286]
- Grab F.L., Reinstein J.A. Determination of caffeine in plasma by gas chromatography. J. pharm. Sci. 1968;57:1703–1706. [PubMed: 5684739]
- Graf U., Wurgler F.E. Investigation of coffee in Drosophila genotoxicity tests. Food chem. Toxicol. 1986;24:835–842. [PubMed: 3096848]
- Graham D.M. Caffeine — its identity, dietary sources, intake and biological effects. Nutr. Rev. 1978;36:97–102. [PubMed: 353595]
- Graham, H.N. (1984a) Tea: the plant and its manufacture, chemistry and consumption of the beverage. In: Spiller, G.A., ed., The Methylxanthine Beverages and Foods: Chemistry, Consumption, and Health Effects, New York, Alan R. Liss, pp. 29–74. [PubMed: 6527997]
- Graham, H.N. (1984b) Mate. In: Spiller, G.A., ed., The Methylxanthine Beverages and Foods: Chemistry, Consumption, and Health Effects, New York, Alan R. Liss, pp. 179–183.
- Granberg-Öhman I., Johansson S., Hjerpe A. Sister-chromatid exchanges and chromosomal aberrations in rats treated with phenacetin, phenazone and caffeine. Mutat. Res. 1980;79:13–18. [PubMed: 6448957]
- Grant D.M., Tang B.K., Kalow W. Variability in caffeine metabolism. Clin. Pharmacol. Then. 1983a;33:591–602. [PubMed: 6687705]
- Grant D.M., Tang B.K., Kalow W. Polymorphic N-acetylation of a caffeine metabolite. Clin. Pharmacol. Ther. 1983b;33:355–359. [PubMed: 6825389]
- Grant D.M., Tang B.K., Campbell M.E., Kalow W. Effect of allopurinol on caffeine disposition in man. Br. J. clin. Pharmacol. 1986;21:454–458. [PMC free article: PMC1400934] [PubMed: 3754760]
- Grant D.M., Campbell M.E., Tang B.K., Kalow W. Biotransformation of caffeine by microsomes from human liver Kinetics and inhibition studies. Biochem. Pharmacol. 1987;36:1251–1260. [PubMed: 3593412]
- Greden J.F. Coffee, tea and you. Sciences. 1979;19:6–11.
- Greer S.B. Growth inhibitors and their antagonists as mutagens and antimutagens in Escherichia coli. J. gen. Microbiol. 1958;18:543–564. [PubMed: 13549686]
- Grice H.C. Genotoxicity and carcinogenicity assessment of caffeine and theobromine (Letter to the Editor). Food chem. Toxicol. 1987;25:295. [PubMed: 3679025]
- Griffith, H.W. (1989) Complete Guide to Prescription and Non-prescription Drugs, Los Angeles, The Body Press, pp. 1044–1045.
- Griffiths R.R., Woodson P.P. Caffeine physical dependence: a review of human and laboratory animal studies. Psychopharmacology. 1988;94:437–451. [PubMed: 3131789]
- Grigg G.W. Caffeine-death in Escherichia coli. Mol. gen. Genet. 1968;102:316–335. [PubMed: 4914755]
- Grigg G.W., Stuckey J. The reversible suppression of stationary phase mutation in Escherichia coli by caffeine. Genetics. 1966;53:823–834. [PMC free article: PMC1211061] [PubMed: 5333373]
- Grygiel J.J., Wing L.M.H., Farkas J., Birkett D.J. Effects of allopurinol on theophylline metabolism and clearance. Clin. Pharmacol. Ther. 1979;26:660–667. [PubMed: 498708]
- Guglielmi G.E., Vogt T.F., Tice R.R. Induction of sister chromatid exchanges and inhibition of cellular proliferation in vitro. I. Caffeine. Environ. Mutagenesis. 1982;4:191–200. [PubMed: 7075579]
- Gulati, D.K., Russell, V.S., Hommel, L.M., Poonacha, K.B., Sabharwal, P.S. & Lamb, J.C. (1984) Caffeine: Reproduction and Fertility Assessment in CD-1 Mice When Administered in Drinking Water (NTP-85-097), Research Triangle Park, NC, National Institute of Environmental Health Sciences.
- Györffy, B. (1960) The effect of some chemical mutagens on Xanthomonas phaseoli var. fuscans (Ger.). In: Abteilung Deutsches Akademie Wissenschaft Berlin, Klinisches Medizin, pp. 110–115.
- Halsey, W.D. & Johnston, B., eds (1987) Caffeine. In: Collier’s Encyclopedia, Vol. 5, New York, MacMillan-Collier, p. 110.
- Haughey D.B., Greenberg R., Schaal S.F., Lima J.J. Liquid chromatographic determination of caffeine in biological fluids. J. Chromatogr. 1982;229:387–395. [PubMed: 7096473]
- Haugli F.B., Dove W.F. Mutagenesis and mutant selection in Physarum polycephalum. Mol. gen. Genet. 1972;118:109–124. [PubMed: 4673170]
- Haynes, R.H. & Collins, J.D.B. (1984) The mutagenic potential of caffeine. In: Dews, P.B., ed., Caffeine. Perspectives from Recent Research, Berlin, Springer, pp. 221–238.
- Heddle, J.A. & Bruce, W.R. (1977) Comparison of tests for mutagenicity or carcinogenicity using assays for sperm abnormality, formation of micronuclei, and mutation in Salmonella. In: Hiatt, H.H., Watson, J.D. & Winsten, J.A., eds, Origins of Human Cancer, Cold Spring Harbor, NY, CSH Press, pp. 1549–1557.
- Heinonen, O.P. (1982) Birth Defects and Drugs in Pregnancy, Littleton, MA, Publishing Sciences.
- Heyden S., Fodor J.G. Coffee consumption and fibrocystic breasts: an unlikely association. Can. J. Surg. 1986;29:208–211. [PubMed: 3708462]
- Heyden S., Muhlbaier L.H. Prospective study of ‘fibrocystic breast disease’ and caffeine consumption. Surgery. 1984;96:479–484. [PubMed: 6474353]
- Hindi-Alexander M.C., Zielezny M.A., Montes N., Bullough B., Middleton E. Jr, Rosner D.H., London W.M. Theophylline and fibrocystic breast disease. J. Allergy clin. Immunol. 1985;75:709–715. [PubMed: 4008800]
- Hirsh, K. (1984) Central nervous system pharmacology of the dietary methylxanthines. In: Spiller, G.A., ed., The Methylxanthine Beverages and Foods: Chemistry, Consumption, and Health Effects, New York, Alan R. Liss, pp. 235–301.
- van’t Hoff W. Caffeine in pregnancy. Lancet. 1982;i:1020. [PubMed: 6122833]
- Holliday R. Induced mitotic crossing-over in Ustilago maydis. Genet. Res. 1961;2:231–248.
- Homonnai Z.T., Paz G., Sofer A., Kraicer P.F., Harell A. Effect of caffeine on the motility, viability, oxygen consumption and glycolytic rate of ejaculated human normokinetic and hypokinetic spermatozoa. Int. J. Fertil. 1976;21:163–170. [PubMed: 12119]
- Horwitz, W., ed. (1980a) Coffee and tea, spectrophotometric and chromatographic methods. In: Official Methods of Analysis of the Association of Official Analytical Chemists, 13th ed., Washington DC, Association of the Official Analytical Chemists, p. 234.
- Horwitz, W., ed. (1980b) Coffee and tea, GLC method. In: Official Methods of Analysis of the Association of Official Analytical Chemists, 13th ed., Washington DC, Association of the Official Analytical Chemists, p. 234.
- Horwitz, W, ed. (1980c) Coca bean and its product, HPLC method. In: Official Methods of Analysis of the Association of Official Analytical Chemists, 13th ed., Washington DC, Association of the Official Analytical Chemists, p. 382.
- Hosaka S., Nagayama H., Hirono I. Suppressive effect of caffeine on the development of hepatic tumors induced by 2-acetylaminofluorene in ACI rats. Gann. 1984;75:1058–1061. [PubMed: 6526220]
- Hoshino H., Tanooka H. Caffeine enhances skin tumor induction in mice. Toxicol. Lett. 1979;4:83–85.
- Hostetler K.A., Morrissey R.B., Tarka S.M. Jr, Apgar J.L., Shively C.A. Three-generation reproductive studies of cocoa powder in rats. Food chem. Toxicol. 1990;28:483–490. [PubMed: 2210520]
- Hoy C.A., Salazar E.P., Thompson L.H. Rapid detection of DNA-damaging agents using repair-deficient CHO cells. Mutat. Res. 1984;130:321–332. [PubMed: 6493253]
- Huber, J., Jr (1964) Caffeine. In: Kirk, R.E. & Othmer, D.E, eds, Kirk-Othmer Encyclopedia of Chemical Technology, 2nd ed., Vol. 3, New York, John Wiley & Sons, pp. 911–917.
- Huff, B.B., ed. (1989a) Physicians’ Desk Reference, 43rd, Oradell, NJ, Medical Economics, pp. 407, 415, 756, 2226.
- Huff, B.B., ed. (1989b) Physicians’ Desk Reference for Non-prescription Drugs, 10th ed., Oradell, NJ, Medical Economics, pp. 516, 527, 529, 556.
- Hurst, W.J., Martin, R.A. & Tarka, S.M., Jr (1984) Analytical methods for quantitation of methylxanthines. In: Spiller, G.A., ed., The Methylxanthine Beverages and Foods: Chemistry, Consumption and Health Effects, New York, Alan R. Liss, pp. 17–28.
- IARC (1979a) IARC Monographs on the Evaluation of the Carcinogenic Risk of Chemicals to Humans, Vol. 20, Some Halogenated Hydrocarbons, Lyon, pp. 545–572. [PubMed: 397178]
- IARC (1979b) IARC Monographs on the Evaluation of the Carcinogenic Risk of Chemicals to Humans, Vol. 20, Some Halogenated Hydrocarbons, Lyon, pp. 449–465. [PubMed: 397173]
- IARC (1987) IARC Monographs on the Evaluation of Carcinogenic Risks to Humans, Suppl. 7, Overall Evaluations of Carcinogenicity: An Updating of IARC Monographs Volumes 1 to 42, Lyon. [PubMed: 3482203]
- IARC (1990) IARC Monographs on the Evaluation of Carcinogenic Risks to Humans, Vol. 50, Pharmaceutical Drugs, Lyon, pp. 307–332. [PMC free article: PMC7682314] [PubMed: 2152767]
- Ide T., Anzai K., Andoh T. Enhancement of SV40 transformation by treatment of C3H2K cells with UV light and caffeine I. Combined effect of UV light and caffeine. Virology. 1975;66:568–578. [PubMed: 168688]
- International Coffee Organization (1981) Robusta Coffee: Study of World Production and Consumption (Publ. No. EB 1860810(E)), London.
- International Coffee Organization (1982) United States of America. Coffee Drinking Study, London.
- International Coffee Organization (1989) United States of America. Coffee Drinking Study. Winter 1989 (Rep. PC-585/89), London.
- Iscovich J.M., Iscovich R.B., Howe G., Shiboski S., Kaldor J.M. A case-control study of diet and breast cancer in Argentina. Int. J. Cancer. 1989;44:770–776. [PubMed: 2583858]
- Ishida R., Kozaki M., Takahashi T. Caffeine alone causes DNA damage in Chinese hamster ovary cells. Cell Struct. Function. 1985;10:405–409. [PubMed: 4084980]
- Ishidate, M., Jr (1988) Data Book of Chromosomal Aberration Tests In Vitro, rev. ed., Amsterdam, Elsevier, p. 69.
- Ishii Y., Bender M.A. Caffeine inhibition of prereplication repair of mytomycin C-induced DNA damage in human peripheral lymphocytes. Mutat. Res. 1978;51:419–425. [PubMed: 714073]
- Iyer V.N., Szybalski W. Two simple methods for the detection of chemical mutagens. Appl. Microbiol. 1958;6:23–29. [PMC free article: PMC1057349] [PubMed: 13509650]
- Jalal M.A.F, Collin H.A. Estimation of caffeine, theophylline and theobromine in plant material. New Phytol. 1976;76:277–281.
- Jensen O.M., Wahrendorf J., Knudsen J.B., Sørensen B.L. The Copenhagen case-control study of bladder cancer II. Effect of coffee and other beverages. Int. J. Cancer. 1986;37:651–657. [PubMed: 3699928]
- Jenssen D., Ramel C. Factors affecting the induction of micronuclei at low doses of X-rays, MMS and dimethylnitrosamine in mouse erythroblasts. Mutat. Res. 1978;58:51–65. [PubMed: 714077]
- Jiritano L., Bortolotti A., Gaspari F., Bonati M. Caffeine disposition after oral administration to pregnant rats. Xenobiotica. 1985;15:1045–1051. [PubMed: 4090524]
- Joeres R., Klinker H., Heusler H., Epping J., Zilly W., Richter E. Influence of smoking on caffeine elimination in healthy volunteers and in patients with alcoholic liver cirrhosis. Hepatology. 1988;8:575–579. [PubMed: 3371873]
- Johansson S.L. Carcinogenicity of analgesics: long-term treatment of Sprague-Dawley rats with phenacetin, phenazone, caffeine and paracetamol (acetamidophen). Int. J. Cancer. 1981;27:521–529. [PubMed: 7275356]
- Johnson A.R. Collaborative study of a method for the determination of caffeine in nonalcoholic beverages. J. Assoc, off. anal. Chem. 1967;50:857–858.
- Kada T., Tutikawa K., Sadaie Y. In vitro and host-mediated ‘rec-assay’ procedures for screening chemical mutagens and phloxine, a mutagenic red dye detected. Mutat. Res. 1972;16:165–174. [PubMed: 4342303]
- Kalow W. Pharmacoanthropology: drug metabolism. Fed. Proc. 1984;43:2326–2331. [PubMed: 6714441]
- Kamei K., Matsuda M., Momose A. New sulfur-containing metabolites of caffeine. Chem. pharm. Bull. (Tokyo). 1975;23:683–685.
- Kao F.-T., Puck T.T. Genetics of somatic mammalian cells IX. Quantitation of mutagenesis by physical and chemical agents. J. Cell Physiol. 1969;74:245–258. [PubMed: 5390857]
- Kapke G.F., Franklin R.B. A direct liquid chromatography method for serum caffeine analysis. J. liquid Chromatogr. 1987;10:451–463.
- Kato H. Induction of sister chromatid exchanges by UV light and its inhibition by caffeine. Exp. Cell Res. 1973;82:383–390. [PubMed: 4797252]
- Kaul B.L., Zutshi U. On the production of chromosome breakage in Vicia faba by caffeine. Cytobios. 1973;7:261–264. [PubMed: 4760572]
- Kazi, T. (1985) Determination of caffeine and other purine alkaloids in coffee and tea products by high performance liquid chromatography. In: 11e Colloque Scientifique Internationale sur le Café, Lomé, 1985, Paris, Association Scientifique Internationale du Café, pp. 227–244.
- Kesavan P.C., Trasi S., Ahmad A. Modification of barley seed sensitivity by post-treatment with caffeine I. Effect of post-irradiation heat shock and nature of hydration. Int. J. Radiat. Biol. 1973;24:581–587. [PubMed: 4543565]
- Khanna K.L., Cornish H.H. The effect of daily ingestion of caffeine on the microsomal enzymes of rat liver. Food Cosmet. Toxicol. 1973;11:11–17. [PubMed: 4716126]
- Khanna N.N., Bada H.S., Somani S.M. Use of salivary concentrations in the prediction of serum caffeine and theophylline concentrations in premature infants. J. Pediatr. 1980;96:494–499. [PubMed: 7359248]
- Kihlman B.A. The effect of purine derivatives on chromosomes (Abstract). Hereditas. 1949;35:393.
- Kihlman B.A. Effects of caffeine on the genetic material. Mutat. Res. 1974;26:53–71. [PubMed: 4595676]
- Kihlman, B.A. (1977) Caffeine and Chromosomes, Amsterdam, Elsevier.
- Kihlman B.A., Sturelid S. Enhancement by methylated oxypurines of the frequency of induced chromosomal aberrations III. The effect in combination with X-rays in root tips of Vicia faba. Hereditas. 1975;80:247–254. [PubMed: 1184408]
- Kihlman B.A., Sturelid S., Norlén K., Tidriks D. Caffeine, caffeine derivatives and chromosomal aberrations II. Different responses of Allium root tips and Chinese hamster cells to treatments with caffeine, 8-ethoxycaffeine and 6-methylcoumarin. Hereditas. 1971a;69:35–50. [PubMed: 5173419]
- Kihlman B.A., Norlén K., Sturelid S., Odmark G. Caffeine and 8-ethoxycaffeine produce different types of chromosome-breaking effects depending on the treatment temperature. Mutat. Res. 1971b;12:463–468.
- Kihlman B.A., Sturelid S., Palitti F., Becchetti A. Effect of caffeine, an inhibitor of post-replication repair in mammalian cells, on the frequencies of chromosomal aberrations and sister chromatid exchanges induced by mutagenic agents (Abstract No 36). Mutat. Res. 1977;46:130.
- Kim J., Levin R.E. Influence of caffeine on mitomycin C induced mutagenesis. Microbios. 1986;46:15–20. [PubMed: 3090403]
- Kimmel C.A., Rimmel G.L., White C.G., Grafton T.F., Young J.F., Nelson C.J. Blood flow changes and conceptal development in pregnant rats in response to caffeine. Fundam. appl. Toxicol. 1984;4:240–247. [PubMed: 6724197]
- King M.-T., Beikirch H., Eckhardt K., Gocke E., Wild D. Mutagenicity studies with X-ray-contrast media, analgesics, antipyretics, antirheumatics and some other pharmaceutical drugs in bacterial, Drosophila and mammalian test systems. Mutat. Res. 1979;66:33–43. [PubMed: 106272]
- Klassen R., Stavric B. HPLC separation of theophylline, paraxanthine, theobromine, caffeine and other metabolites in biological fluids. J. liquid Chromatogr. 1983;6:895–906.
- Knoche C., König J. Prenatal toxicity of diphenylpyraline-8-chlorotheophyllinate, having regard to experience with thalidomine and caffeine (Ger.). Arzeimittel-Forsch. 1964;14:415–424. [PubMed: 14238604]
- Knutti R., Rothweiler H., Schlatter C. Effect of pregnancy on the pharmacokinetics of caffeine. Eur. J. clin. Pharmacol. 1981;21:121–126. [PubMed: 7341280]
- Knutti R., Rothweiler H., Schlatter C. The effect of pregnancy on the pharmacokinetics of caffeine. Arch. Toxicol, Suppl. 1982;5:187–192. [PubMed: 6954898]
- Koch A.L., Lamont W.A. The metabolism of methylpurines by Escherichia coli. IL Enzymatic studies. J. biol. Chem. 1956;219:189–201. [PubMed: 13295271]
- Koerting-Keiffer L.E., Mickey G.H. Influence of caffeine on chromosomes (Ger.). Z. Pflanzenzucht. 1969;61:244–251.
- Kolb C.A., Mansfield J.M. Effect of theophylline treatment of mouse B–16 melanoma cells in vitro. Oncology. 1980;37:343–352. [PubMed: 6255390]
- Kotake A.N., Schoeller D.A., Lambert G.H., Baker A.L., Schaffer D.D., Josephs H. The caffeine CO2 breath test: dose response and route of N-demethylation in smokers and nonsmokers. Clin. Pharmacol. Ther. 1982;32:261–269. [PubMed: 6807598]
- Kraft General Foods (1989) Coffee Consumption, White Plains, NY.
- Kreiser W.R., Martin R.A. Jr. High pressure liquid chromatographic determination of theobromine and caffeine in cocoa and chocolate products. J. Assoc. off. anal. Chem. 1978;61:1424–1427. [PubMed: 730647]
- von Kreybig T., Czok G. Teratogenic and mutagenic studies with caffeine in animal experiment (Ger.). Z. Ernährungswiss. 1976;15:64–70. [PubMed: 960793]
- Kubitschek E.H., Bendigkeit H.E. Delay in the appearance of caffeine-induced T5 resistance in Escherichia coli. Genetics. 1958;43:647–661. [PMC free article: PMC1209908] [PubMed: 17247784]
- Kubitschek H.E., Bendigkeit H.E. Mutation in continuous cultures I. Dependence of mutational response upon growth-limiting factors. Mutat. Res. 1964;1:113–120. [PubMed: 14237062]
- Kuhlmann W., Fromme H.-G., Heege E.-M., Ostertag W. The mutagenic action of caffeine in higher organisms. Cancer Res. 1968;28:2375–2389. [PubMed: 4881507]
- Kunze E., Rath G., Graewe T. Effect of phenacetin and caffeine on N-butyl-N-(4-hydroxybutyl)nitrosamine-initiated urothelial carcinogenesis in rats. Urol, int. 1987;42:108–114. [PubMed: 3617238]
- Kuzma J.W., Sokol R.J. Maternal drinking behavior and decreased intrauterine growth. Alcohol. clin. exp. Res. 1982;6:396–402. [PubMed: 6751136]
- Lachance M.P. The pharmacology and toxicology of caffeine. J. Food Saf. 1982;4:71–112.
- Lakhanisky T., Hendrickx B., Mouton R.F. Chemical induction of DNA damage and its repair in T pyriformis. Influence of caffeine, quinacrine and chloroquine (Abstract). Mutat. Res. 1981;85:275.
- Lang T., Degoulet R, Aime F., Fouriaud C., Jacquinet-Salord M.-C, Laprugne J., Main J., Oeconomos J., Phalente J., Prades A. Relation between coffee drinking and blood pressure: analysis of 6,321 subjects in the Paris region. Am. J. Cardiol. 1983;52:1238–1242. [PubMed: 6650411]
- Latini R., Bonati M., Castelli D., Garattini S. Dose-dependent kinetics of caffeine in rats. Toxicol. Lett. 1978;2:267–270.
- Latini R., Bonati M., Marzi E., Tacconi M.T., Sadurska B., Bizzi A. Caffeine disposition and effects in young and one-year old rats. J. Pharm. Pharmacol. 1980;32:596–599. [PubMed: 6106707]
- La Vecchia C., Franceschi S., Parazzini F., Regallo M., Decarli A., Gallus G., Di Pietro S., Tognoni G. Benign breast disease and consumption of beverages containing methylxanthines. J. natl Cancer Inst. 1985;74:995–1000. [PubMed: 3858587]
- Lawson D.H., Jick H., Rothman K.J. Coffee and tea consumption and breast disease. Surgery. 1981;90:801–803. [PubMed: 7302833]
- Ledbetter D.H., Airhart S.D., Nussbaum R.L. Caffeine enhances fragile (X) expression in somatic cell hybrids. Am. J. med. Genet. 1986;23:445–455. [PubMed: 2937299]
- Ledinko N., Evans M. Enhancement of adenovirus transformation of hamster cells by N-methyl-N′-nitro-N-nitrosoguanidine, caffeine, and hydroxylamine. Cancer Res. 1973;33:2936–2938. [PubMed: 4795905]
- Lee S. Chromosome aberrations induced in cultured human cells by caffeine. Jpn. J. Genet. 1971;46:337–344.
- Legator M.S., Zimmering S. Review of the genetic effects of caffeine. J. environ. Sci. Health. 1979;C13:135–188. [PubMed: 224088]
- Lehmann A.R. Effects of caffeine and theophylline on DNA synthesis in untreated and UV-irradiated mammalian cells (Abstract No 15). Mutat. Res. 1973;21:192–193. [PubMed: 4522823]
- Lehmann A.R., Kirk-Bell S. Effects of caffeine and theophylline on DNA synthesis in unirradiated and UV-irradiated mammalian cells. Mutat. Res. 1974;26:73–82. [PubMed: 4522823]
- Lelo A., Miners J.O., Robson R., Birkett D.J. Assessment of caffeine exposure: caffeine content of beverages, caffeine intake, and plasma concentration of methylxanthines. Clin. Pharmacol. Then. 1986;39:54–59. [PubMed: 3943270]
- Leonard T.K., Watson R.R., Mohs M.E. The effects of caffeine on various body systems: a review. J. Am. diet. Assoc. 1987;87:1048–1053. [PubMed: 3301987]
- Levi V., Jacobson E.L., Jacobson M.K. Inhibition of poly(ADP-ribose)polymerase by methylated xanthines and cytokinins. FEBS Lett. 1978;88:144–146. [PubMed: 205432]
- Levine J. Determination of caffeine in coffee products, beverages and tablets. J. Assoc. off. anal. Chem. 1962;45:254–255.
- Lieb M. Enhancement of ultraviolet-induced mutation in bacteria by caffeine. Z Vererbungsl. 1961;92:416–429.
- Linn S., Schoenbaum S.C., Monson R.R., Rosner B., Stubblefield P.G., Ryan K.J. No association between coffee consumption and adverse outcomes of pregnancy. New Engl. J. Med. 1982;306:141–145. [PubMed: 7054656]
- Liwerant I.J., Pereira Da Silva L.H. Comparative mutagenic effects of ethyl methanesulfonate, N-methyl-N′-nitro-N-nitrosoguanidine, ultraviolet radiation and caffeine on Dictyostelium discoideum. Mutat. Res. 1975;33:135–146. [PubMed: 175268]
- Loprieno N., Schüpbach M. On the effect of caffeine on mutation and recombination in Schizosaccharomyces pombe. Mol. gen. Genet. 1971;110:348–354. [PubMed: 5574427]
- Loprieno N., Barale R., Baroncelli S. Genetic effects of caffeine. Mutat. Res. 1974;26:83–87. [PubMed: 4822597]
- Lubin, F., Ron, E., Wax, Y., Funaro, M., Shitrit, A., Black, M. & Modan, B. (1984) Coffee and methylxanthine in benign and malignant breast diseases. In: MacMahon, B. & Sugimura, T., eds, Coffee and Health (Banbury Report 17), Cold Spring Harbor, NY, CSH Press, pp. 177–187.
- Lubin F., Ron E., Wax Y., Black M., Funaro M., Shitrit A. A case-control study of caffeine and methylxanthines in benign breast disease. J. Am. med. Assoc. 1985a;253:2388–2392. [PubMed: 3981766]
- Lubin F., Ron E., Wax Y., Modan B. Coffee and methylxanthines and breast cancer: a case-control study. J. natl Cancer Inst. 1985b;74:569–573. [PubMed: 3856060]
- Lyon M.F., Phillips R.J.S., Searle A.G. A test for mutagenicity of caffeine in mice. Z. Verebungsl. 1962;93:7–13. [PubMed: 14467628]
- Macklin A.W., Szot R.J. Eighteen month oral study of aspirin, phenacetin and caffeine in C57B1/6 mice. Drug Chem. Toxicol. 1980;3:135–163. [PubMed: 7227215]
- Macrae, R. (1985) Nitrogenous components. In: Clarke, R.Y. & Macrae, R., eds, Coffee, Vol. 1, Chemistry, London, Elsevier Applied Science, pp. 115–152.
- Madison B.L., Kozarek W.J., Damo C.P. High-pressure liquid chromatography of caffeine in coffee. J. Assoc. off. anal. Chem. 1976;59:1258–1261. [PubMed: 993180]
- Maher V.M., Quellette L.M., Curren R.D., McCormick J.J. Caffeine enhancement of the cytotoxic and mutagenic effect of ultraviolet irradiation in a xeroderma pigmentosum variant strain of human cells. Biochem. biophys. Res. Comm. 1976;71:228–234. [PubMed: 962915]
- Maickel R., Snodgrass W.R. Physicochemical factors in maternal-fetal distribution of drugs. Toxicol, appl. Pharmacol. 1973;26:218–230. [PubMed: 4751100]
- Marks V., Kelly J.F. Absorption of caffeine from tea, coffee, and Coca Cola. Lancet. 1973;i:827. [PubMed: 4121243]
- Marshall J., Graham S., Swanson M. Caffeine consumption and benign breast disease: a case-control comparison. Am. J. public Health. 1982;72:610–612. [PMC free article: PMC1650117] [PubMed: 7072882]
- Martin T.R., Bracken M.B. The association between low birth weight and caffeine consumption during pregnancy. Am. J. Epidemiol. 1987;126:813–821. [PubMed: 3661529]
- Martin J.B., Annegers J.F., Curb J.D., Heyden S., Howson C., Lee E.S., Lee M. Mortality patterns among hypertensives by reported level of caffeine consumption. Prey. Med. 1988;17:310–320. [PubMed: 3043418]
- Matter B.E., Grauwiler J. Micronuclei in mouse bone-marrow cells. A simple in vivo model for the evaluation of drug-induced chromosomal aberrations. Mutat. Res. 1974;23:239–249. [PubMed: 4365427]
- Mau G., Netter P. Coffee and alcohol consumption Risk factors in pregnancy? (Ger.). Geburtsh. Frauenheilk. 1974;34:1018–1022. [PubMed: 4457384]
- May D.C., Jarboe C.H., VanBakel A.B., Williams W.M. Effects of cimetidine on caffeine disposition in smokers and nonsmokers. Clin. Pharmacol. Ther. 1982;31:656–661. [PubMed: 7075114]
- Mayanna S.M., Jayaram B. Determination of caffeine using sodium N-chloro-p-toluenesulfonamide. Analyst. 1981;106:729–732. [PubMed: 7258658]
- McCall A.L., Millington W.R., Wurtman R.J. Blood-brain barrier transport of caffeine: dose-related restriction of adenine transport. Life Sci. 1982;31:2709–2715. [PubMed: 7154859]
- McCann J., Choi E., Yamasaki E., Ames B.N. Detection of carcinogens as mutagens in the Salmonella/microsome test: assay of 300 chemicals. Proc. natl Acad. Sci. USA. 1975;72:5135–5139. [PMC free article: PMC388891] [PubMed: 1061098]
- McCutheon, G.F. (1969) Caffeine. In: Snell, F.D. & Ettre, L.S., eds, Encyclopedia of Industrial Chemical Analysis, Vol. 8, New York, Interscience, pp. 55–71.
- McElvoy, G.K. (1989) American Health Formulary Service (AHFS) Drug Information, Bethesda, MD, American Society of Hospial Pharmacists, pp. 1179–1181.
- McMillan S., Fox M. Failure of caffeine to influence induced mutation frequencies and the independence of cell killing and mutation induction in V79 Chinese hamster cells. Mutat. Res. 1979;60:91–107. [PubMed: 431556]
- Meatherall R., Ford D. Isocratic liquid chromatographic determination of theophylline, acetaminophen, chloramphenicol, caffeine, anticonvulsants, and barbiturates in serum. Ther Drug Monit. 1988;10:101–115. [PubMed: 3376176]
- Menthe, J. (1985) Caffeine — a commodity in demand. Tea Coffee Trade J., February, 18.
- Merriman R.L., Swanson A., Anders M.W., Sladek N.E. Micro-determination of caffeine in blood by gas chromatography-mass spectrometry. J. Chromatogr. 1978;146:85–90. [PubMed: 670361]
- Minton J.P., Foecking M.K., Webster D.J.T., Matthews R.H. Response of fibrocystic disease to caffeine withdrawal and correlation of cyclic nucleotides with breast disease. Am. J. Obstet. Gynecol. 1979a;135:157–158. [PubMed: 224703]
- Minton J.P., Foecking M.K., Webster D.J.T., Matthews R.H. Caffeine, cyclic nucleotides, and breast disease. Surgery. 1979b;86:105–109. [PubMed: 222001]
- Minton J.P., Abou-Issa H., Reiches N., Roseman J.M. Clinical and biochemical studies on methylxanthine-related fibrocystic breast disease. Surgery. 1981;90:299–304. [PubMed: 7256542]
- Minton J.P., Abou-Issa H., Foecking M.K., Sriram M.G. Caffeine and unsaturated fat diet significantly promotes DMBA-induced breast cancer in rats. Cancer. 1983;51:1249–1253. [PubMed: 6402286]
- Mirvish S.S., Cardesa A., Wallcave L., Shubik P. Induction of mouse lung adenomas by amines or ureas plus nitrite and by N-nitroso compounds: effects of ascorbate, gallic acid, thiocyanate, and caffeine. J. natl Cancer Inst. 1975;55:633–636. [PubMed: 1159840]
- Mitchell M.C., Hoyumpa A.M., Schenker S., Johnson R.F., Nichols S., Patwardhan R.V. Inhibition of caffeine elimination by short-term ethanol administration. J. Lab. clin. Med. 1983;101:826–834. [PubMed: 6854123]
- Mitoma C., Lombrozo L., LeValley S.E., Dehn F. Nature of the effect of caffeine on the drug-metabolizing enzymes. Arch. Biochem. Biophys. 1969;134:434–441. [PubMed: 5354771]
- Mittler S., Mittler J.E., Tonetti A.M., Szymczak M.E. The effect of caffeine on chromosome loss and nondisjunction in Drosophila. Mutat. Res. 1967a;4:708–710. [PubMed: 6054965]
- Mittler S., Mittler J.E., Owens S.L. Loss of chromosomes and nondisjunction induced by caffeine in Drosophila. Nature. 1967b;214:424. [PubMed: 6032860]
- Mitznegg P., Heim F., Hach B., Säbel M. The effect of ageing, caffeine-treatment, and ionising-radiation on nucleic acid synthesis in the mouse liver. Life Sci. 1971;10:1281–1292. [PubMed: 5144388]
- Moffat, A.C., ed. (1986) Clarke’s Isolation and Identification of Drugs, 2nd ed., London, The Pharmaceutical Press, pp. 420–422.
- Mohr U., Althoff J., Ketkar M.B., Conradt P., Morgareidge K. The influence of caffeine on tumour incidence in Sprague-Dawley rats. Food chem. Toxicol. 1984;22:377–382. [PubMed: 6539287]
- Moores R.G., Campbell H.A. Determination of theobromine and caffeine in cocoa materials. Anal. Chem. 1948;20:40–47.
- Morgan W.F., Cleaver J.E. 3-Aminobenzamide synergistically increases sister-chromatid exchanges in cells exposed to methyl methanesulfonate but not to ultraviolet light. Mutat. Res. 1982;104:361–366. [PubMed: 6287250]
- Morgan K.J., Stults V.J., Zabik M.E. Amount and dietary sources of caffeine and saccharin intake by individuals aged 5 to 18 years. Regul. Toxicol. Pharmacol. 1982;2:296–307. [PubMed: 7185096]
- Mortelmans K., Haworth S., Lawlor T., Speck W., Tainer B., Zeiger E. 1986Salmonella mutagenicity tests: II. Results from the testing of 270 chemicals Environ. Mutagenesis 8(Suppl. 7)1–119. [PubMed: 3516675]
- Muir K.T., Kunitani M., Riegelman S. Improved high-performance liquid chromatographic assay for theophylline in plasma and saliva in the presence of caffeine and its metabolites and comparisons with three other assays. J. Chromatogr. 1982;231:73–82. [PubMed: 7119067]
- Müller W.-U., Streffer C., Wurm R. Supraadditive formation of micronuclei in preimplantation mouse embryos in vitro after combined treatment with X-rays and caffeine. Teratog. Carcinog. Mutagenesis. 1985;5:123–131. [PubMed: 2859666]
- Mulvihill J.J. Caffeine as teratogen and mutagen. Teratology. 1973;8:69–72. [PubMed: 4737491]
- Muñoz L.M., Lönnerdal B., Keen C.L., Dewey K.G. Coffee consumption as a factor in iron deficiency anemia among pregnant women and their infants in Costa Rica. Am. J. clin. Nutr. 1988;48:645–651. [PubMed: 3414579]
- Murata T., Murakami A. Induction of dominant lethal mutation by alkylating agents in germ cells of the silkworm, Bombyx mori (Abstract No 14). Mutat. Res. 1976;38:343–344. [PubMed: 972631]
- Murphy T.L., McIvor C., Yap A., Cooksley W.G.E., Haliiday J.W., Powell L.W. The effect of smoking on caffeine elimination: implications for its use as a semiquantitative test of liver function. Clin. exp. Pharmacol. Physiol. 1988;15:9–13. [PubMed: 2482799]
- Myers M.G. Effects of caffeine on blood pressure. Arch. intern. Med. 1988;148:1189–1193. [PubMed: 3284502]
- Myers M.G., Harris L., Leenen F.H.H., Grant D.M. Caffeine as a possible cause of ventricular arrhythmias during the healing phase of acute myocardial infarction. Am. J. Cardiol. 1987;59:1024–1028. [PubMed: 2437789]
- Nakanishi Y., Schneider E.L. In vivo sister-chromatid exchange: a sensitive measure of DNA damage. Mutat. Res. 1979;60:329–337. [PubMed: 113672]
- Nakanishi K., Fukushima S., Shibata M., Shirai T., Ogiso T., Ito N. Effect of phenacetin and caffeine on the urinary bladder of rats treated with N-butyl-N-(4-hydroxybutyl)nitrosamine. Gann. 1978;69:395–400. [PubMed: 669140]
- Nakanishi K., Hirose M., Ogiso T., Hasegawa R., Arai M., Ito N. Effects of sodium saccharin and caffeine on the urinary bladder of rats treated with N-butyl-N-(4-hydroxybutyl)nitrosamine. Gann. 1980;71:490–500. [PubMed: 7429086]
- Nakazawa K., Tanaka H., Arima M. The effect of caffeine ingestion on pharmacokinetics of caffeine and its metabolites after a single administration in pregnant rats. J. Pharmacobio-Dyn. 1985;8:151–160. [PubMed: 4009407]
- Nash J., Persaud T.V.N. Reproductive and teratological risks of caffeine. Anat. Anz. (Jena). 1988;167:265–270. [PubMed: 3066235]
- Natarajan A.T., Csukás I., van Zeeland A.A. Contribution of incorporated 5-bromodeoxyuridine in DNA to the frequencies of sister-chromatid exchanges induced by inhibitors of poly-(ADP-ribose)-polymerase. Mutat. Res. 1981;84:125–132. [PubMed: 7199115]
- National Research Council (1977a) Drinking Water and Health, Washington DC, National Academy of Sciences.
- National Research Council (1977b) Estimating Distribution of Daily Intakes of Caffeine. Committee on GRAS List Survey — Phase III Food and Nutrition Board, Washington DC, National Academy of Sciences.
- National Research Council (1981) Food Chemicals Codex, 3rd ed., Washington DC, National Academy Press, p. 44.
- National Research Council (1989) Diet and Health — Implications for Reducing Chronic Disease Risk, Washington DC, National Academy Press, pp. 465–471. [PubMed: 25032333]
- National Soft Drink Association (1982) What’s in Soft Drinks, 2nd ed., Washington DC.
- National Soft Drink Association (1986) Sales Survey of the Soft Drink Industry, Washington DC.
- Neims A.H., Bailey J., Aldridge A. 1979Disposition of caffeine during and after pregnancy (Abstract) Clin. Res. 27236A.
- Nelson M.M., Forfar J.O. Associations between drugs administered during pregnancy and congenital abnormalities of the fetus. Br. med. J. 1971;i:523–527. [PMC free article: PMC1795296] [PubMed: 4396080]
- Nesterov M., Kucherya L.A., Zaval’nyuk R.G., Alibaeva T.D. Improvement of caffeine synthesis (Russ.). Khim.-Farm. Zh. 1985;19:1389–1390.
- Newton R., Broughton L.J., Lind M.J., Morrison P.J., Rogers H.J., Bradbrook I.D. Plasma and salivary pharmacokinetics of caffeine in man. Eur. J. clin. Pharmacol. 1981;21:45–52. [PubMed: 7333346]
- Nishimura H., Nakai K. Congenital malformations in offspring of mice treated with caffeine. Proc. Soc. exp. biol. Med. 1960;104:140–142. [PubMed: 14427313]
- Nolen, G.A. (1988) The developmental toxicology of caffeine. In: Kalter, H., ed., Issues and Reviews in Teratology, New York, Plenum Press, pp. 305–350.
- Nomura T. Diminution of tumorigenesis initiated by 4-nitroquinoline-1-oxide by post-treatment with caffeine in mice. Nature. 1976;260:547–549. [PubMed: 817208]
- Nomura T. Timing of chemically induced neoplasia in mice revealed by the antineoplastic action of caffeine. Cancer Res. 1980;40:1332–1340. [PubMed: 6766806]
- Nomura T. Comparative inhibiting effects of methylxanthines on urethan-induced tumors, malformations, and presumed somatic mutations in mice. Cancer Res. 1983;43:1342–1346. [PubMed: 6825104]
- Novick A., Szilard L. Experiments on spontaneous and chemically induced mutations of bacteria growing in the chemostat. Cold Spring Harbor Symp. Quant. Biol. 1951;16:337–343. [PubMed: 14942748]
- O’Connell S.E., Zurzola F.J. Rapid quantitative liquid chromatographic determination of caffeine levels in plasma after oral dosing. J. pharm. Sci. 1984;73:1009–1011. [PubMed: 6470942]
- Odenheimer D.J., Zunzunegui M.V., King M.C., Shipler C.P., Friedman G.D. Risk factors for benign breast disease: a case-control study of discordant twins. Am. J. Epidemiol. 1984;120:565–571. [PubMed: 6540984]
- Osiecka R. Effect of the treatment-temperature on induction of chromosome aberrations by caffeine in Vicia faba. Genet. Pol. 1976;17:159–163.
- Ostertag W. Caffeine and theophylline mutagenicity in cell and leucocyte cultures of man (Ger.). Mutat. Res. 1966;3:249–267. [PubMed: 5962398]
- Ostertag W., Haake J. The mutagenicity in Drosophila melanogaster of caffeine and other compounds which produce chromosome breakage in human cells in culture. Z. Vererbungsl. 1966;98:299–308.
- Ostertag W., Duisberg E., Stürmann M. The mutagenic activity of caffeine in man. Mutat. Res. 1965;2:293–296. [PubMed: 5878259]
- Painter R.B. Effect of caffeine on DNA synthesis in irradiated and unirradiated mammalian cells. J. mol. Biol. 1980;143:289–301. [PubMed: 7218356]
- Palitti F., Becchetti A. Effect of caffeine on sister chromatid exchanges and chromosomal aberrations induced by mutagens in Chinese hamster cells. Mutat. Res. 1977;45:157–159. [PubMed: 917034]
- Palitti F., Rizzoni M., Gatti M., Olivieri G. Chromosomal aberrations induced by caffeine in endoreduplicated Chinese hamster cells. Mutat. Res. 1974;26:145–150. [PubMed: 4822595]
- Palm P.E., Arnold E.P., Rachwall P.C., Leyczek J.C., Teague K.W., Kensler C.J. Evaluation of the teratogenic potential of fresh-brewed coffee and caffeine in the rat. Toxicol. appl. Pharmacol. 1978;44:1–16. [PubMed: 675682]
- Panigrahi G.B., Rao A.R. Influence of caffeine on arecoline-induced SCE in mouse bone-marrow cells in vivo. Mutat. Res. 1983;122:347–353. [PubMed: 6656817]
- Pant G.S., Kamada N., Tanaka R. Sister chromatid exchanges in peripheral lymphocytes of atomic bomb survivors and of normal individuals exposed to radiation and chemical agents. Hiroshima J. med. Sci. 1976;25:99–105. [PubMed: 1026669]
- Pao, E.M., Fleming, K.H., Guenther, P.M. & Mickle, S.J. (1982) Foods Commonly Eaten by Individuals: Amount Per Day and Per Eating Occasion (Home Economics Research Report No. 44), Hyattsville, MD, US Department of Agriculture.
- Papadoyannis I.N., Caddy B. Application of high-performance liquid chromatography for the simultaneous determination of morphine, codeine and caffeine. Microchem. J. 1987;36:182–191.
- Parazzini F., La Vecchia C., Riundi R., Pampallona S., Regallo M., Scanni A. Methylxanthine, alcohol-free diet and fibrocystic breast disease: a factorial clinical trial. Surgery. 1986;99:576–580. [PubMed: 3518107]
- Paribok V.P., Kassinova G.V., Bandas E.L. Effects of repair inhibitors on the reversion frequency in the polyauxotrophic strain of Escherichia coli during ultraviolet light radiation (Russ.). Tsitologiya. 1967;9:1496–1502. [PubMed: 4876362]
- Parry J.M., Sharp D., Tippins R.S., Parry E.M. Radiation-induced mitotic and meiotic aneuploidy in the yeast Saccharomyces cerevisiae. Mutat. Res. 1979;61:37–55. [PubMed: 381905]
- Parsons W.D., Neims A.H. Effect of smoking on caffeine clearance. Clin. Pharmacol. Ther. 1978;24:40–45. [PubMed: 657717]
- Parsons W.D., Neims A.H. Prolonged half-life of caffeine in healthy term newborn infants. J. Pediatr. 1981;98:640–641. [PubMed: 7205496]
- Parsons W.D., Pelletier J.G. Delayed elimination of caffeine by women in the last 2 weeks of pregnancy. Can. med. Assoc. J. 1982;127:377–380. [PMC free article: PMC1861995] [PubMed: 7104915]
- Parsons W.D., Aranda J.V., Neims A.H. Elimination of transplacentally acquired caffeine in fullterm neonates (Abstract No 195). Pediatr. Res. 1976;10:333.
- Patwardhan R.V., Desmond P.V., Johnson R.F., Schenker S. Impaired elimination of caffeine by oral contraceptive steroids. J. Lab. clin. Med. 1980;95:603–608. [PubMed: 7359014]
- Periti M., Salvaggio A., Quaglia G., Di Marzio L. Coffee consumption and blood pressure: an Italian study. Clin. Sci. 1987;72:443–447. [PubMed: 3829593]
- Perry, D.L., Chuang, C.C., Jungclaus, G.A. & Warner, J.S. (1979) Identification of Organic Compounds in Industrial Effluent Discharges (EPA-600/4-79-016), Athens, GA, US Environmental Protection Agency.
- Petrek J.A., Sandberg W.A., Cole M.N., Silberman M.S., Collins D.C. The inhibitory effect of caffeine on hormone-induced rat breast cancer. Cancer. 1985;56:1977–1981. [PubMed: 4027928]
- Phelps H.M., Phelps C.E. Caffeine ingestion and breast cancer. A negative correlation. Cancer. 1988;61:1051–1054. [PubMed: 3276391]
- Pienta, R.J. (1980) Evaluation and relevance of the Syrian hamster embryo cell system. In: Williams, G.M., Kroes, R., Waaijers, H.W. & Van de Poll, K.W., eds, The Predictive Value of Short-term Screening Tests in Carcinogenicity, Amsterdam, Elsevier, pp. 149–169.
- Pike M.C., Bernstein L. Statistical errors invalidate conclusions in ‘caffeine and unsaturated fat diet significantly promotes DMBA-induced breast cancer in rats’ Cancer. 1985;55:1855–1858. [PubMed: 3919918]
- Pozner J., Papatestas A.E., Fagerstrom R., Schwartz I., Saevitz J., Feinberg M., Aufses A.H. Jr. Association of tumor differentiation with caffeine and coffee intake in women with breast cancer. Surgery. 1986;100:482–488. [PubMed: 3738767]
- Prineas R.J., Jacobs D.R. Jr, Crow R.S., Blackburn H. Coffee, tea and VPB. J. chron. Dis. 1980;33:67–72. [PubMed: 6153390]
- Rafter J.J., Nilsson L. Involvement of the intestinal microflora in the formation of sulfur-containing metabolites of caffeine. Xenobiotica. 1981;11:771–778. [PubMed: 7336758]
- Rajaraman R., Faulkner G. Reverse transformation of Chinese hamster ovary cells by methyl xanthines. Exp. Cell Res. 1984;154:342–356. [PubMed: 6090184]
- Rall, T.W. (1985) The methylxanthines. In: Gilman, A.G., Goodman, L.S., Rall, T.W. & Murad, F., eds, Goodman and Gilman’s The Pharmacological Basis of Therapeutics, 7th ed., New York, MacMillan, pp. 589–603.
- Rao G.S., Khanna K.L., Cornish H.H. Identification of two new metabolites of caffeine in the rat urine. Experientia. 1973;29:953–955. [PubMed: 4733311]
- Reel, J.R., Wolkowski-Tyl, R., Lawton, A.D., Feldman, D.B. & Lamb, J.C. (1984) Caffeine: Reproduction and Fertility Assessment in CD-1 Mice When Administered in the Drinking Water (NTP-84-158), Research Triangle Park, NC, National Institute of Environmental Health Sciences.
- Reguly M.L., Marques E.K. Effect of caffeine on the radioresistance and radiosensitivity of Drosophila melanogaster. Rev. Brasil. Genet. 1988;11:1–11.
- Reid S.J., Good T.J. Use of chromatographic mode sequencing for sample preparation in the analysis of caffeine and theobromine from beverages. J. agric. Food Chem. 1982;30:775–778.
- Renner H.W. Sister chromatid exchanges induced by methylxanthines contained in coffee, tea and cocoa. Experientia. 1982;38:600. [PubMed: 7095098]
- Renner E., Wietholtz H., Huguenin P., Arnaud M.J., Preisig R. Caffeine: a model compound for measuring liver function. Hepatology. 1984;4:38–46. [PubMed: 6420303]
- Reynolds, J.E.F., ed. (1989) Martindale. The Extra Pharmacopoeia, 29th ed., London, The Pharmaceutical Press, pp. 1522–1524.
- Richardson C.L., Grant A.D., Schulman G.E. The interaction of caffeine and other xanthine analogs with DNA as measured by competitive fluorescence polarization (Abstract No AC-5). Environ. Mutagenesis. 1981;3:343.
- Riechert M. Micromethod for the determination of caffeine and theophylline allowing direct application of biological fluids to thin-layer chromatography plates. J. Chromatogr. 1978;146:175–180. [PubMed: 670355]
- Roberts J.J. The repair of DNA modified by cytotoxic, mutagenic, and carcinogenic chemicals. Adv. Radiat. Biol. 1978;7:211–436.
- Roberts, J.J. (1984) Mechanism of potentiation by caffeine of genotoxic damage induced by physical and chemical agents: possible relevance to carcinogenesis. In: Dews, P.B., ed., Caffeine. Perspectives from Recent Research, Berlin, Springer, pp. 239–253.
- Robertson, D. & Curatolo, P.W. (1984) The cardiovascular effects of caffeine. In: Dews, P.B., ed.s Caffeine. Perspectives from Recent Research, Berlin, Springer, pp. 77–85.
- Robertson D., Frölich J.C., Carr R.K., Watson J.T., Hollifield J.W., Shand D.G., Oates J.A. Effects of caffeine on plasma renin activity, catecholamines and blood pressure. New Engl. J. Med. 1978;298:181–186. [PubMed: 339084]
- Robertson D., Wade D., Workman R., Woosley R.L., Oates J.A. Tolerance to the humoral and hemodynamic effects of caffeine in man. J. clin. Invest. 1981;67:1111–1117. [PMC free article: PMC370671] [PubMed: 7009653]
- Rohan T.E., McMichael A.J. Methylxanthines and breast cancer. Int. J. Cancer. 1988;41:390–393. [PubMed: 3346103]
- Rohan T.E., Cook M.G., McMichael A.J. Methylxanthines and benign proliferative epithelial disorders of the breast in women. Int. J. Epidemiol. 1989;18:626–633. [PubMed: 2681017]
- Röhrborn, G. (1972) Mutagenicity studies on mouse after chronic administration of caffeine (Ger.). Z. Emährungswiss., Suppl. 14, 54–67. [PubMed: 4509625]
- Röhrborn G., Buckel U. Investigations on the frequency of chromosome aberrations in bone marrow cells of Chinese hamster after simultaneous application of caffeine and cyclophosphamide. Hum. Genet. 1976;33:113–119. [PubMed: 939563]
- Rosenberg L., Mitchell A.A., Shapiro S., Slone D. Selected birth defects in relation to caffeine-containing beverages. J. Am. med. Assoc. 1982;247:1429–1432. [PubMed: 7035697]
- Rosenkranz H.S., Ennever F.K. Genotoxicity and carcinogenicity assessment of caffeine and theobromine (Letter to the Editor). Food chem. Toxicol. 1987;25:795–796. [PubMed: 3679025]
- Rothwell K. Dose-related inhibition of chemical carcinogenesis in mouse skin by caffeine. Nature. 1974;252:69–70. [PubMed: 4427686]
- Roulland-Dussoix D. Degradation of ultraviolet-irradiated lambda bacteriophage DNA by the host cell (Fr.). Mutat. Res. 1967;4:241–252. [PubMed: 4860998]
- Routh J.I., Shane N.A., Arredondo E.G., Paul W.D. Determination of caffeine in serum and urine. Clin. Chem. 1969;15:661–668. [PubMed: 5808449]
- Ryu J.E. Caffeine in human milk and in serum of breast-fed infants. Dev. Pharmacol. Ther. 1985;8:329–337. [PubMed: 4075932]
- Sacks L.E., Mihara K. Induction at high frequency of a unique phenotypic class of Bacillus subtilis mutants by methylxanthines. Mutat. Res. 1983;117:55–65. [PubMed: 6403857]
- Sandlie L., Solberg K., Kleppe K. The effect of caffeine on cell growth and metabolism of thymidine in Escherichia coli. Mutat. Res. 1980;73:29–41. [PubMed: 7019679]
- Sasaki M.S. Sister chromatid exchange and chromatid interchange as possible manifestation of different DNA repair processes. Nature. 1977;269:623–625. [PubMed: 917113]
- Sasaki M.S., Tonomura A. A high susceptibility of Fanconi’s anemia to chromosome breakage by DNA cross-linking agents. Cancer Res. 1973;33:1829–1836. [PubMed: 4352739]
- Sato C., Izumi N. Mechanism of increased hepatotoxicity of acetaminophen by the simultaneous administration of caffeine in the rat. J. Pharmacol. exp. Ther. 1989;248:1243–1247. [PubMed: 2703973]
- Schairer C., Brinton L.A., Hoover R.N. Methylxanthines and benign breast disease. Am. J. Epidemiol. 1986;124:603–611. [PubMed: 3752054]
- Schairer C., Brinton L.A., Hoover R.N. Methylxanthines and breast cancer. Int. J. Cancer. 1987;40:469–473. [PubMed: 3117709]
- Schöneich J., Michaelis A., Rieger R. Caffeine and chemical induction of chromatid aberrations in Vicia faba and ascites tumours of the mouse (Ger.). Biol Zentralbl. 1970;88:49–63.
- Schrieber G.B., Maffeo C.E., Robins M., Masters M.N., Bond A.P. Measurement of coffee and caffeine intake: implications for epidemiologic research. Prev. Med. 1988;17:280–294. [PubMed: 3405985]
- Scott N.R., Chakraborty J., Marks V. Urinary metabolites of caffeine in pregnant women. Br. J. clin. Pharmacol. 1986;22:475–478. [PMC free article: PMC1401148] [PubMed: 3768258]
- Senanayake U.M., Wijesekera R.O.B. A rapid micro-method for the separation, identification and estimation of the purine bases: caffeine, theobromine and theophylline. J. Chromatogr. 1968;32:75–86. [PubMed: 4297182]
- Senanayake U.M., Wijesekera R.O.B. Theobromine and caffeine content of the cocoa bean during its growth. J. Sci. Food Agric. 1971;22:262–263.
- Shakarnis V.F. A comparative study on the action of caffeine on the X-chromosome nondisjunction and recessive sex-linked lethal mutation of different Drosophila melanogaster stocks (Russ.). Genetica. 1970;6:83–87.
- Shankel D.M. ‘Mutational synergism’ of ultraviolet light and caffeine in Escherichia coli. J. Bacteriol. 1962;84:410–415. [PMC free article: PMC277891] [PubMed: 13976769]
- Shirlow M.J. Patterns of caffeine consumption. Human Nutr. appl. Nutr. 1983;37A:307–313. [PubMed: 6643125]
- Shively, C.A. & Tarka, S.M., Jr (1984) Methylxanthine composition and consumption patterns of cocoa and chocolate products. In: Spiller, G.A., ed., The Methylxanthine Beverages and Foods: Chemistry, Consumption, and Health Effects, New York, Alan R. Liss, pp. 149–178.
- Singh R.N., Kashyap A.K. Induction of mutations in the blue-green alga Plectonema boryanum Gomont. Mutat. Res. 1977;43:37–44.
- Singh K.P., Saxena A.K., Srivastava S.N., Shanker R. Effect of caffeine (1,3,7-trimethylxanthine) on bone marrow cells of mice. Indian J. exp. Biol. 1984;22:608–611. [PubMed: 6534850]
- Sleigh M.J., Grigg G.W. Induction of local denaturation in DNA in vitro by phleomycin and caffeine. FEBS Lett. 1974;39:35–38. [PubMed: 4277535]
- Smeets D., Verhagen A., Hustinx T. Familial and individual variation in chromosome fragility. Mutat. Res. 1989;212:223–229. [PubMed: 2499778]
- Smith S.E., McElhatton P.R., Sullivan F.M. Effects of administering caffeine to pregnant rats either as a single daily dose or as divided doses four times a day. Food chem. Toxicol. 1987;25:125–133. [PubMed: 3557234]
- Smith D.F., MacGregor J.T., Hiatt R.A., Hooper N.K., Wehr C.M., Peters B., Goldman L.R., Yuan L.A., Smith P.A., Becker C.E. Micronucleated erythrocytes as an index of cytogenetic damage in humans: demographic and dietary factors associated with micronucleated erythrocytes in splenectomized subjects. Cancer Res. 1990;50:5049–5054. [PubMed: 2379170]
- Smith-Sonneborn J. Age-correlated effects of caffeine on non-irradiated and UV-irradiated Paramecium aurelia. J. Gerontol. 1974;29:256–260. [PubMed: 4821841]
- Smits P., Pieters G., Thien T. The role of epinephrine in the circulatory effects of coffee. Clin. Pharmacol. Ther. 1986;40:431–437. [PubMed: 3530587]
- Sobotka T.J., Spaid S.L., Brodie R.E. Neurobehavioral teratology of caffeine exposure in rats. Neurotoxicology. 1979;1:403–416.
- Solberg K.A., Øvrebø S., Kleppe R.K., Kleppe K. Effect of caffeine on DNA polymerase I from Escherichia coli. Studies in vitro and in vivo. Mutat. Res. 1978;51:1–10. [PubMed: 353536]
- Somani S.M., Khanna N.N., Bada H.S. Caffeine and theophylline: serum/CSF correlation in premature infants. J. Pediatr. 1980;96:1091–1093. [PubMed: 7189555]
- Somorin O. Spectrophotometric determination of caffeine in Nigerian kola nuts. J. Food Sci. 1973;38:911–912.
- Somorin O. Caffeine distribution in C acuminata, T. cacao and C. arabica. J. Food Sci. 1974;39:1055–1056.
- Speit G. The relationship between the induction of SCEs and mutations in Chinese hamster cells. I. Experiments with hydrogen peroxide and caffeine. Mutat. Res. 1986;174:21–26. [PubMed: 3702903]
- Spindle A., Wu K. Development and cytogenetic effects of caffeine on mouse blastocysts, alone or in combination with benzo[a]pyrene. Teratology. 1985;32:213–218. [PubMed: 4049279]
- Srinivasan A., Reddy E.P., Sarma P.S., Schultz R.A. Inhibition of RNA-dependent DNA polymerase activity of oncornaviruses by caffeine. Biochem. biophys. Res. Commun. 1979;91:239–246. [PubMed: 92987]
- Stabilimento Farmaceutico ‘Cau. G. Testa’ (1989) Caffeine Production, Albenga.
- Stanovnik B., Mirtič T., Koren B., Tišler M., Belčič B. Methylation of some oxopurines and 1,2,4-triazoles with N,N-dimethylformamide dimethyl acetal. Vestn. Slov. Kem. Drus. 1982;29:331–343.
- Statland B.E., Demas T.J. Serum caffeine half-lives. Healthy subjects vs patients having alcoholic hepatic disease. Am. J. clin. Pathol. 1980;73:390–393. [PubMed: 7361718]
- Statland B.E., Demas T.J., Danis M. Caffeine accumulation associated with alcoholic liver disease. New Engl. J. Med. 1976;295:110–111. [PubMed: 1272309]
- Stavric B. Methylxanthines: toxicity to humans 2. Caffeine. Food chem. Toxicol. 1988;26:645–662. [PubMed: 3053369]
- Stavric B., Klassen R. Automated high-performance liquid chromatographic assay for monitoring caffeine and its metabolites in biological fluids of monkeys consuming caffeine. J. Chromatogr. 1984;310:107–118. [PubMed: 6501507]
- Stavric B., Klassen R. Caffeine content in colas from New York State and Ontario. J. Food Saf. 1987;8:179–185.
- Stavric B., Klassen R., Watkinson B., Karpinski K., Stapley R., Fried P. Variability in caffeine consumption from coffee and tea: possible significance for epidemiological studies. Food chem. Toxicol. 1988;26:111–118. [PubMed: 3366410]
- Strubelt O. Toxicity of coffee and caffeine (Ger.). Dtsch. med. Wochenschr. 1987;112:852–858. [PubMed: 3556132]
- Sturelid S. Enhancement by caffeine of cell killing and chromosome damage in Chinese hamster cells treated with thiotepa. Hereditas. 1976;84:157–162. [PubMed: 402344]
- Sutherland D.J., McPherson D.D., Renton K.W., Spencer C.A., Montague T.J. The effect of caffeine on cardiac rate, rhythm, and ventricular repolarization Analysis of 18 normal subjects and 18 patients with primary ventricular dysrhythmia. Chest. 1985;87:319–324. [PubMed: 3971755]
- Sved S., Wilson D.L. Simultaneous assay of the methylxanthine metabolites of caffeine in plasma by high performance liquid chromatography. Res. Commun, chem. Pathol. Pharmacol. 1977;17:319–331. [PubMed: 877416]
- Swenberg, J.A. (1981) Utilization of the alkaline elution assay as a short-term test for chemical carcinogens. In: Stich, H.F. & San, R.H.C., eds, Short-term Tests for Chemical Carcinogens, New York, Springer, pp. 48–58.
- Swietlin′ska Z. High frequency of chromosome aberrations induced by DEB with caffeine posttreatment in Vicia faba var Minor. Mol. gen. Genet. 1971;112:87–90.
- Swietlin′ska Z., Zaborowska D., Zuk J. Induction of chromosome aberrations by diepoxybutane and caffeine in root meristems and germinating seeds of Vicia faba. Mutat. Res. 1973;17:199–205.
- Takayama S., Kuwabara N. Long-term study on the effect of caffeine in Wistar rats. Gann. 1982;73:365–371. [PubMed: 7129001]
- Tanaka H., Nakazawa K., Arima M., Iwasaki S. Caffeine and its dimethylxanthines in fetal cerebral development in rat. Brain Dev. 1984;6:355–361. [PubMed: 6496872]
- Tang B.K., Grant D.M., Kalow W. Isolation and identification of 5-acetylamino-6-formylamino-3-methyluracil as a major metabolite of caffeine in man. Drug Metab. Dispos. 1983;11:218–220. [PubMed: 6135579]
- Tarka S.M. Jr. The toxicology of cocoa and dimethylxanthines: a review of the literature. CRC crit. Rev. Toxicol. 1982;9:275–312. [PubMed: 6765610]
- Thayer P.S., Kensler C.J. Genetic tests in mice of caffeine alone and in combination with mutagens. Toxicol. appl. Pharmacol. 1973;25:157–168. [PubMed: 4715480]
- Thayer P.S., Palm P.E. A current assessment of the mutagenic and teratogenic effects of caffeine. CRC crit. Rev. Toxicol. 1975;3:345–369. [PubMed: 1097193]
- Thayer P.S., Himmelfarb P., Liss R.H., Carlson B.L. Continuous exposure of HeLa cells to caffeine. Mutat. Res. 1971;12:197–203. [PubMed: 5094807]
- Theiss J.C., Shimkin M.B. Inhibiting effect of caffeine on spontaneous and urethan-induced lung tumors in strain A mice. Cancer Res. 1978;38:1757–1761. [PubMed: 647685]
- Thithapandha A., Chaturapit S., Limlomwongse L., Sobhon P. The effects of xanthines on mouse liver cell. Arch. Biochem. Biophys. 1974;161:178–186.
- Timbie D.J., Sechrist L., Keeney P.G. Application of high-pressure liquid chromatography to the study of variables affecting theobromine and caffeine concentrations in cocoa beans. J. Food Sci. 1978;43:560–565.
- Timson J. Caffeine. Mutat. Res. 1977;47:1–52. [PubMed: 342928]
- Tin A.A., Somani S.M., Bada H.S., Khanna N.N. Caffeine, theophylline and theobromine determinations in serum, saliva and spinal fluid. J. anal. Toxicol. 1979;3:26–29.
- Tobias D.Y. Current Methods of Caffeine Determination: Review of the Literature, 1975–1980. FDA By Lines. 1982;3:129–156.
- Tohda H., Oikawa A. Characterization of the enhancing effect of caffeine on sister-chromatid exchanges induced by ultraviolet radiation in excision-proficient xeroderma pigmentosum lymphoblastoid cells. Mutat. Res. 1988;201:1–8. [PubMed: 2843764]
- Tohnai I., Oka T., Ohno Y. 1984A case-control study on cleft lip and/or palate: maternal dietary practices in early pregnancy (Abstract) Teratology 3023A.
- Tornaletti S., Russo P., Parodi S., Pedrini A.M. Studies on DNA binding of caffeine and derivatives: evidence of intercalation by DNA-unwinding experiments. Biochem. biophys. Acta. 1989;1007:112–115. [PubMed: 2909237]
- Traub A.I., Earnshaw J.C., Brannigan P.D., Thompson W. A critical assessment of the response to caffeine of human sperm motility. Fertil. Steril. 1982;37:436–437. [PubMed: 7060792]
- Trosko J.E., Chu E.H.Y. Effects of caffeine on the induction of mutations in Chinese hamster cells by ultraviolet light. Mutat. Res. 1971;12:337–340. [PubMed: 5563947]
- Ts’o P.O., Lu P. Interaction of nucleic acids. I. Physical binding of thymidine, adenine, steroids, and aromatic hydrocarbons to nucleic acids. Proc. natl Acad. Sci. USA. 1964;51:17–24. [PMC free article: PMC300597] [PubMed: 14104599]
- Ts’o P.O.P., Helmkamp G.K., Sander C. Interaction of nucleosides and related compounds with nucleic acids as indicated by the change of helix-coil transition temperature. Proc. natl Acad. Sci. USA. 1962;48:686–698. [PMC free article: PMC220834] [PubMed: 13922738]
- Tsuboi M., Yanagishima N. Comparative studies on sporulation-promotive actions of cyclic AMP, theophylline and caffeine in Saccharomyces cerevisiae. Arch. Mikrobiol. 1975;105:83–86. [PubMed: 173249]
- Tsuchimoto T., Matter B.E. In vivo cytogenetic screening methods for mutagens, with special reference to the micronucleus test. Arch. Toxicol. 1979;42:239–248. [PubMed: 160219]
- Turmen T., Louridas T.A., Aranda J.V. Relationship of plasma and CSF concentrations of caffeine in neonates with apnea. J. Pediatr. 1979;95:644–646. [PubMed: 480049]
- Tyrala E.E., Dodson W.E. Caffeine secretion into breast milk. Arch. Dis. Child. 1979;54:787–789. [PMC free article: PMC1545656] [PubMed: 507903]
- US Bureau of the Census (1989) US Exports and US Imports for Consumption (Customs Values) (Reports EM 522 and IM146), Washington DC, Foreign Trade Commission, pp. 5161–5162, 5179–5180.
- US Food and Drug Administration (1980) Caffeine Content of Various Products (FDA Talk Paper T80-45), Rockville, MD.
- US Food and Drug Administration (1984) The latest caffeine scorecard. FDA Consumer, March, 14–16.
- US Food and Drug Administration (1988) Caffeine. US Code fed. Regul, 182.1180, 392.
- US International Trade Commission (1987) Synthetic Organic Chemicals, US Production and Sales 1986 (USITC Publ. No. 2009), Washington DC, US Government Printing Office.
- US Pharmacopeial Convention (1990) US Pharmacopeia — The National Formulary, 22nd ed., Rockville, MD, pp. 204–205.
- Van der Meer C., Haas R.E. Determination of caffeine in serum by straight-phase high-performance liquid chromatography. J. Chromatogr. 1980;182:121–124. [PubMed: 7380897]
- Vergnes M.F., Alary J. Determination of natural xanthines by HPLC (Fr.). Talanta. 1986;33:997–1000. [PubMed: 18964243]
- Vig B.K. Somatic crossing over in Glycine max (L.) Merrill: effect of some inhibitors of DNA synthesis on the induction of somatic crossing over and point mutations. Genetics. 1973;73:583–596. [PMC free article: PMC1212915] [PubMed: 17248598]
- Vogel F., Krüger J., Kurth M., Schroeder T.M. On the lack of a relationship between coffee drinking by parents and the sex ratio of their children (Ger.). Humangenetik. 1966;2:119–132. [PubMed: 5915639]
- Voogd C.E., van der Vet P. Mutagenic action of ethylene halogenhydrins. Experientia. 1969;25:85–86. [PubMed: 5766598]
- Wahlländer A., Renner E., Karlaganis G. High-performance liquid chromatographic determination of dimethylxanthine metabolites of caffeine in human plasma. J. Chromatogr. (Biomed. Appl.). 1985;338:369–375. [PubMed: 3998024]
- Waksvik H., Brøgger A., Stene J. Psoralen/UVA treatment and chromosomes. I. Aberrations and sister chromatid exchange in human lymphocytes in vitro and synergism with caffeine. Hum. Genet. 1977;38:195–207. [PubMed: 908566]
- Waldren C.A. 1973The use of in vitro techniques in mammalian cell biology: some aspects of the action of caffeine and other agents on cells in culture Diss. Abstr. int. B 34553-B-554-B.
- Waldren C.A., Patterson D. Effect of caffeine on purine metabolism and ultraviolet light-induced lethality in cultured mammalian cells. Cancer Res. 1979;39:4975–4982. [PubMed: 498124]
- Walton H.F., Eiceman G.A., Otto J.L. Chromatography of xanthines on ion-exchange resins. J. Chromatogr. 1979;180:145–156. [PubMed: 44303]
- Warszawski D., Ben-Zvi Z., Gorodischer R. Caffeine metabolism in liver slices during postnatal development in the rat. Biochem. Pharmacol. 1981;30:3145–3150. [PubMed: 7317099]
- Warszawski D., Ben-Zvi Z., Gorodischer R., Arnaud M.J., Bracco I. Urinary metabolites of caffeine in young dogs. Drug Metab. Disposition. 1982;10:424–428. [PubMed: 6126345]
- Watkinson B., Fried P.A. Maternal caffeine use before, during and after pregnancy and effects upon offspring. Neurobehav Toxicol. Teratol. 1985;7:9–17. [PubMed: 4000371]
- Webb R.B. Continuous cultures in mutagen testing (Abstract). Genetics. 1970;64:S64–S65.
- Weinstein D., Mauer I., Solomon H.M. Effect of caffeine on chromosomes of human lymphocytes: in vivo and in vitro studies. Mutat. Res. 1972;16:391–399. [PubMed: 4638573]
- Weinstein D., Mauer I., Katz M.L., Kazmer S. The effect of caffeine on chromosomes of human lymphocytes: a search for the mechanism of action. Mutat. Res. 1973a;20:115–125. [PubMed: 4357386]
- Weinstein D., Mauer I., Katz M., Kazmer S. The effect of caffeine on chromosomes of human lymphocytes: non-random distribution of damage. Mutat. Res. 1973b;20:441–443. [PubMed: 4778858]
- Weinstein D., Mauer I., Katz M.L., Kazmer S. The effect of methylxanthines on chromosomes of human lymphocytes in culture. Mutat. Res. 1975;31:57–61. [PubMed: 1128545]
- Welch R.M., Hsu S.-Y., DeAngelis R.L. Effect of Aroclor 1254, phenobarbital, and polycyclic aromatic hydrocarbons on the plasma clearance of caffeine in the rat. Clin. Pharmacol. Ther. 1977;22:791–798. [PubMed: 410581]
- Welsch C.W., DeHoog J.V. Influence of caffeine consumption on 7,12-dimethylbenz(a)anthracene-induced mammary gland tumorigenesis in female rats fed a chemically defined diet containing standard and high levels of unsaturated fat. Cancer Res. 1988;48:2074–2077. [PubMed: 3127045]
- Welsch C.W., Scieszka K.M., Senn E.R., DeHoog J.V. Caffeine (1,3,7-trimethylxanthine), a temperate promotor of DMBA-induced rat mammary gland carcinogenesis. Int. J. Cancer. 1983;32:479–484. [PubMed: 6413433]
- Welsch C.W., DeHoog J.V., O’Connor D.H. Influence of caffeine consumption on carcinomatous and normal mammary gland development in mice. Cancer Res. 1988a;48:2078–2082. [PubMed: 3127046]
- Welsch C.W., DeHoog J.V., O’Connor D.H. Influence of caffeine and/or coffee consumption on the initiation and promotion phases of 7,12-dimethyl-benz(a)anthracene-induced rat mammary gland tumorigenesis. Cancer Res. 1988b;48:2068–2073. [PubMed: 3127044]
- West G.L., Sobotka T.J., Brodie R.E., Beier J.M., O’Connell M.W Jr. Postnatal neurobehavioral development in rats exposed in utero to caffeine. Neurobehav. Toxicol. Teratol. 1986;8:29–43. [PubMed: 3703093]
- Wietholtz H., Voegelin M., Arnaud M.J., Bircher J., Preisig R. Assessment of the cytochrome P-448 dependent liver enzyme system by a caffeine breath test. Eur. J. clin. Pharmacol. 1981;21:53–59. [PubMed: 6800827]
- Wilson, J.G. & Scott, W.J., Jr (1984) The teratogenic potential of caffeine in laboratory animals. In: Dews, P.B., ed., Caffeine. Perspectives from Recent Research, Berlin, Springer, pp. 165–187.
- Windholz, M., ed. (1983) The Merck Index, 10th ed., Rahway, NJ, pp. 225–226.
- Wolf K., Kaudewitz F. Effect of caffeine on the rho-induction with ethidium bromide in Saccharomyces cerevisiae. Mol. gen. Genet. 1976;146:89–93. [PubMed: 785213]
- Wong S.H.Y., Marzouk N., Aziz O., Sheeran S. Microbore liquid chromatography for therapeutic drug monitoring and toxicology: clinical analyses of theophylline, caffeine, procainamide and N-acetyl procainamide. J. liquid Chromatogr. 1987;10:491–506.
- Wragg J.B., Carr J.V., Ross V.C. 1967Inhibition of DNA polymerase activity by caffeine in a mammalian cell line (Abstract No 305) J. Cell Biol. 35146A–147A.
- Wurzner H.-P., Lindström E., Vuataz L., Luginbühl H. A 2-year feeding study of instant coffees in rats I. Body weight. Food consumption, haematological parameters and plasma chemistry. Food Cosmet. Toxicol. 1977a;15:7–16. [PubMed: 852786]
- Wurzner H.-P., Lindström E., Vuataz L., Luginbiihl H. A 2-year feeding study of instant coffees in rats II. Incidence and types of neoplasms. Food Cosmet. Toxicol. 1977b;15:289–296. [PubMed: 590890]
- Yamagami T., Handa H., Takeuchi J., Munemitsu H., Aoki M., Kato Y. Rat pituitary adenoma and hyperplasia induced by caffeine administration. Surg. Neurol. 1983;20:323–331. [PubMed: 6623345]
- Yamamoto K., Yamaguchi H. Inhibition by caffeine of the repair of γ-ray-induced chromosome breaks in barley. Mutat. Res. 1969;8:428–430. [PubMed: 5366018]
- Yanders A.F., Seaton R.K. The lack of mutagenicity of caffeine in Drosophila. Am. Nat. 1962;96:277–280.
- Yefremova G.I., Filippova L.M. Effect of caffeine on crossing-over in Drosophila melanogaster. Mutat. Res. 1974;23:347–352. [PubMed: 4210198]
- Yesair, D.W., Branfman, A.R. & Callahan, M.M. (1984) Human disposition and some biochemical aspects of methylxanthines. In: Spiller, G.A., ed., The Methylxanthine Beverages and Foods: Chemistry, Consumption and Health Effects, New York, Alan R. Liss, pp. 215–233.
- Zajdela F., Latarjet R. Inhibitory effect of caffeine on the induction of skin cancers by UV light in the mouse (Fr.). C.R. Acad. Sci. (Paris). 1973;277:1073–1076. [PubMed: 4206012]
- Zajdela, F. & Latarjet, R. (1975) The inhibitory effect of caffeine on the induction of cutaneous tumors in mice by ultraviolet rays. In: Bucalossi, P., Veronesi, U. & Cascinelli, N., eds, Proceedings of the XI International Cancer Congress, Florence, 20–26 October 1974, Vol. 3, Amsterdam, Excerpta Medica, pp. 120–123.
- Zajdela F., Latarjet R. Ultraviolet light induction of skin carcinoma in the mouse; influence of cAMP modifying agents. Bull. Cancer. 1978a;65:305–314. [PubMed: 214189]
- Zajdela F., Latarjet R. Inhibition of skin carcinogenesis in vivo by caffeine and other agents. Natl Cancer Inst. Monogr. 1978b;50:133–140. [PubMed: 753971]
- Zetterberg G. The mutagenic effect of 8-ethoxycaffeine, caffeine and dimethylsulfate in the Ophiostoma back-mutation test. Hereditas. 1960;46:279–311.
- Zettle T.E., Murnik M.R. Effects of caffeine on chromosomal loss and nondisjunction in Drosophila melanogaster. Genetica. 1973;44:146–153.
- Zoumas B.L., Kreiser W.R., Martin R.A. Theobromine and caffeine content of chocolate products. J. Food Sci. 1980;45:314–316.
Footnotes
- 1
For description of the italicized terms, see Preamble, pp. 27–31.
- Caffeine link to miscarriage.[Midwives. 2008]Caffeine link to miscarriage.. Midwives. 2008 Feb-Mar; 11(1):8.
- Caffeine ingestion during pregnancy: in utero exposure and possible effects.[Semin Perinatol. 1981]Caffeine ingestion during pregnancy: in utero exposure and possible effects.Soyka LF. Semin Perinatol. 1981 Oct; 5(4):305-9.
- Delayed elimination of caffeine by women in the last 2 weeks of pregnancy.[Can Med Assoc J. 1982]Delayed elimination of caffeine by women in the last 2 weeks of pregnancy.Parsons WD, Pelletier JG. Can Med Assoc J. 1982 Sep 1; 127(5):377-80.
- Review Psychotropic effects of caffeine.[Am Fam Physician. 1988]Review Psychotropic effects of caffeine.Clementz GL, Dailey JW. Am Fam Physician. 1988 May; 37(5):167-72.
- Review Caffeine (1, 3, 7-trimethylxanthine) in foods: a comprehensive review on consumption, functionality, safety, and regulatory matters.[J Food Sci. 2010]Review Caffeine (1, 3, 7-trimethylxanthine) in foods: a comprehensive review on consumption, functionality, safety, and regulatory matters.Heckman MA, Weil J, Gonzalez de Mejia E. J Food Sci. 2010 Apr; 75(3):R77-87.
- Caffeine - Coffee, Tea, Mate, Methylxanthines and MethylglyoxalCaffeine - Coffee, Tea, Mate, Methylxanthines and Methylglyoxal
Your browsing activity is empty.
Activity recording is turned off.
See more...