Included under terms of UK Non-commercial Government License.
NCBI Bookshelf. A service of the National Library of Medicine, National Institutes of Health.
Murphy GJ, Mumford AD, Rogers CA, et al. Diagnostic and therapeutic medical devices for safer blood management in cardiac surgery: systematic reviews, observational studies and randomised controlled trials. Southampton (UK): NIHR Journals Library; 2017 Sep. (Programme Grants for Applied Research, No. 5.17.)

Diagnostic and therapeutic medical devices for safer blood management in cardiac surgery: systematic reviews, observational studies and randomised controlled trials.
Show detailsAbstract
Background: Coagulopathic bleeding is a common and severe complication of cardiac surgery.
Objective: To assess the benefits of introducing routine POC tests or an expanded range of laboratory diagnostic tests for coagulopathy compared with the current standard of care (clinical risk assessment) for the management of cardiac surgery patients with severe bleeding.
Methods: A diagnostic accuracy study and linked cost-effectiveness analysis compared POC platforms in current use for the prediction of post-surgery haemorrhage [rotational thromboelastometry (ROTEM) (ROTEM® Delta; TEM International GmbH, Munich, Germany), thromboelastography (TEG) (TEG® 5000 Thromboelastograph® Haemostasis Analyzer; Haemonetics Corporation, Niles, IL, USA) and Multiplate® analyzer (Roche, Rotkreuz, Switzerland)], as well as alternative LRTs of coagulopathy, with standard care. The clinical effectiveness of POC testing in cardiac surgery was evaluated in a systematic review of RCTs.
Results: In the predictive accuracy study 2541 participants were recruited between March 2010 and September 2012. Perioperative POC and LRT results did not result in clinically important improvements in predictive accuracy beyond that achieved using clinical risk prediction alone. The cost-effectiveness analysis demonstrated very little difference between any of the POC tests and current practice in either costs or life-years saved. The systematic review demonstrated that the routine use of POC tests did not improve clinical outcomes.
Conclusions: Existing diagnostic tests for coagulopathy have limited additional value for the diagnosis and treatment of coagulopathic bleeding in cardiac surgery beyond the current standard of care.
Study registration: Current Controlled Trials ISRCTN20778544 and PROSPERO CRD42016033831.
Background
The clinical problem
Coagulopathic bleeding is a common and severe complication of cardiac surgery. In the UK up to 5% of all patients require emergency re-exploration for bleeding in the immediate postoperative period.9 This is associated with a fourfold increase in mortality and resource use (Figure 1). Adverse events associated with severe bleeding may be attributable to the severity of the underlying illness and the complexity of surgery, which contribute to coagulopathy, as well as the consequences of significant haemorrhage and shock. The adverse effects of coagulopathic bleeding may also be attributable in part to the side effects of treatment. Large-volume red cell transfusions or the administration of pro-haemostatic therapies such as platelet and FFP transfusion and recombinant activated factor VII (rFVIIa) have well-documented risks.7 These risks, although offset by the risks of ongoing bleeding in coagulopathic patients, may be clinically significant in those without coagulopathy or when administered to those who are not actively bleeding.

FIGURE 1
Forest plots summarising effect estimates from observational studies that have considered the effects of emergency reternotomy for bleeding on mortality. M–H, Mantel–Haenszel.
A range of medical devices are in common use as POC diagnostic tests for the prediction of severe bleeding in patients and its treatment; however, the evidence to support their use is inconsistent and this is reflected by wide variation in their use.9 This chapter will establish whether commonly used POC testing devices have good predictive accuracy for coagulopathic bleeding when used routinely and consider whether the introduction of POC testing algorithms for the diagnosis and management of bleeding is cost-effective. It will also consider whether the introduction of additional diagnostic tests improves predictive accuracy and effectiveness.
Coagulopathic bleeding
Coagulopathic haemorrhage is poorly defined. The current consensus definition of coagulopathy is a prolonged prothrombin time (PT) of > 1.2 times normal.51 However, prolonged PT and abnormalities in other standard laboratory tests (SLTs) have not been found to have predictive accuracy for coagulopathic haemorrhage in cardiac or non-cardiac surgery.52,53 Many patients with abnormal standard laboratory coagulation tests do not develop bleeding and coagulopathic bleeding may occur when these tests are normal. This reflects the limitations of the tests and the complex aetiology of coagulopathy. This means, however, that it is often difficult to distinguish coagulopathic bleeding from non-coagulopathic bleeding. Defining excessive bleeding is also problematic in situations in which bleeding occurs to some degree in all patients. The efficacy of haemostatic interventions is also unclear. The lack of a clear definition of coagulopathic bleeding complicates epidemiological analyses and the development of accurate diagnostic tests and treatments. Excessive bleeding in cardiac surgery may be defined clinically as:
- Emergency re-sternotomy for life-threatening haemorrhage or cardiac tamponade within hours of surgery. This definition includes patients with very severe coagulopathic haemorrhage in which conventional pro-haemostatic therapies have failed to control bleeding. This has limitations as a definition because emergency re-sternotomy may be required for ‘surgical’ bleeding in the absence of coagulopathy. There are also important institutional differences in how patients with haemorrhage are managed and how readily patients are re-explored when tamponade is suspected. This is reflected by emergency re-sternotomy rates that range from 0.3% to 12% between UK units.9 This definition may therefore underestimate the proportion of patients with coagulopathic haemorrhage.
- Large-volume red cell transfusion. Massive blood transfusion (MBT) defined as ≥ 10 units of red cells within 24 hours, or 4 units within 1 hour, is rare in cardiac surgery. Large-volume red cell transfusion (≥ 4 units of red cells) is common, however. In the UK 22% of patients receive a LVBT perioperatively.9 This equates to approximately 1000 ml of packed red cells or 20% of the circulating blood volume of a 70-kg adult. Transfusion of ≥ 4 units has been shown to be associated with significant increases in perioperative morbidity and mortality in cardiac surgery.10 A limitation of this definition is that red cells are also commonly administered for the treatment of anaemia and this may therefore overestimate the frequency of coagulopathic haemorrhage. Late transfusions, for example after 24 hours postoperatively, are also unlikely to represent treatment for bleeding.
- Transfusion of allogenic non-red cell components to promote haemostasis. In the UK up to 25% of cardiac surgery patients receive FFP or platelets to arrest or prevent coagulopathic bleeding.9 The limitation of this definition is that currently non-red cell transfusions are often administered empirically based on subjective assessments by clinical staff. As a consequence there is variation in the frequency of non-red cell component transfusion across UK units.9 This reflects differences in case mix and institutional practices; however, it also suggests that many patients receive unnecessary transfusions. This definition would therefore overestimate the frequency of coagulopathic bleeding.
- Excessive blood loss. The volume of blood loss in the drains is routinely measured. Excessive bleeding has been defined as > 10 ml/kg/hour.54 This has limitations as an indicator of coagulopathic bleeding because (1) patients with coagulopathic bleeding who receive effective pro-haemostatic treatment may not have excessive blood loss, (2) excessive blood loss may be masked by blocked chest drains and this may present as tamponade or late pleural and pericardial collections, (3) excessive blood loss may have a surgical as opposed to a coagulopathic cause and (4) drain losses may not represent active bleeding and may consist of pleural or pericardial collections and effusions.
In summary, existing consensus definitions of coagulopathy or coagulopathic haemorrhage in cardiac surgery have important limitations. Clinical studies must anticipate these limitations by, for example, excluding patients with clear ‘surgical’ causes of bleeding; by careful assessment of adherence to blood management protocols for transfusion and the use of therapeutic adjuncts such as cell salvage or antifibrinolytic use; and by using definitions of drain losses that are most likely to reflect active bleeding. Assessment of predictive accuracy should also consider alternative definitions of coagulopathic haemorrhage within analyses to obtain a balanced assessment of utility. These are features of the COPTIC study.
Clinical risk factors for coagulopathic bleeding
Careful clinical assessment can be used to identify patients at risk of coagulopathic haemorrhage and this is recommended as part of standard care.24 Diagnostic tests for coagulopathy should be interpreted, and pro-haemostatic treatments should be administered, only in the context of known clinical characteristics.
Preoperative factors
Heritable coagulopathy
Mild heritable coagulation factor defects are prevalent in the general population and are associated with increased bleeding during surgery.55 These may be detected by routine screening SLTs or by clinical assessment and a history of bleeding after surgical procedures. These require further detailed investigation by a haematologist.
Antiplatelet agents
Most patients with symptomatic cardiovascular disease receive aspirin. In addition, patients with acute coronary syndromes or those who have received coronary stents receive dual antiplatelet therapy (DAPT) with the addition of the P2Y12 receptor antagonists clopidogrel, prasugrel (Efient®; Eli Lilly and Co., Indianapolis, IN, USA) or ticagrelor (Brilique®; AstraZeneca, Cambridge, UK), because of the proven benefit of DAPT in these settings.56–58 Platelet inhibition increases blood loss during surgery,59 but, although desirable, early withdrawal of antiplatelet therapy increases the risk of preoperative acute cardiovascular events and death in those at greatest risk. Current guidelines recommend that aspirin be continued until the time of surgery as RCTs have indicated that, although this increases blood loss marginally, there are no serious adverse events attributable to this blood loss and there are clear benefits in terms of fewer adverse cardiovascular events prior to surgery.60 Observational analyses demonstrate more severe bleeding and adverse events attributable to platelet inhibition with DAPT relative to aspirin alone61,62 and current guidelines (class I recommendation, evidence level B) recommend that P2Y12 receptor antagonists be stopped 3–5 days prior to surgery.24 This empirical approach is not without risk; many patients may be left at increased risk of adverse cardiovascular events prior to surgery and this is not detected by observational studies that commonly analyse events from surgery, a form of lead time bias. Conversely, other patients may have significant residual platelet inhibition at operation. This variability in responsiveness is more commonly observed with clopidogrel than with the newer agents such as ticagrelor and prasugrel, for which pharmacokinetics are more predictable.
Preoperative morbidity
Clinical risk prediction scores have been developed that allow objective assessment of bleeding risk. These identify age, female sex, low body mass index (BMI), preoperative anaemia, chronic kidney disease, poor left ventricular function and recent myocardial ischaemia as predictive of excessive bleeding or MBT.63,64 The mechanism by which these factors contribute to bleeding is poorly understood. Existing scores are not widely used because of the limited generalisability of risk models developed in single centres or subpopulations of patients. This is considered in detail in Chapter 3.
Intra- and postoperative factors
Protease activation/clotting factor consumption/fibrinolysis
Direct activation of the contact system of proteases (kallikrein, kininogen) by the negatively charged surface of the CPB circuit results in the generation of factor XIIa and activation of the intrinsic clotting cascade (Figure 2). In addition, release of tissue factor by surgical trauma and the activation of inflammatory cells will activate the extrinsic coagulation pathway. Both result in activation of the common coagulation pathway and the uncontrolled generation of thrombin during CPB. Thrombin and activated contact proteases also promote fibrinolysis through the activation of tissue plasminogen activator. High levels of thrombin formation and fibrinolysis ultimately result in factor depletion and deranged haemostasis, which is most evident after prolonged CPB duration.66 This is further impaired by haemodilution with circuit prime, platelet dysfunction and depletion.
Platelet dysfunction
The circulation of blood through the CPB circuit directly activates circulating platelets, as do thrombin and contact proteases, which then adhere to the circuit or activated endothelium and leucocytes. This may lead to depletion of platelets, whose number may be further reduced by haemodilution. Non-adherent activated platelets become desensitised to further stimuli and the degree of platelet dysfunction correlates well with CPB duration.67 Post-cardiac surgery platelet dysfunction is common and is enhanced by the administration of antiplatelet agents such as aspirin or P2Y12 receptor antagonists preoperatively. Administration of more potent antiplatelet agents such as P2Y12 receptor antagonists up to the time of surgery may be associated with severe bleeding.61
Acidosis, hypothermia and haemodilution
These factors serve to reduce coagulation factor and platelet activity through direct suppression of enzyme activity. They also promote inflammation, endothelial dysfunction and organ injury.68
Inflammation and organ dysfunction
Protease and platelet activation during CPB lead to oxidative stress, leucocyte and endothelial activation, leucocyte extravasation, endothelial dysfunction, refractory tissue hypoxia and ultimately organ injury.2 The contribution of these factors to coagulopathy is poorly understood; however, there are strong associations between coagulopathy and post-cardiac surgery organ failure in observational studies.69
Heparin
Heparin is used in almost every patient undergoing cardiac surgery to provide systemic anticoagulation and prevent fibrin formation and thrombosis within the patient or activated CPB circuit. Heparin is reversed by the administration of protamine once the patient has been weaned from bypass successfully. Heparin is a small highly sulfated glycosaminoglycan and can accumulate in the extravascular compartment during CPB, where protamine may be less effective at reversal. Subsequent re-entry of heparin to the intravascular space may lead to residual heparin activity or ‘heparin rebound’ and contribute to bleeding.70
Blood management adjuncts
Evidence from RCTs indicates that the administration of antifibrinolytics such as tranexamic acid reduces bleeding and improves survival in cardiac surgery.17 Concomitant use of blood management adjuncts is an important consideration when determining the effectiveness of blood management interventions being evaluated in clinical trials.
Diagnosis of coagulopathy
Standard laboratory tests
Prothrombin time is a measure of the extrinsic pathway of coagulation. The PT is the time that plasma takes to clot after the addition of tissue factor. PT is often expressed as the international normalised ratio, which is the ratio of a patient’s PT to a normal (control sample) referenced against the international sensitivity index value for the analytical system used and corrects for differences in assay responses to different sources of tissue factor.
The activated partial thromboplastin time (aPTT) measures the ‘intrinsic’ or contact activation pathway and the common coagulation pathway. An activated matrix (e.g. silica, celite, kaolin) and calcium are mixed into the plasma sample and the time that the sample takes to clot is measured.
Fibrinogen (factor I) is a soluble plasma protein that is cleaved by thrombin to generate insoluble fibrin polymers, a principal component of the primary haemostatic response. Fibrinogen levels are commonly measured using the Clauss assay, with the time taken for plasma to clot in the presence of high thrombin concentrations compared with that of reference plasma with a known level of fibrinogen calibrated against a known international standard. Low preoperative fibrinogen levels or depletion of fibrinogen during prolonged CPB have been associated with excessive blood loss and adverse clinical events in observational studies.71,72
Cross-linked fibrin is cleaved in the presence of plasma into fibrin degradation products, the largest of which is the D-dimer. Levels of the D-dimer are therefore considered as markers of thrombus (fibrin) formation and lysis.
Standard laboratory tests are considered to have limited utility in the setting of acute haemorrhage and coagulopathy. These limitations include the following:
- There is a slow turnaround time for results of 40 minutes on average, which precludes their use in unstable bleeding patients.
- These tests (aPTT, PT, fibrinogen) evaluate time to clot formation in platelet-poor plasma at 37 °C in standardised conditions and were developed to screen for clotting factor deficiencies or to monitor anticoagulant therapy. They were not designed to diagnose coagulopathy in the acutely bleeding surgical patient.73 Moreover, the effects of important factors such as the patient’s temperature, acidosis or platelet function are not reflected in these tests.
- The tests lack specificity with regard to the specific defect in the coagulation pathway responsible for prolonged PT or aPTT values and do not reflect platelet dysfunction.
- Platelet counts in whole blood do not account for alterations in platelet function or clinical status (antiplatelet therapy), which limits their utility in cardiac surgery.
As a consequence of these limitations, SLTs have poor predictive and prognostic utility in cardiac surgery.52,53 Despite this, they are routinely performed pre- and postoperatively in all patients.
Laboratory reference tests
Reference assays have important advantages compared with SLTs. They can measure specific components of the coagulation pathway, as well as provide quantitative measures of pathway activity. They have important disadvantages, however, in that they are expensive and time-consuming to perform and this limits clinical utility. However, they may be used to accurately define specific phenotypes of coagulopathy that other tests may be compared against and have a valuable role as reference tests in studies of coagulopathy.
Factor XIII or fibrin-stabilising factor is a circulating transglutaminase that is cleaved by thrombin to form activated factor XIII. This acts on fibrin to form cross-links between fibrin molecules to form an insoluble clot. Factor XIII becomes depleted during CPB and has been implicated in post-cardiac surgery coagulopathy.74 Factor XIII activity can be assessed using the Berichrom® XIII assay (Sysmex, Kobe, Japan), which measures ammonia release (transglutaminase activity) by activated factor XIII in soluble fibrin.
Incomplete reversal of heparin leads to anticoagulation and bleeding. There is no accurate SLT for incomplete reversal or ‘heparin rebound’ and it is common for this to be evaluated using the activated clotting/coagulation time (ACT), a measure of global clotting activity in whole blood. However, the ACT may be affected by non-heparin factors and can be normal in the presence of residual heparin activity or coagulopathy.75 Heparin acts by binding to antithrombin (see Figure 2). This inhibits both thrombin (factor II) and factor Xa. Anti-Xa activity is less sensitive to the effects of non-heparin factors than ACT or aPTT and is considered a more accurate measure of residual heparin activity.76 Anti-Xa activity may be measured accurately in automated laboratory analysers using a commercial assay (anti-Xa kit; HYPHEN BioMed, Neuville-sur-Oise, France).
The endogenous thrombin potential (ETP) is a test of global coagulation pathway function, similar to the PT and aPTT, performed using a thrombinoscope. Unlike SLTs, the output of this assay is presented as a thrombin generation curve, rather than simply the time to clot formation. This curve reflects all three phases of coagulation: initiation, which corresponds to the PT or aPTT depending on the activator, followed by propagation and termination, which are quantitative measures of thrombin activity. It has been hypothesised that this is a measure of the thrombin burst, a functional, rather than a static, measure of common coagulation pathway activity. The propagation and termination phases of the curve can be quantified as the area under the curve (AUC), which is termed the ETP. In one study the ETP was shown to discriminate between cardiac surgery patients who developed severe blood loss and those who did not.77
Von Willebrand factor (vWF) is a multimeric adhesive glycoprotein that mediates the adhesion of platelets to injured subendothelium (collagen) and to the platelet surface receptor GPIb. It is required for the formation of the initial platelet plug that achieves primary haemostasis following vascular injury. It also serves as the specific carrier protein for coagulation factor VIII in plasma, preventing proteolytic degradation. vWF, as a large multimeric protein, is subject to shear and fragmentation during CPB. Depletion of multimeric vWF has been implicated in post-CPB bleeding.78 vWF activity can be measured using the collagen binding assay, which detects the ability of multimeric forms of vWF to bind collagen.
In addition to data from specific LRTs, routinely recorded data obtained from automated cell counters used as part of standard clinical care may be able to provide information that will discriminate between patients who are likely to bleed and those who are not. Automated cell counters that measure the standard platelet count can detect thrombocytopenia, a risk factor for bleeding. In addition, they also measure the immature platelet count. This is a measure of thrombopoiesis that reflects the accelerated reduction of immature platelets in the setting of accelerated platelet consumption, as seen, for example, following prolonged CPB. High levels of immature platelets would implicate low platelet function as a cause of associated coagulopathy. Conversely, an elevated mean platelet volume (MPV) is associated with increased platelet activation and thrombosis. A low platelet volume may reflect reduced platelet activity. As no additional tests are performed beyond existing care, these data are available at no additional cost as they are obtained routinely as part of usual care. The predictive accuracy of these measures for bleeding has not previously been reported.
Point-of-care tests
Near-patient tests allow clinical teams to undertake rapid, reproducible and repeated assays of coagulation with short turnaround times at the POC. These offer more personalised and effective management in bleeding patients, whose clinical status may be unstable. The two most common POC testing platforms in clinical use are the viscoelastic tests ROTEM and TEG.
These devices have limited ability to discriminate between platelet dysfunction and defects in fibrin generation and are often complemented by platforms that can detect specific defects in platelet function.79 A commonly used platelet function testing platform in the UK is the Multiplate analyzer.
Rotational thromboelastometry
Previously known as rotational TEG, the ROTEM assays are based on the oscillation of a disposable sensor pin suspended within a citrated whole blood sample (340 µl) placed within a disposable cuvette (sample cup). Coagulation is activated by a range of assays and as the clot forms the resistance to the rotation of the pin is increased to provide a measure of clot firmness. This is detected by an optical sensor and translated into the ROTEM output (Figure 3). By using different activators discrete components of clot formation can be assessed within a single integrated platform. The individual assays are as follows:
- INTEM. Uses an activator of the contact (kallikrein) proteases and measures the intrinsic clotting pathway.
- EXTEM. Uses tissue factor as an activator and is a measure of the extrinsic pathway. This assay is not affected by heparin.
- HEPTEM. Uses an activator of the contact proteases along with heparinise (effectively the INTEM assay in the presence of heparinase) and indicates the presence of residual heparin activity.
- FIBTEM. Uses tissue factor as an activator along with an inhibitor of platelet activation (effectively the EXTEM pathway in the absence of platelet function). The output of this assay denotes fibrin formation and is considered a measure of fibrinogen concentration.
Each test generates multiple values that reflect dynamic clot formation and lysis. A separate graphical output of results is produced for each assay. A representative output is displayed in Figure 3. Output definitions are listed in Box 1.
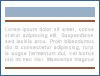
BOX 1
Rotational thromboelastometry outputs and interpretation
Thromboelastography
The TEG system is also based on a viscoelastic test, with some differences between this system and ROTEM. In this system 340 µl of citrated whole blood is placed in a cuvette. A disposable pin is then lowered into the fluid blood and the cuvette is rotated. As the first fibrin strands are formed the pin becomes tethered to the cup and starts to follow its motion. When maximum clot firmness (MCF) is achieved, the cup and pin move in unison. The motion of the pin is detected by a torsion wire linked to a transducer that is interpreted as a graphical trace by computer software. As with ROTEM, different activators may be used to evaluate different components of clot formation:
- kaolin is used as an activator of the intrinsic pathway – analogous to the INTEM assay
- heparinase is used along with kaolin – analogous to the HEPTEM assay.
Other activators and assays have been described, including a functional fibrinogen assay and platelet mapping; however, these are not in common use and are associated with additional and significant costs relative to the two principal assays. As with ROTEM, the graphical output provides visual information related to dynamic clot formation and also provides information that reflect specific stages of clot formation (Figure 4). Definitions of TEG outputs are listed in Box 2.
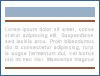
BOX 2
Thromboelastography outputs and interpretation
Multiplate
Platelets are activated by a wide array of stimuli that include thrombin, tissue factor, adenosine diphosphate (ADP) and arachidonic acid metabolites such as thromboxane. Activation is accompanied by aggregation and adhesion to other platelets via fibrin strands and to exposed extravascular matrix through interaction with vWF. Aggregation can be measured in small volumes of whole blood (1.2 ml) by multiple electrode aggregometry (MEA) using the Multiplate device. The instrument performs the analysis by employing two independent sensor units for every test cell, representing an internal control of the reaction. Each unit consists of two silver-coated, highly conductive copper wires on which the platelets adhere on specific activation. The aggregation function is measured in terms of increasing electrical impedance between the metallic electrodes of the sensor as platelets aggregate on the electrodes in the presence of an activator. The results are displayed graphically (Figure 5) within minutes. Up to four activators may be assessed in a single assay. The cyclo-oxygenase pathway is activated with 0.5 mM of arachidonic acid (ASPI) (ASPItest®), the P2Y12 receptor pathway with 6.5 µM of ADP (ADPtest®) and the thrombin receptor with a thrombin receptor-activating peptide (TRAP) (TRAPtest®). By comparing these results with those for maximal platelet activation using high adrenaline (epinephrine) concentrations (20 mg/ml) (ADRENtest®), the degree of inhibition of specific activation pathways can be assessed. Outcomes are expressed as the AUC [in aggregation units (AUs)*min] or the maximum amplitude (in AUs). The test results can be assessed more rapidly using the test velocity, expressed as dAU/dt, within minutes of activation (see Figure 5). A limitation of the Multiplate is that platelet counts must ideally be > 100 × 109/l to obtain reproducible results.
Treatment
Without accurate diagnostic tests to identify specific defects in the coagulation pathway, which if treated or reversed can improve clinical outcomes, the management of coagulopathy is often empirical, non-specific and based on the assumption that reversal of coagulopathy is beneficial.
Fresh-frozen plasma
One unit of FFP is derived from a single unit of donated red cells and contains variable concentrations of the coagulation factors I (fibrinogen), II, V, VII, VIII, IX, X and XI. It also contains anticoagulants such as protein C, protein S and antithrombin along with a large number of proteins such as immunoglobulins, albumin and acute-phase proteins. A standard dose of FFP is 2 units and there is uncertainty whether this significantly increases coagulation factor concentrations in recipients. Uncertainty over the risks and benefits of FFP80,81 is reflected by the variability in its use, with use ranging from 9% to 59% of patients between centres (mean 25%).9 Cardiac surgery uses up to 12% of all FFP produced by NHS Blood and Transplant.82
Platelets
In the UK a standard unit of platelets contains the pooled platelet component of up to six donors. There is very little evidence to guide platelet transfusion in the setting of coagulopathic haemorrhage. A recent RCT reported that empirical transfusion of higher platelet ratios, in combination with higher plasma ratios, improves haemostasis and leads to fewer deaths from bleeding at 24 hours in trauma patients.83 Evidence on the risks and benefits of platelet transfusion in cardiac surgery comes from observational analyses and shows conflicting results.84,85 The rate of platelet transfusion in cardiac surgery is variable, ranging from 10% to 56% (mean 28%) of patients between UK cardiac surgery units. Cardiac surgery is one of the largest single consumers of platelets (17%) produced by NHS Blood and Transplant.82
Fibrinogen
UK guidelines recommend that fibrinogen is replaced during major blood loss or as part of the management of disseminated intravascular coagulation once the Clauss fibrinogen value falls below 1.5 g/l, although this recommendation is based on weak evidence.86 Cryoprecipitate is the first-line treatment in the UK for acquired hypofibrinogenaemia and a standard adult dose (two pools) raises the plasma fibrinogen level by 1 g/l. A Cochrane review of predominantly cardiac surgical RCTs found that fibrinogen reduced transfusion requirements (very low-quality evidence), but there was insufficient evidence to evaluate differences in important clinical end points, including mortality.87
Recombinant activated factor VII
Recombinant activated factor VII is a potent pharmacological pro-haemostatic agent licensed for use in patients with haemophilia. This has led to the off-label use of rFVIIa for the treatment of severe coagulopathic bleeding in trauma and surgical settings as an adjunct to conventional non-red cell blood components.
Prothrombin complex concentrates
Prothrombin complex concentrates are plasma-derived coagulation factor concentrates that contain three or four vitamin K-dependent factors at high concentration, typically factors II, VII, IX and X, as well as variable amounts of anticoagulants and heparin. These are increasingly used off-licence in cardiac surgery in the setting of coagulopathic haemorrhage; however, this is not supported by evidence.
Current compared with proposed new standards of care for the diagnosis and treatment of coagulopathy
As both preoperative and operative variables may contribute to coagulopathy and bleeding in cardiac surgery, we considered how improved testing of coagulopathy both before and after surgery would benefit patients when used in conjunction with careful clinical assessment.
Preoperative testing
Current standard of care
Patients undergoing cardiac surgery undergo preoperative screening with SLTs including tests of PT and aPTT. In some centres preoperative fibrinogen is also measured routinely. Platelet counts are measured on standard haematology analysers. Abnormal screening tests or a clinical history of excessive blood loss following previous dental extractions or minor surgical procedures may lead to further haematological investigations on an individual basis. In addition to standard care, current blood management guidelines recommend (class 1, level of evidence C24) that patients undergo a formal assessment and documentation of bleeding risk. Risk scores that may accurately predict the risk of bleeding and red cell transfusion exist;63,64,88,89 however, these are not widely used. The predictive accuracy of any new diagnostic test should therefore complement appropriate clinical assessment and the benefits of additional testing should be assessed beyond those attributable to clinical assessment combined with standard testing.
Proposed new standard of care
Our proposed new standard of care includes routine preoperative platelet function testing in addition to current standard care. We suggest that this may have clinical benefits by allowing targeted interventions to be given to patients with pre-existing platelet dysfunction that will reduce the risk of severe bleeding and its complications, particularly in patients receiving antiplatelet therapy preoperatively. Currently, it is recommended that aspirin be continued until surgery but that P2Y12 receptor antagonists be discontinued 3–5 days prior to surgery.24 This reflects the reversal characteristics of current P2Y12 receptor antagonists and clinical outcomes in observational studies in which patients on recent DAPT have undergone cardiac surgery. There is marked variation in the implementation of these guidelines. Specifically, increases in the numbers of non-elective surgeries for patients with unstable cardiovascular disease or recent coronary stenting in the setting of myocardial infarction (MI) mean that patients frequently present to surgery within 3–5 days of taking DAPT or require DAPT because of the risk of acute stent thrombosis.90 In these patients preoperative platelet function testing may allow more informed decision-making as to the risks of surgery and bleeding and the need for pre-emptive platelet transfusion or deferment of surgery.
What evidence supports this change in the current standard of care for preoperative testing?
Corredor et al.25 recently reviewed clinical studies that have evaluated the predictive accuracy of POC platelet function tests to detect platelet dysfunction and predict postoperative bleeding in cardiac surgery (Table 3). They identified 16 studies in which platelet function tests had been used in isolation to predict postoperative haemorrhage. The studies included in the systematic review were at high risk of bias and only five of 15 studies reported test discrimination for bleeding or transfusion. Overall, the Multiplate was evaluated in the largest number of patients and showed modest discrimination. Test results were more likely to accurately predict bleeding in patients receiving P2Y12 receptor antagonists prior to surgery. The authors concluded that there was inconsistent evidence that routine preoperative platelet function testing had predictive accuracy for bleeding, particularly in patients receiving DAPT. This uncertainty is reflected in current treatment guidelines. The Society of Thoracic Surgeons clinical practice guidelines (updated in 2012) state that ‘For patients on dual antiplatelet therapy, it is reasonable to make decisions about surgical delay based on tests of platelet inhibition rather than arbitrary use of a specified period of surgical delay (Class Iia, Level of Evidence B)’.91 Conversely, a recent position statement has recommended routine Multiplate use in patients receiving P2Y12 receptor antagonists.92 To address this uncertainty we assessed the predictive accuracy of routine preoperative platelet function tests in the COPTIC A study.
TABLE 3
Summary of observational studies of POC and platelet function tests
The COPTIC A study
The objective of this study was to examine the relationship between preoperative abnormalities in blood coagulation and coagulopathic bleeding after cardiac surgery. Although numerous abnormalities in both clotting factors and platelets have been reported in patients before cardiac surgery, the most prevalent and clinically important abnormalities are likely to be in platelets. This is because antiplatelet drugs such as aspirin and the P2Y12 blockers clopidogrel, ticagrelor or prasugrel are prescribed widely to patients before cardiac surgery and markedly reduce platelet function.
Approximately 50% of cardiac surgery patients receive aspirin alone up to the day of surgery and approximately 25% receive DAPT. For the dual-therapy subgroup, current practice guidelines suggest withdrawal of the P2Y12 blocker approximately 3–5 days before cardiac surgery but continuation of aspirin up to the day of surgery.24,60 In practice, the duration of withdrawal varies between 0 and 7 days before surgery because of clinical operational constraints. We hypothesised that exposure to aspirin alone or exposure to aspirin plus a P2Y12 blocker would be associated with more clinical concern about bleeding (CCB) than no exposure to antiplatelet drugs. We also hypothesised that, in the DAPT subgroup, a short time of withdrawal of the P2Y12 blocker would be associated with more coagulopathic bleeding than a long time of withdrawal.
It is already known that antiplatelet drugs cause abnormal results in some laboratory tests of platelet function. Some other preoperative factors may also influence platelet function tests. It is unknown whether any laboratory test or combination of tests of platelet function can be used to predict CCB. The overall aim of the COPTIC A study was to estimate the association between the results from tests of platelet function and platelet count performed before cardiac surgery and CCB in all patients. As antiplatelet drugs are known to influence Multiplate results, we also estimated the association between test results and coagulopathic bleeding in patient subgroups (no antiplatelet drugs vs. aspirin alone vs. aspirin plus P2Y12 blockers). We also estimated the association between test results and duration of withdrawal of P2Y12 blockers and the relationship between duration of withdrawal of P2Y12 blockers and coagulopathic bleeding. Other preoperative, intraoperative and postoperative factors were considered as potential confounders of the association between platelet test results and coagulopathic bleeding. Finally, estimates of association were used to generate a parsimonious predictive model of the probability of coagulopathic bleeding based on preoperative platelet test results and baseline clinical factors.
Postoperative laboratory testing
Current standard of care
A recent audit of UK cardiac centres9 demonstrated wide variation in the diagnosis and management of postoperative bleeding across UK centres. The majority of centres use routine SLTs in combination with ad hoc POC tests in patients who are bleeding or in those considered as being at high risk. In the 2011 UK audit of blood transfusion in adult cardiac surgery,9 of the 17 UK centres that replied to a questionnaire on the use of blood management adjuncts, two reported that they did not use POC testing, four reported that POC testing was used in 1–25% of patients and six reported the use of POC tests in 75–100% of patients.
Proposed new standard of care
The routine use of pre- and postoperative POC tests in all patients, including TEG, ROTEM and Multiplate, would have important clinical benefits beyond those attributable to the current standard of care by enabling early and accurate diagnosis of coagulopathy and the early targeted treatment of patients who are likely to develop coagulopathic haemorrhage.
What evidence supports a change in the current standard of care for postoperative testing?
Predictive accuracy of point-of-care tests
Corredor et al.25 summarised the results of existing clinical studies that have considered the predictive accuracy of TEG/ROTEM and platelet function tests in cardiac surgery in a systematic review (see Table 3). All of these studies were of poor methodological quality. Most were small single-centre studies that recruited non-consecutive cohorts and included only small numbers of patients with coagulopathic bleeding, limiting study power and precision. In addition, clinicians in most studies were aware of the test results and this is likely to have influenced the study outcomes. The definition of bleeding was not standardised between studies and in some cases outcome definitions identified only those with the most severe coagulopathic bleeding, inflating estimates of diagnostic accuracy and reducing the likelihood that the findings would translate to unselected populations. The main limitation was that most studies reported associations between test results and bleeding and not measures of diagnostic accuracy, limiting interpretation of the likely clinical utility of the tests. The authors of the review concluded that platelet function tests in combination with TEG/ROTEM had greater discriminatory power than the assessment of platelet function by TEG/ROTEM alone. They also concluded that there was uncertainty as to the most appropriate tests for the management of postoperative bleeding in cardiac surgery patients.
Clinical effectiveness of point-of-care tests
The results of RCTs in cardiac surgery patients that have evaluated the clinical effectiveness of POC test-based treatment algorithms for coagulopathic bleeding have been summarised in a systematic review undertaken by Whiting et al.26 This formed the basis for recent NICE guidelines that recommended the use of TEG/ROTEM devices for the management of coagulopathic haemorrhage in cardiac surgery.23 The authors identified 11 RCTs that had evaluated the use of POC testing algorithms in 1089 patients. The included trials evaluated either ROTEM or TEG, with (n = 2) or without (n = 9) additional platelet function testing. Two trials evaluated ad hoc testing in bleeding patients and nine evaluated routine testing. Eight trials undertook serial pre- and postoperative tests. Quantitative meta-analysis that included all trials concluded that the intervention, POC testing algorithms, reduced the rate of red cell transfusion (RR 0.88, 95% CI 0.80 to 0.96). There was no difference between intervention and control groups for important clinical outcomes including death (RR 0.87, 95% CI 0.35 to 2.18) and reoperation for bleeding (RR 0.72, 95% CI 0.41 to 1.26) or for intensive care or hospital length of stay (LOS).
Cost-effectiveness of point-of-care tests
Whiting et al.26 also undertook a cost–utility analysis of the use of POC tests in cardiac surgery. No formal cost-effectiveness analysis of the use of POC tests has been performed to date and therefore clinical events, costs and utilities [quality-adjusted life-years (QALYs)] were estimated from published data. This analysis concluded that TEG/ROTEM dominated SLTs, being cost saving and more effective. This analysis was limited, however, in that it was based largely on a previous study conducted for the Scottish NHS in 2006 and published in 2008.93 The authors used several assumptions from the 2006 analysis that recent clinical studies have shown to be incorrect. The principal limitation of this analysis is that the economic model was based on the difference in transfusion rates between groups allocated to POC testing algorithms and groups allocated to standard care identified in the systematic review.26 The economic model in this analysis presupposed that red cell transfusion causes harm, an assumption based on a previous observational analysis showing significant associations between red cell transfusion and adverse clinical outcomes.94 However, the association between transfusion and harm is most likely the result of confounding.32 Other limitations include the omission from the economic model of the assessment of clinical outcomes measured in the trials and that the model used estimates of utilities (QALYs) from 199695 and not measured values. All of these limitations will have biased the analyses towards the use of POC testing.
In summary, analyses of trial evidence have concluded that the addition of routine POC tests to standard care will reduce transfusion rates and be cost-effective. The evidence from predictive accuracy studies suggests that these tests may discriminate between patients who will develop severe bleeding and those who will not. There is considerable uncertainty around these findings, however, because of variable quality of the evidence. This uncertainty is reflected in the wide variation in the use of POC tests in UK cardiac surgery centres. To address this uncertainty and to provide high-quality evidence to inform treatment decisions we conducted the COPTIC B study.
Rationale for the COPTIC B study
We hypothesised that the routine use of POC tests in all patients, including TEG, ROTEM and the Multiplate test, would have important clinical benefits beyond those attributable to the current standard of care plus appropriate preoperative risk assessment. We also hypothesised that clinical benefits would be achieved by enabling early and accurate diagnosis of coagulopathy and the early targeted treatment of patients who are likely to develop coagulopathic haemorrhage.
The COPTIC B study considered whether routine pre- and postoperative POC tests would have predictive accuracy for the development of coagulopathic haemorrhage and whether the routine use of these tests would be cost-effective if introduced alongside standard care.
The COPTIC B study evaluated both TEG and ROTEM. We hypothesised that ROTEM would have better predictive accuracy than TEG because it has greater specificity for some common coagulopathies in cardiac surgery, such as fibrinogen deficiency. TEG and ROTEM have not previously been compared directly in cardiac surgery. We also evaluated the additional benefits of using the Multiplate test in isolation or in combination with ROTEM and TEG. We hypothesised that this would further improve discrimination as suggested by the systematic review of predictive accuracy studies.25 We hypothesised that any additional benefit would be attributable to the added value of a test that can detect the effects of antiplatelet drugs and discriminate between platelet dysfunction and fibrin deficiency.79
Finally, if coagulopathic haemorrhage has a causal relationship with adverse clinical outcomes, it is sensible to suggest that POC tests that improve clinical prediction for bleeding may also have the potential to predict adverse clinical outcomes. This has not previously been investigated. We tested this hypothesis in an exploratory analysis within the COPTIC B study.
Reference test analysis
In addition to our evaluation of POC tests we also considered whether the predictive accuracy of POC testing would be improved by the use of additional LRTs to identify coagulopathies that are not detected or which are misclassified by the TEG and ROTEM assays. Assay systems that measure fibrinogen, D-dimer, vWF and factor XIII concentrations as well as assays of anti-Xa activity and thrombin generation are now available commercially. Additional data on platelet size and maturity are routinely measured using automated cell counters as part of standard care and may also have clinical utility. Introduction of these tests, or the development of rapid testing for these assays, may permit detailed investigation of specific coagulopathies in cardiac surgery and improve the predictive accuracy of current tests. However, there is no high-quality evidence available on which additional assays should be performed and whether the potential increase in patient benefit from performing additional assays justifies testing. To address this issue we therefore assessed the potential benefits of introducing a range of LRTs of coagulopathy alongside the current standard of care.
If our analyses identified significant improvements in predictive accuracy with the use of either POC or LRTs it was our intention to assess the relationship between diagnostic error and clinical outcomes, including bleeding, and use these data to generate alternative management algorithms that consider the value of additional reference tests. The potential clinical and economic benefits of these refined algorithms would then be estimated by modelling using the clinical and resource use data collected in the study combined with NHS costs obtained from reference sources.
Systematic review
We identified important limitations in the evidence used by NICE when recommending that viscoelastic POC devices enter routine use in adult cardiac surgery.23,26 To address the limitations of the evidence used to support the current NICE guidance, and to place the results of the COPTIC study in context, we undertook a systematic review of RCTs that have assessed the clinical efficacy of viscoelastic tests in cardiac surgery. We assessed important clinical outcomes included kidney injury, stroke and prolonged respiratory support and we summarised the evidence using the GRADE (Grades of Recommendation, Assessment, Development and Evaluation) approach (GRADEpro version 3.6 for Windows; Jan Brozek, Andrew Oxman and Holger Schünemann, McMaster University, 2008).
COPTIC study aim and objectives
Study aim
The aim of the COPTIC study was to estimate patient benefit from the measurement of coagulation factors and platelet function using POC testing platforms in common use or a range of LRTs in patients undergoing cardiac surgery.
Study objectives
The specific study objectives common to each analysis were as follows:
- determine the clinical characteristics of participants who develop the reference outcome CCB, a composite outcome of objective measures of clinically important bleeding, as defined in Definition of study end points, compared with the clinical characteristics of those who do not develop CCB
- characterise the association between prespecified clinical characteristics and CCB, with specific consideration of the effects of DAPT administration in close proximity to surgery
- characterise the distributions of a range of index tests from POC devices in common clinical use or a range of LRTs in participants with and without CCB
- develop a clinical risk prediction model for CCB using baseline clinical data and quantify its predictive accuracy for CCB
- evaluate the benefits of introducing the results from separate platforms to the clinical prediction model, either as single tests or as combinations of different testing platforms
- develop a clinical risk prediction score for a range of secondary clinical outcomes using baseline clinical data and quantify its predictive accuracy
- compare these results with the predictive accuracy of the clinical risk prediction score plus the results of index tests, either as single tests or as combinations of different testing platforms.
Methods
Overview
We carried out a prospective, single-centre observational cohort study to test the predictive accuracy of a range of diagnostic tests for coagulopathic bleeding in adult cardiac surgery. A schematic of the timings of the (index) test samples and the determination of reference outcomes is shown in Figure 6. We collected venous blood samples pre- and postoperatively (times T0 and T3 respectively) and conducted a range of POC and laboratory tests on these samples. The primary (reference) outcome of the study was an estimate of the frequency of coagulopathic haemorrhage, termed CCB, which is defined in Definition of study end points. Our analyses considered the importance of key preoperative predictors of bleeding that could be used to estimate the risk of CCB without the need for diagnostic tests. We then estimated the additional value of the index test results for the prediction of CCB. We considered all participants as well as the subgroup of participants receiving P2Y12 receptor blockers preoperatively, those receiving aspirin and those receiving no antiplatelet therapy. The study included three distinct analyses:
- COPTIC A. This analysis considered the benefits of the measurement of preoperative platelet function using Multiplate aggregometry.
- COPTIC B. This analysis considered the benefits of postoperative POC tests including TEG and ROTEM with or without pre- and postoperative measurement of platelet function using the Multiplate test.
- LRTs. This analysis considered the benefits of:
- preoperative measurements of red cell mass and platelet morphology derived from automated cell counters (LRT analysis 1)
- preoperative and postoperative data on red cell mass and platelet morphology derived from automated cell counters and LRTs of coagulation (LRT analysis 2).

FIGURE 6
Timeline indicating research procedures undertaken in the COPTIC study. CICU, cardiac intensive care unit.
The LRT analyses were initially intended to complement the COPTIC B analysis; however, this did not occur because of the poor predictive accuracy of the LRTs.
The study was conducted in accordance with the International Conference for Harmonisation of Good Clinical Practice guidelines, the Research Governance Framework for Health and Social Care, the European Union Directive 2001/20/EC on clinical trials, the Data Protection Act 199896 and the Human Tissue Act 2004.97 The research was conducted as a partnership between the Bristol Heart Institute and the University of Bristol, UK. The study was sponsored by the University Hospitals Bristol NHS Foundation Trust and authorised by a UK NHS research ethics committee (REC) (reference 09/H0104/53) and was registered prospectively (ISRCTN20778544). The study protocol is available along with this report online as Report Supplementary Material 1. Changes to the study design after commencement of the study are listed in Appendix 1. The study is reported in accordance with the STARD (Standards for Reporting Diagnostic Accuracy) statement98 (see Appendix 1).
Study population
Study setting
The study was conducted in the Bristol Heart Institute, University Hospitals Bristol NHS Foundation Trust, a tertiary cardiac surgery centre in the UK.
Study population
Consecutive adult patients undergoing cardiac surgery that necessitated opening the pericardium were assessed for eligibility according to the following criteria:
- inclusion criteria:
- aged > 18 years
- undergoing cardiac surgery at Bristol Heart Institute
- exclusion criteria:
- prisoners
- patients unable to give prospective or retrospective consent through mental incapacity.
Characteristics of non-study patients
To evaluate the representativeness of the study participants, demographic and clinical data were compared between the following groups:
- ineligible patients
- eligible patients who were not approached
- eligible patients who were approached but who did not consent
- consented patients who did not provide a postoperative blood sample
- consented patients who did provide a postoperative blood sample for which at least one laboratory test result was available.
The reasons why eligible patients were not approached, why eligible patients refused consent and why samples were not taken from consenting patients were documented. Formal statistical comparisons between the final analysis group and those eligible and not included in the analyses were undertaken using t-tests, Mann–Whitney tests and chi-squared tests as appropriate. Comparisons between all other groups were descriptive.
Withdrawals
Participants (or clinicians on their behalf) could withdraw from the study at any time post consent (including prior to surgery). The data available for analysis depended on the timing of withdrawal (see Figure 6). Data collected up to the point of withdrawal were retained for analysis.
Protocol deviations
The protocol stated that all research samples should be collected after the return of cell saver and/or pump blood and after all ‘routine’ protamine doses had been administered. All samples that were recorded as not being collected at this specific time were reviewed and excluded if necessary. The overlap with the return of pump blood was of particular concern as this is known to alter coagulation.
Analysis populations
The analysis population was defined as all eligible, consenting participants who provided a pre- and/or postoperative blood sample for testing. The final data sets were restricted to complete cases, those with laboratory assay results from the research blood samples and case report form and clinical data extracted from the hospital information systems – the cardiac surgery Patient Analysis and Tracking System® (Dendrite Clinical Systems, Henley-on-Thames, UK) and the intensive care Innovian® data management system (Drager Medical Inc., Telford, PA, USA) – to define the primary outcome. Consenting participants who had a research blood sample collected but who did not undergo surgery for medical or other reasons were excluded from the analysis population. The risk of bias was examined by comparing the characteristics of participants retained and excluded from the proposed analysis population. Effect sizes in the form of standardised mean differences (SMDs) were calculated. These can be interpreted as follows: SMDs of 0.20 are ‘small’ in magnitude, those around 0.50 are ‘medium’ and those ≥ 0.80 are ‘large’.99 Involvement in any other Bristol Clinical Trials and Evaluation Unit studies was also reviewed and any interventions deemed to have an impact were reported.
Definition of study end points
Primary outcome (reference outcome)
As discussed in Coagulopathic bleeding there is no consensus definition of coagulopathic haemorrhage that could serve as the reference outcome. Anticipating the limitations of existing outcomes we used a novel composite definition of postoperative CCB, that is, bleeding that was considered likely to cause direct patient harm or indirect patient harm by precipitating a non-routine pro-haemostatic treatment or reoperation for bleeding for which no direct surgical cause was identified. A participant was classified as having experienced the primary outcome of postoperative CCB if any of the three circumstances below was documented within the designated time interval:
- Postoperative blood loss (volume collected through the chest drain) of > 600 ml at 6 hours post protamine reversal of heparin (between T3 and T5; interval 2a in Figure 6). It was considered that drain losses after this time may not represent active bleeding.
- Intervention with a non-routine early pro-haemostatic treatment, defined as additional protamine after heparin reversal (this did not include additional protamine administered routinely after re-transfusion of pump blood), FFP, cryoprecipitate, platelets, antifibrinolytic drugs, rFVII, prothrombin complex concentrate or fibrinogen concentrate during interval 2b (T3–T6; see Figure 6).
- Reoperation for bleeding recorded during the hospital stay and failure to demonstrate a surgical cause for bleeding, as recorded in the operation record.
For the COPTIC A study a modification of this definition was used. As a preoperative test could potentially influence pro-haemostatic treatments administered at any time during the course of the operation, it would be more correct to consider any such treatment administered after the start of the procedure (intervals 1 and 2, between T0 and T6; see Figure 6) in addition to treatment as in the postoperative CCB definition. For the purposes of clarity this is referred to in the analyses as any CCB.
Secondary clinical outcomes
Secondary clinical outcomes and the data sources used to identify these are listed in Table 4. Red cell transfusion of > 4 units has been used as a definition of coagulopathic bleeding in previous studies;10 however, because red cell transfusion is also likely to reflect the treatment of anaemia in addition to the treatment of bleeding, it was recorded as a secondary outcome in the COPTIC study.
TABLE 4
Secondary end point definitions
Test predictors
Predictors from index POC tests are listed in Table 5, predictors from index differential cell counter tests are listed in Table 6 and predictors from index SLTs and LRTs are listed in Table 7.
TABLE 5
Predictor variables from index POC tests
TABLE 6
Predictor variables from index differential cell counter tests
TABLE 7
Predictor variables from index SLTs and LRTs of coagulation
Timing of test results
We evaluated the potential advantages of using early test results for each assay when these were available (Table 8). The early time frame tests have never been incorporated into POC testing algorithms developed empirically by others in cardiac surgery. However, they may be more useful clinically by virtue of providing informative test results more quickly than ‘standard’ tests. Models were fitted first for the standard time frame. Then, models were fitted for the early time frame, testing whether this resulted in a ‘significant’ deterioration in prediction. Comparing early and standard time frames is interesting because there may be a trade-off between the potential benefits of earlier decision-making (e.g. allows definitive treatment to be provided more quickly, thereby preventing blood loss) and the potential harms (e.g. somewhat less good prediction). For each platform, the test results were classified as being relevant to an early or ‘standard’ time frame, for instance:
- Multiplate:
- early = velocity
- standard = AUC, amplitude
- ROTEM:
- early = A10 or A5 [amplitude 10 or 5 minutes after clotting time (CT)]
- standard = MCF variables (measurements 20 minutes after starting the analysis)
- TEG:
- early = no immediately available measurements comparable with those for ROTEM (but available in principle)
- standard = MCF variables (measurements 20 minutes after starting the analysis).
TABLE 8
POC complete and corresponding early test parameters
Research procedures
Patient recruitment
Consecutive patients undergoing cardiac surgery at the Bristol Heart Institute during the recruitment period were screened for eligibility and approached for consent when research staff were available. Some patients were not approached because admission occurred outside normal working hours or because staff members were on leave. Participants provided informed consent on admission to hospital. In the rare event of a patient with diminished capacity being unable to physically complete the consent form, verbal consent was accepted and this was documented on the trial-specific consent form and in the medical notes. The person taking consent and the participant (or someone in a qualifying relationship) were required to sign the request for participation. This was agreed with the REC and the sponsor, as it was recognised that critically ill patients undergoing emergency surgery are among those most likely to benefit from improved diagnosis and treatment of coagulopathic bleeding. All recruited participants ultimately provided informed consent for enrolment in the study.
Blood sampling
For the research, two 22.5-ml blood samples (45 ml in total) were obtained in the operating theatre at the following time points (see Figure 6):
- immediately before induction of anaesthesia (T0)
- on reversal of heparin anticoagulation (T3).
All blood samples were taken from existing arterial lines inserted as part of standard clinical care. Blood samples for MEA platelet function testing were collected into 3-ml vacuum collection tubes containing hirudin (final concentration 15 µg/ml). Blood samples for TEG, ROTEM and SLTs and LRTs were collected into 4.5-ml vacuum collection tubes containing trisodium citrate (final concentration 0.105 M). The MEA, ROTEM and TEG tests were analysed in a research laboratory specifically staffed and equipped for the COPTIC study using whole blood within 120 minutes of blood collection. For the reference tests, platelet-poor plasma was prepared by centrifugation of the blood samples at 2240 g for 10 minutes. The plasma was then decanted into a new specimen tube before recentrifugation using the same conditions. Plasma samples were then stored in aliquots at –70 °C for analysis in batches.
Rotational thromboelastometry
For the ROTEM tests, 300 µl of anticoagulated blood was recalcified in the reaction cup with 20 µl of star-TEM® reagent and was then activated with the following reagents in accordance with the manufacturer’s instructions: (1) EXTEM reagent, (2) FIBTEM reagent, (3) INTEM reagent and (4) HEPTEM reagent (all reagents manufactured by ROTEM, TEM International GmbH, Munich, Germany). Changes in clot viscoelastic strength were measured for 60 minutes. Analyses were performed on two ROTEM Delta devices.
Thromboelastography
For the TEM tests, 20 µl of 0.2 M CaCl2 was added to each TEG cup. In accordance with the manufacturer’s instructions, 1 ml of anticoagulated blood was added to kaolin reagent TEG bottle and mixed gently. For each test, 340 µl of this mixture was then added to either the plain or the heparinase-coated TEG cups. Changes in clot viscoelastic strength were measured for 60 minutes. Analyses were performed on two TEG® 5000 Thromboelastograph® Haemostasis Analyzer Systems (Haemonetics Corporation, Niles, IL, USA).
For both the ROTEM and the TEG analyses, traces that were identified as being of poor quality during the initial analyses were immediately repeated using spare blood samples. After completion of the assays, numerical parameters derived from the TEG curves by the device automated algorithms were inspected manually. For traces in which the derived parameters had not been correctly recorded, correct estimates were obtained manually by inspection of the x,y co-ordinates of the raw viscoelastometry traces. ROTEM and TEG parameters that were absent or incorrect and which could not be resolved by this method were excluded from the analyses.
Multiplate
The hirudinised blood was first mixed 1 : 1 with 0.9% NaCl preheated to 37 °C in standard test cells on a Multiplate multiple electrode aggregometer according to the manufacturer’s instructions. After 3 minutes’ incubation, platelet activation was initiated by the addition of the following agonists: (1) ADPtest reagent (ADP final concentration 6.5 µM), (2) ASPItest reagent (arachidonic acid final concentration 0.5 mM), (3) TRAPtest reagent (TRAP final concentration 32 µM) and (4) ADRENtest [adrenaline (epinephrine) final concentration 20 mg/ml]. The increase in electrical impedance from the two electrode pairs within each test cell was recorded for 6 minutes and was expressed as a mean of the AUC measurements in arbitrary units of AU*min, converted to U (where 1 U = 10 AU*min) to enable comparison with other clinical studies. Internal MEA quality control software generates an error flag if the difference in AUC measurements between the two electrode pairs exceeds 20% of the mean of the AUC measurements. In this circumstance, the assay was immediately repeated using the same blood sample. Mean AUC measurements were excluded from the analyses if repeat testing also failed to generate a result within this quality control threshold.
Laboratory reference tests
The reference tests for soluble coagulation factor activities and clotting times were all performed in batches on thawed plasma aliquots using standard manufacturer’s protocols on a Sysmex CS2100i analyser. Differential cell counts were performed on a Sysmex XE-2100 automated haematology analyser. The following test reagents were used: PT – Innovin®; aPTT – Actin FS; Clauss fibrinogen activity – thrombin reagent; vWF – ristocetin cofactor activity (BC RiCof assay); factor XIII activity – Berichrom XIII; and D-dimer concentration – INNOVANCE® (all from Sysmex, Kobe, Japan). The anti-Xa assay was performed using the HYPHEN BioMed anti-Xa kit, using an unfractionated heparin standard. Thrombin generation was measured using a Fluoroskan Ascent™ device (ThermoFisher Scientific, Paisley, UK) and the FluCa-kit (Stago, Asnières sur Seine, France), according to the manufacturer’s instructions.
Measurement of clinical outcomes
Clinical outcomes as described in Definition of study end points were measured as follows. Blood loss at 6 hours, non-routine administration of protamine and administration of non-routine pro-haemostatic treatments during surgery (T0–T3, interval 1; see Figure 6) were recorded on case report forms. Reopening for bleeding, MI, stroke, acute kidney injury (AKI) requiring dialysis, wound infection and death were recorded on the hospital’s Patient Analysis and Tracking System. Baseline participant demographics, comorbidity and operative details were also recorded on the Patient Analysis and Tracking System. Non-routine early pro-haemostatic treatment, reopening for bleeding, MI, stroke, AKI requiring dialysis and sepsis were recorded on the intensive care Innovian data management system. Serum creatinine data required for the estimation of AKI were obtained from the hospital’s clinical biochemistry database. Details of data extraction and coding are recorded in the statistical analysis plans (available online in Report Supplementary Materials 2 and 3).
Duration of follow-up
The duration of follow-up was until hospital discharge or death.
Clinical management of study subjects
Participants underwent standard preoperative, anaesthetic, surgical and postoperative care according to existing unit protocols. Decisions about intra- and postoperative haemostasis and transfusion treatment were guided by ad hoc TEG analyses and standard laboratory investigations (PT, aPTT, ACT, fibrinogen, platelet count), performed at the discretion of the responsible clinician in accordance with routine institutional practice. These decisions were not influenced by participation in the study.
Measures taken to avoid bias
Selection bias was minimised by selecting consecutive participants who satisfied the prespecified inclusion criteria. There were few exclusion criteria and this was intended to select a population that was as representative as possible of the reference cardiac surgery population. Selection bias was also minimised by the development of a dedicated COPTIC laboratory, to ensure that all consented participants were recruited and samples analysed. We tested for selection bias by comparing the characteristics of patients who were recruited with the characteristics of those who were not recruited.
Index tests included POC tests, SLTs in common use for the management of coagulopathic bleeding in cardiac surgery and LRTs that can diagnose common acquired coagulopathies in cardiac surgery. The timing of the tests corresponded to critical stages in the patient journey when these tests are commonly performed. Interventions based on pre- and postoperative POC and coagulation tests have been shown to reduce coagulopathic bleeding in cardiac surgery patients. All tests were performed in a laboratory that was separate from the site of clinical care of the study subjects and almost all tests were carried out by staff who were blinded to the clinical course of the patients. Quality assurance steps were taken on an ongoing basis to ensure adequate data quality. Laboratory test results were unavailable to the clinicians responsible for direct patient care and therefore did not influence the clinical management of participants or the reference outcome.
We used a novel definition of the primary (reference) outcome CCB. We considered that this had important advantages over definitions of coagulopathic bleeding used in previous studies. We also evaluated the robustness of our primary analysis by considering alternative definitions of CCB in sensitivity analyses.
Data collection and linkage
Almost all data were collected from routine sources. Data required to characterise (1) participants preoperatively, (2) operations and (3) postoperative complications were collected through the audit infrastructure currently in place for all patients undergoing cardiac surgery (Patient Administration and Tracking System) and the intensive care unit (ICU) Innovian data management system. All SLT and reference test data and TEG, Multiplate and ROTEM data were stored in electronic laboratory databases until linkage. Data describing transfusions (red cells, platelets, FFP) were obtained from the hospital blood bank. Creatinine measurements for classification of AKI were obtained from laboratory data collected for routine clinical care. A minimal number of data not available from the above sources (e.g. blood products in theatre given prior to sample collection and blood loss at 6 and 12 hours post admission to intensive care) were collected from medical records or postoperative care charts and recorded on case report forms. Information on treatment taken (aspirin, clopidogrel, prasugrel or a combination) and the time that treatment was stopped was recorded using the Patient Analysis and Tracking System. The data were linked for analysis on the basis of a pseudonymised participant identifier, that is, hospital number, in addition to the date of operation (Figure 7). Further details of the data linkage procedures are listed in the statistical analysis plans (available online in Report Supplementary Materials 2 and 3). Data were collected and retained in accordance with the UK Data Protection Act 1998.96

FIGURE 7
Sequence of linkage of data sources. BRI, Bristol Royal Infirmary; CICU, cardiac intensive care unit; CRF, case report from; FBC, full blood count.
Storage of clinical material
To enable retrospective analysis of confounding variables and for laboratory quality control and assay validation purposes, blood samples not used in the initial analyses were stored at –80°C in a secure storage facility in the hospital laboratory for the duration of the study.
Sample size considerations
The study sample size detailed in the protocol (version 6, 21 March 2012) was 2400, which corresponds to the entire predicted patient throughput at Bristol Heart Institute for the 2-year study period, allowing for up to 10% of patients to opt out. We estimated that a sample size of 2400 would have > 95% power to detect significant associations between continuous exposures and outcomes at a two-tailed significance level of 0.01. As an example, assuming that (1) the prevalence of clopidogrel withdrawal < 5 days from surgery is 50% and (2) the overall risk of excess bleeding is 15% (historical Patient Analysis and Tracking System data for Bristol), the study would have about 90% power to detect a RR of developing the primary outcome of about 1.7 (11% vs. 19%) at a two-tailed significance level of 0.05 in the subgroup of participants (approximately 800) taking clopidogrel prior to surgery.
More recent data extraction from the Patient Analysis and Tracking System led to a review of this estimate. This demonstrated that the proportion of patients on clopidogrel in a more contemporary cohort was 29% (estimated as 33% in the previous example), the proportion of patients who have clopidogrel withdrawn within 4 days of surgery was 38.6% (estimated as 50% in the previous example) and the excess bleeding rate was 13.1% (estimated as 15% in the previous example). Using these figures, we estimated that the COPTIC study would now have 84% power to detect a 1.9-fold increase in the risk of excess bleeding between those who had clopidogrel withdrawn and those who did not (incidence of excess bleeding 9.03% vs. 17.17% respectively) at a two-tailed significance level of 0.05. This revised estimate did not indicate that a change in the sample size was required to meet the study objectives.
Plan of analysis
Overview
The objective of the COPTIC analyses was to estimate how well the results of POC blood tests, or LRTs, assist in the prediction of CCB. This was achieved by developing predictive models for the reference outcome CCB, initially using variables related to existing participant characteristics. Index (predictor) POC or LRT variables were then included in refined models and the predictive value compared with that of models using participant characteristics alone. Differences in predictive accuracy were expressed as differences in the AUC or the number of participants reclassified as having the reference outcome. Finally, we fitted separate regression models to estimate associations between the results of POC tests and secondary outcomes and developed models that would be informative in predicting the risk of each secondary outcome according to POC test results. Statistical analyses and reporting were undertaken in accordance with the STARD guidelines,98 as described in Appendix 1.
Clinical prediction model
To develop a clinical prediction model for CCB that would complement the current standard of care we prespecified a priori expected relationships between a series of baseline participant characteristics and an increased likelihood of CCB. This included whether or not participants were receiving DAPT, aspirin alone or no antiplatelet treatment. The DAPT subgroup was also further subdivided into those participants who had stopped their P2Y12 receptor blocker > 7 days before surgery, 6–7 days before surgery, 3–5 days before surgery or 0–2 days before surgery. This was stratified by the type of cardiac surgery. Cardiac procedures are recorded and categorised in the Patient Analysis and Tracking System as CABG (coronary artery bypass graft) only, CABG and valve replacement surgery, CABG and other, CABG and valve replacement surgery plus other, valve replacement surgery alone, valve replacement surgery plus other and other procedure. These three factors (DAPT, timing of withdrawal and type of operation) co-vary with one another in that the treatments of interest (DAPT and timing of withdrawal) are not necessarily relevant to all cardiac procedures. Therefore, we considered these factors by making a categorical variable that coded clinically relevant combinations. The nature of the ‘other’ cardiac procedures was heterogeneous and required additional characterisation. Any combination that included an ‘other’ procedure was reviewed and classified according to whether or not the ‘other’ procedure was considered to be the primary determinant of the risk of bleeding (from the point of view of the operation being carried out). Participants undergoing an ‘other’ procedure classified as being the determinant of bleeding became a new subset; all participants with an ‘other’ procedure were reclassified according to their main procedure, for example ‘CABG and other’ was reclassified as ‘CABG only’. If the only procedure was an ‘other’ procedure judged to have a high bleeding risk, it was classified with the new ‘other’ subset; if it was judged to have a low bleeding risk, for example atrial fibrillation radiofrequency ablation, the participant was excluded from the analysis population. The ‘other’ procedures identified as having a high bleeding risk were K08 ‘Repair of double outlet ventricle (Clean)’, K33 ‘Aortic root replacement’ and L19 ‘Other replacement of aneurysmal segment of aorta’. The new categories combining the treatment group, time since withdrawal of antiplatelet therapy and cardiac procedure are as follows [reducing a three-dimensional matrix of four cells (cardiac procedure) × three cells (treatment group) × three cells (time since withdrawal)], as shown in Box 3.
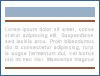
BOX 3
Baseline characteristics preselected as potential predictors of CCB
Specific analysis procedures
We evaluated the benefits of introducing the results from separate platforms to the clinical prediction model [Multiplate (preoperative), ROTEM, TEG, Multiplate (postoperative)]. Platforms were added individually in the first instance. We then evaluated whether the inclusion of additional platforms would improve the prediction of CCB. The reason for considering groups of variables by platform (rather than simply choosing the ‘best’ subset of variables) was that there is no cost of using extra variables from one platform, but there is an extra cost of carrying out a second test.
The specific steps undertaken were as follows. In step 1 we fitted regression models to estimate associations between the results of laboratory tests and CCB. For all continuous terms the linearity of any potential associations was investigated using multivariable fractional polynomial (FP) models. If the model fit improved by transformation of the term, this was performed. The prediction achieved using participant characteristics was determined and an optimal model based only on ‘baseline’ covariates was defined. Separate models were developed for any CCB (intraoperative or postoperative) for the COPTIC A study and the evaluation of the LRTs and for postoperative CCB for the COPTIC A and B studies and the evaluation of the LRTs.
In step 2 we reviewed the predictive accuracy of the POC test results for ROTEM, TEG and Multiplate to obtain the best-performing model for each (highest AUC), including the baseline covariates. First, the contributions of all of these baseline characteristics to the prediction of CCB were incorporated into a single score, which was forced into models of laboratory predictors with a coefficient of 1.00 to enable comparisons between different models based on the various laboratory test platforms. Next, all of the test results from each device were included for each model, with multivariable FP techniques used to investigate the linearity of terms. All transformations and centring found to improve the fit of the models were implemented. Backward elimination was undertaken to select the final models, with a threshold of inclusion of 0.05 and a threshold of exclusion of 0.10. Initial models considered only the standard test results (see Tables 6 and 7); subsequently, the ‘early-phase’ alternatives were considered for any terms that significantly contributed to the model. Goodness of fit for models of baseline characteristics and best-performing models was assessed using the Hosmer–Lemeshow test and by calculating Brier scores.
The following models were constructed (Figure 8):
- model 2A – preoperative Multiplate platform in relation to any CCB
- model 2B – preoperative Multiplate platform in relation to postoperative CCB
- model 2C – postoperative Multiplate platform in relation to postoperative CCB
- model 2D – postoperative ROTEM in relation to postoperative CCB
- model 2E – postoperative TEG in relation to postoperative CCB.

FIGURE 8
Summary of analyses of preoperative and postoperative tests.
Each model was then compared with a baseline model containing only the baseline characteristics to evaluate the contribution of the selected laboratory test results to the prediction of CCB. These analyses are presented as receiver operating characteristic (ROC) curves. We hypothesised that each of these models would consider all variables for the test/time point, that is, consider the test/time point as a ‘package’. The first model stands alone for any CCB. For postoperative CCB, this step will identify which of the other four models is the best platform for predicting CCB, that is, provides the best prediction. We hypothesised that the best platform would one of the postoperative platforms because the information from these is more ‘proximal’ to the outcome for these models, that is, postoperative CCB. For the best test/time point we also investigated whether a model using test variables that are available early (for the test identified as best) performs as well as a model using the standard test variables available at ‘test completion’.
In step 3 we tested whether the addition of one of the other (postoperative) platforms would improve the best-performing model for postoperative CCB (highest AUC) identified in step 2. We expected, on the basis of existing data, that this would be a test of whether or not addition of the postoperative Multiplate platform improves prediction compared with either ROTEM or TEG alone (or the addition of ROTEM or TEG to the postoperative Multiplate platform, as ROTEM and TEG assess similar mechanisms and the Multiplate platform assesses different mechanisms). The combination of ROTEM and TEG together was not considered. For the test/time point added at step 3, we also investigated whether or not a model using test variables that are available early (for the test/time point added at step 3) performed as well as a model using the standard test variables available at ‘test completion’.
In step 4 we tested whether or not the addition of the preoperative Multiplate platform would improve the model identified in step 3. If the preoperative Multiplate platform was added at step 4, we also planned to consider whether or not a model using test variables that are available early for the preoperative Multiplate platform performs as well as a model using the standard test variables available at ‘test completion’.
Finally, for the best-performing predictive model, the predictive value was expressed as the proportion of participants correctly classified as having CCB or no CCB, with those with a fitted probability of CCB of ≥ 0.5 classified as having CCB. The predictive value was also described by the change in post-test probability conditional on the test results in the model, as a function of pre-test probability.100 The net reclassification improvement (NRI) was calculated. This index quantifies how well the best-performing predictive model reclassifies subjects – either appropriately or inappropriately – compared with the baseline characteristics model.
All analyses were undertaken using Stata® version 14 (StataCorp LP, College Station, TX, USA).
Sensitivity analyses
We undertook sensitivity analyses to address uncertainty in relation to the reference outcome CCB. These sensitivity analyses were specified in the context of there being a large proportion of participants classified as having CCB because they received one or more pro-haemostatic treatments but who did not experience excessive bleeding (chest drain < 600 ml at 6 hours post operation) or did not require a reoperation. Anecdotal evidence suggests that it is common for one pro-haemostatic agent (1 unit of pooled platelets or 2 units of FFP) to be administered to participants considered to be at high risk of bleeding in the absence of evidence of bleeding (i.e. a priori concern about bleeding). Therefore, the sensitivity analyses were intended to explore whether discrimination on the basis of platelet function might be improved by excluding this group that contained an unknown (and unknowable) proportion of participants in whom pro-haemostatic treatment had been administered without evidence of bleeding or evidence to cause concern about bleeding. Two sensitivity analyses were prespecified:
- 1.
Sensitivity analysis 1 (SA1). Those defined as having received early pro-haemostatic treatment but who did not experience excessive bleeding (chest drain < 600 ml at 6 hours post operation) or require reoperation for bleeding were excluded from the analyses and the models and ROC curves detailed in Specific analysis procedures were repeated.
- 2.
Sensitivity analysis 2 (SA2). Those defined as having received early pro-haemostatic treatment but who did not experience excessive bleeding (chest drain < 600 ml at 6 hours post operation) or require reoperation for bleeding were included in the analyses but were reclassified as being free from CCB.
In addition, we performed an additional post hoc sensitivity analysis:
- 3.
Sensitivity analysis 3 (SA3). Those defined as having received only a single treatment of early pro-haemostatic treatment defined as 1 pooled unit of platelets and 2 units of FFP were included in the analysis but reclassified as free from CCB. The resulting CCB definition was considered to have reflected more severe coagulopathy i.e. not responsive to initial early pro-haemostatic treatment.
Internal validation
The AUC statistics from the primary outcome models were internally validated by bootstrapping with 1000 replicates and cross-validated by removing one observation and then using the remaining study population to create models that generated the predicted probability of CCB for that one observation. After repeating for each observation, the predicted probabilities were used to build ROC curves and to calculate AUC statistics.
External validation
The baseline-only model was validated in a separate cohort of adult cardiac surgery participants (n = 1611) recruited to the multicentre TITRe2 trial between July 2009 and February 2013,4 excluding participants at the Bristol Heart Institute. The data for this cohort did not exactly match those collected for the COPTIC cohort in the following ways: (1) the preoperative platelet count was not measured in the TITRe2 trial; (2) blood loss was not measured at 6 hours post operation and so was interpolated from measurements at 4 and 12 hours; (3) procedure type and antiplatelet medication were not recorded in identical ways and (4) non-standard care pro-haemostatic treatments were recorded for the hospital admission in the TITRe2 trial, which was longer than the observation interval in the COPTIC study. To accommodate these differences, small changes were made in the baseline-only model to enable comparison between the cohorts.
Secondary outcomes
Coagulopathic bleeding is strongly associated with adverse outcomes. To assess whether or not prediction of bleeding risk may also translate into accurate prediction of a range of adverse clinical outcomes we developed baseline clinical predictive models for our prespecified secondary outcomes. Steps 1–4, as described earlier, were then repeated to assess whether or not the addition of the postoperative POC test results evaluated in the COPTIC B study would improve the discrimination of these models.
Analyses of laboratory reference tests
Using the COPTIC B data set we performed two separate analyses for the LRTs.
In LRT analysis 1 we developed a baseline clinical prediction score for the reference outcome any CCB, as described in Clinical prediction model. Next, we repeated steps 1 and 2, described earlier, to identify the final model. In LRT analysis 2 we included the results of multiple tests and multiple testing platforms. In this analysis we repeated steps 1–4, as described earlier. Sensitivity analyses (SA1–3) were also performed to test uncertainty in relation to the reference outcome, as described earlier.
Statistical analysis plans
The statistical analysis plans were written prior to the analyses being carried out. Separate statistical analysis plans were written for the preoperative, postoperative and reference test analyses (available online in Report Supplementary Materials 2, 3 and 4).
Results
The results of the predictive accuracy study are considered in two parts. The analyses of the COPTIC A and B studies are reported together. The analysis of the reference tests was originally intended to complement the COPTIC B analysis to assess whether or not the addition of specific reference tests would improve the predictive accuracy of the best-performing model developed using the POC tests (highest AUC). This analysis was not performed because of the poor predictive accuracy of all of the models and therefore the results of the reference tests are reported separately.
Study and analysis cohorts
A total of 3638 participants were identified as being eligible for the study between 30 March 2010 and 31 August 2012, of whom 2541 (69.8% of those who were eligible) provided consent to participate (Figure 9). Among the 1097 participants who did not consent to participate, the proportion undergoing other (9.2% vs. 2.5%; SMD 0.32) or urgent (46.7% vs. 35.7%; SMD 0.23) procedures was higher. Other differences were considered small (SMD < 0.2) (Table 10).
TABLE 10
Characteristics of consented compared with non-consented participants
In the COPTIC A analysis of preoperative test results 2435 participants had a preoperative sample collected, of whom 2427 had both the sample analysed and the primary outcome recorded. In total, 344 participants were not included in the analysis because of incomplete baseline or laboratory data, leaving an analysis population of 2197 participants. The analysis population had a median age of 67.3 years [interquartile range (IQR) 61.7–75.7 years] and included 1674 males (76.2%). Most of the analysis population underwent CABG with aspirin treatment (38.4%) (Table 11). Among the 344 participants who were excluded from further analysis because of incomplete data, the proportion of men was lower (67.2%; SMD 0.21). The proportion of participants undergoing CABG and valve replacement surgery with aspirin treatment was higher among the participants not included in the analysis (9.9% vs. 7.2%; SMD 0.10), as was the proportion of participants undergoing CABG only who had been taking DAPT within 6–7 days of surgery (7.0% vs. 4.7%; SMD 0.11) and the proportion of patients in the ‘other’ (high-risk) group (7.6% vs. 5.1%; SMD 0.11). Otherwise, the baseline characteristics of these groups were similar. Given that the differences between the groups were small (defined as a SMD of < 0.2), with the exception of male sex (SMD 0.21), we concluded that the analysis population was representative of the entire study population (see Table 11).
TABLE 11
The baseline characteristics of the analysis population and the population of participants who were consented but who were excluded from the COPTIC A analysis because of incomplete baseline characteristics or near-patient test result data
In the COPTIC B analysis, of the 2433 participants for whom a postoperative sample was collected, 202 were excluded because of incomplete POC test data or components of the primary outcome, leaving 2231 participants for whom both the sample was analysed and the primary outcome was reported. For the comparison of a predictive model that incorporated the baseline characteristics of the participants and alternative models that also included all preoperative and postoperative test results, a further 399 participants were excluded for incompleteness of baseline data, outcome data or preoperative or postoperative test data (see Figure 9). The primary analysis was restricted to the 1833 participants (72.1% of those consented) with a complete set of baseline characteristics and predictor test results. The analysis population had a median age of 68.9 years (IQR 61.9–75.7) and included 1389 males (75.8%). Most of the analysis population underwent CABG with aspirin treatment (40.2%), valve replacement without antiplatelet drugs (16.4%) or valve replacement with aspirin treatment (7.3%; Table 12). Among the 708 participants who were excluded from further analysis because of incomplete data, the proportion undergoing CABG with aspirin treatment was lower than in the analysis population (31.7%; SMD 0.18) and the proportion undergoing valve replacement with aspirin treatment was higher than in the analysis population (10.3%; SMD 0.11). Otherwise, the baseline characteristics of the groups were similar, indicating that the analysis population was representative of the entire study population (see Table 12).
TABLE 12
The baseline characteristics of the analysis population and the population of participants who were consented but who were excluded from the COPTIC B analysis because of incomplete baseline characteristics or near-patient test result data
Clinical outcomes
The primary outcome of any CCB was recorded in 686 out of 2197 (31%) of the COPTIC A study cohort (Figure 10). The largest single group included those receiving non-routine pro-haemostatic treatments (n = 622; 28%). Drain losses of > 600 ml at 6 hours occurred in 334 participants (15%) and reoperations for bleeding occurred in 119 participants (4%). Postoperative CCB occurred in 449 out of 1833 (24%) of the COPTIC B study cohort (Figure 11). The proportion of participants receiving non-routine pro-haemostatic treatments was less (20%) than the proportion receiving non-routine pro-haemostatic treatments using the any CCB definition, whereas the proportions with excessive drain loss (14%) and reoperation for bleeding (3%) were similar. This was expected as the difference in the any CCB and postoperative CCB definitions was the intraoperative administration of pro-haemostatic treatments.

FIGURE 10
Primary outcome in the COPTIC A study (preoperative samples): any CCB (686/2197; 31.0%) (participants with missing data for one component of the primary outcome are not included in the Venn diagram).

FIGURE 11
Primary outcome in the COPTIC B study (postoperative samples): postoperative CCB (449/1833; 24.5%) (participants with missing data for one component of the primary outcome are not included in the Venn diagram).
Of the 535 (29.2%) participants with any CCB in the COPTIC B analysis population, 449 (24.5% of the analysis population) were recorded as having postoperative CCB (Table 13). Eighty-six participants (4.7% of the analysis population) had CCB reported both during and after surgery. All participants in the analysis population with recorded CCB during surgery also had recorded CCB after surgery. The frequency of the secondary outcomes is also reported in Table 13. Overall, the red cell transfusion rate was 32% in the COPTIC B analysis population, with 3.3% of participants requiring > 4 units in intensive care. The mortality rate as well as the rates of major morbidities were typical of reported event rates for a UK cardiac surgery population, with the exception of AKI, which occurred in 45% of participants.
TABLE 13
Prevalence of the primary and secondary outcomes in the COPTIC B analysis population
Baseline characteristics and clinical concern about bleeding
The frequencies of expected baseline risk factors in participants with any CCB in the COPTIC A cohort and participants with postoperative CCB in the COPTIC B cohort are shown in Tables 14 and 15 respectively. No formal comparison of these values in the CCB and non-CCB groups was performed.
TABLE 14
Preoperative analysis data set: baseline characteristics and CCB in the COPTIC A cohort
TABLE 15
Postoperative analysis data set: baseline characteristics and CCB in the cohort B analysis population
Point-of-care tests and clinical concern about bleeding
The results of the preoperative MEA test results and the postoperative MEA, ROTEM and TEG results are shown subdivided according to analysis population and occurrence of CCB in Table 16.
TABLE 16
Point-of-care test results and CCB in the COPTIC A and COPTIC B analysis populations
Results of specific analysis procedures
Step 1: modelling for baseline characteristics
We developed a clinical prediction model for any CCB in the COPTIC A analysis population and a predictive model for postoperative CCB in the COPTIC B analysis population using regression analyses. The baseline characteristics of male sex, absence of diabetes, procedure and antiplatelet treatment group, low preoperative haematocrit, low preoperative platelet count and low BMI explained most of the variation in any CCB and postoperative CCB (Tables 17 and 18). There were a number of similarities between the models: any CCB and postoperative CCB were less evident among female, diabetic and elective patients. Increasing the preoperative haematocrit and increasing BMI were associated with reduced odds of CCB. A reduced preoperative platelet count was also associated with reduced odds of CCB. The odds ratios (ORs) for preoperative platelet count are not comparable between the preoperative analysis and the postoperative analysis as these data were transformed for the any CCB model. In terms of the procedure and treatment categories, compared with the CABG with no treatment group, the participants with the highest odds of CCB were those undergoing CABG and valve replacement on DAPT, although this was a very small group (n = 22 in the preoperative analysis; n = 14 in the postoperative analysis). Increased odds of CCB in relation to the CABG with no treatment group were also observed for CABG and valve replacement patients on aspirin, CABG-only patients on DAPT with withdrawal at 0–2 days before surgery and patients undergoing high-risk other procedures, for any CCB only. Participating in another study also appeared to be related to the odds of CCB; in particular, those participants also enrolled in the TITRe2 RCT4 had increased odds of CCB.
TABLE 17
Expected relationships and findings: univariate analyses
TABLE 18
Baseline characteristics and CCB: multivariable models
Predictive models incorporating the baseline characteristics generated AUCs of 0.74 (95% CI 0.72 to 0.76) for any CCB (COPTIC A population) and 0.72 (95% CI 0.69 to 0.75) for postoperative CCB (COPTIC B population). The Hosmer–Lemeshow test demonstrated a satisfactory model fit (p = 0.28 and p = 0.59 for the COPTIC A and B baseline models respectively) and the Brier statistics were close to zero (0.18 for the COPTIC A model and 0.16 for the COPTIC B model). Bootstrapping the estimates of the baseline characteristics-only model gave AUCs of 0.74 (95% CI 0.72 to 0.76) for the COPTIC A population and 0.72 (95% CI 0.69 to 0.75) for the COPTIC B population and internal cross-validation gave AUCs of 0.74 (95% CI 0.72 to 0.76) for the COPTIC A population and 0.72 (95% CI 0.69 to 0.75) for the COPTIC B population. This model correctly classified 76.8% of participants in the COPTIC B analysis population as having CCB or having no CCB. The adapted baseline characteristics model for predicting any CCB in the COPTIC study, allowing for differences between the COPTIC population and the test data set, as described in Clinical prediction model, had an AUC of 0.70 (95% CI 0.67 to 0.73). The predictive model was externally validated in a cohort of 1611 participant recruited to the TITRe2 trial at 16 UK cardiac surgery centres (excluding Bristol) between July 2009 and February 2013.4 The adapted model is reported in Table 19. The AUC for any CCB in the TITRe2 cohort was 0.64 (95% CI 0.60 to 0.67).
TABLE 19
Adapted COPTIC study clinical risk prediction model used for external validation in the TITRe2 data set
Step 2: modelling for test predictors
Sequential addition of postoperative MEA, postoperative ROTEM and postoperative TEG test results to the baseline characteristics model caused a progressive increase in the AUC for prediction of postoperative CCB.
Model 2A: predictive accuracy of preoperative Multiplate (multiple electrode aggregometry) values for any clinical concern about bleeding
Of the preoperative MEA test predictors, only the ASPItest AUC was significantly associated with any CCB after taking the baseline characteristics into account; increasing the ASPItest AUC was associated with reduced odds of CCB (Table 20). The difference between the ROC curves illustrated in Figure 12 was not significant (0.74 vs. 0.74; p = 0.274), which is clearly demonstrated by the overlapping curves for the baseline model and the baseline model plus Multiplate preoperative measurements. To examine early test results, ASPI velocity was included as a potential determinant of CCB along with the ASPItest AUC. The selection process identified the ASPItest AUC as having a stronger effect. After bootstrapping the estimates, the model had an AUC of 0.74 (95% CI 0.72 to 0.76); cross-validation resulted in an AUC of 0.74 (95% CI 0.72 to 0.76). The model had an adequate fit (Hosmer–Lemeshow p-value = 0.28; Brier score = 0.18).
TABLE 20
Preoperative Multiplate (MEA) measurements in relation to any CCB (model 2A)

FIGURE 12
Preoperative Multiplate (MEA) measurements in relation to any CCB (model 2A).
Model 2B: predictive accuracy of preoperative Multiplate (multiple electrode aggregometry) values for postoperative clinical concern about bleeding
Only the preoperative ASPItest AUC was significantly associated with postoperative CCB after taking the baseline characteristics into account (Table 21). Increasing the preoperative ASPItest AUC was associated with reduced odds of CCB. The difference between the ROC curves was not significant (0.72 vs. 0.72; p = 0.3603; Figure 13). To examine early test results, the ASPI velocity and ASPItest AUC were both included as potential determinants of CCB. The selection process identified the ASPI velocity as having a stronger effect (ROC 0.72); however, this was still not significantly better than the clinical risk model (Table 22).
TABLE 21
Preoperative Multiplate (MEA) measurements in relation to postoperative CCB (model 2B)

FIGURE 13
Preoperative Multiplate (MEA) measurements in relation to postoperative CCB (model 2B).
TABLE 22
Preoperative Multiplate (MEA) measurements in relation to postoperative CCB (model 2B) using early test results
Model 2C: predictive accuracy of postoperative Multiplate (multiple electrode aggregometry) values for postoperative clinical concern about bleeding (COPTIC B study)
The natural log of the postoperative ADPtest AUC and the postoperative ADRENtest AUC were significantly associated with postoperative CCB after taking the baseline characteristics into account (Table 23); increasing the log of the postoperative ADPtest AUC was associated with reduced odds of CCB and increasing the postoperative ADRENtest AUC was associated with increased odds of CCB. The difference between the ROC curves was statistically significant (best baseline characteristics plus test 0.74 vs. baseline characteristics-only model 0.72; p = 0.001; Figure 14). To examine early test results, ADPtest velocity and ADRENtest velocity were both included as potential determinants of CCB alongside the ADPtest AUC and the ADRENtest AUC. The selection process identified the model detailed in Table 23 as the best model (ROC 0.74).
TABLE 23
Postoperative Multiplate (MEA) measurements in relation to postoperative CCB (model 2C)

FIGURE 14
Postoperative Multiplate (MEA) measurements in relation to postoperative CCB (model 2C).
Model 2D: predictive accuracy of postoperative rotational thromboelastometry for postoperative clinical concern about bleeding
Of the postoperative ROTEM predictors, only INTEM MCF was significantly associated with CCB after taking baseline characteristics into account. ROTEM FIBTEM MCF was dropped from the model because of colinearity. Increasing the INTEM MCF was associated with reduced odds of CCB (OR 0.95, 95% CI 0.94 to 0.97; Table 24). The difference between the ROC curves was statistically significant (best baseline characteristics plus test model 0.73 vs. baseline characteristics-only model 0.72; p = 0.0148; Figure 15). To examine early test results, INTEM A5 and INTEM A10 (measurements 5 and 10 minutes after starting the analysis) were both included as potential determinants of CCB alongside INTEM MCF. The selection process identified the standard tests model, that is, the model with results available at test completion, as the best model (ROC 0.73).
TABLE 24
Postoperative ROTEM measurements in relation to postoperative CCB (model 2D)

FIGURE 15
Postoperative ROTEM measurements in relation to postoperative CCB (model 2D).
Model 2E: predictive accuracy of postoperative thromboelastography for postoperative clinical concern about bleeding
Of the postoperative TEG values, celite kaolin (CK) α-angle and CK maximum amplitude (MA) were both significantly associated with CCB after taking baseline characteristics into account; increasing the CK α-angle was associated with increased odds of CCB (OR 1.02, 95% CI 1.00 to 1.04) and increasing the CK MA was associated with reduced odds of CCB (OR 0.93, 95% CI 0.91 to 0.96; Table 25). The difference between the ROC curves was statistically significant (best baseline characteristics plus test model 0.74 vs. baseline characteristics-only model 0.72; p = 0.006; Figure 16). To examine early test results, CK A30 (amplitude 30 minutes after clotting time) was included as a potential determinant of CCB alongside the CK α-angle and CK MA. The selection process selected CK A30 as a stronger predictor than the combination of CK α-angle and CK MA (see Table 25). The ROC for this model was 0.73, higher than that for the baseline model (0.72; p = 0.0167). However, the ROC for the initial model that did not use rapid rest results (Table 26) was marginally higher (0.74) and so may be considered preferable.

FIGURE 16
Postoperative TEG measurements in relation to postoperative CCB (model 2E).
TABLE 25
Postoperative TEG measurements in relation to postoperative CCB (model 2E)
TABLE 26
Postoperative TEG measurements in relation to postoperative CCB (model 2E) using early test results
Step 3: modelling for the predictive accuracy of combinations of postoperative thromboelastography/rotational thromboelastometry and Multiplate parameters
Model 2C (postoperative Multiplate parameters) and model 2E (TEG parameters) appear to demonstrate marginally better prediction of CCB than model 2D (ROTEM parameters). Step 3 considered whether the combination of postoperative measurements from the viscoelastic and Multiplate platforms could improve the model further.
All measurements from each of these models were considered for inclusion in the model. The test results for CK α-angle, CK MA, CK R – celite kaolin heparinase (CKH) R (TEG parameters) and ADRENtest AUC (Multiplate) were centred; the ADPtest AUC was log-transformed and centred. The final model from this stage is shown in Figure 17 and Table 27. The difference between the ROC curves was statistically significant (best baseline characteristics plus test model 0.75 vs. baseline characteristics-only model 0.72; p < 0.001). To test whether the addition of the postoperative Multiplate measurements significantly improved the model based on TEG parameters alone, the ROC curve for the TEG model was compared with the ROC curve for the model based on TEG and postoperative Multiplate parameters (0.75 vs. 0.74; p = 0.003). Additionally, the inclusion of the early-phase alternative parameters demonstrated that TEG CK A30 was a marginally inferior predictor than TEG CK MA, with a ROC curve of 0.75. After bootstrapping the estimates, the model had an AUC of 0.74 (95% CI 0.72 to 0.77); cross-validation resulted in an AUC of 0.74 (95% CI 0.72 to 0.77). The model had an adequate fit (Hosmer–Lemeshow p-value = 0.15; Brier score = 0.16).

FIGURE 17
Postoperative TEG and postoperative Multiplate measurements in relation to postoperative CCB (model 3).
TABLE 27
Postoperative TEG and postoperative Multiplate measurements in relation to postoperative CCB (model 3)
As the ROC curve for the model based on TEG parameters (model 2E) was only marginally better than that for the model based on ROTEM parameters (model 2D), this approach was repeated for the ROTEM parameters, that is, the postoperative Multiplate parameters were also considered for inclusion in the model (Figure 18 and Table 28). Increasing the ROTEM INTEM α-angle and log-transformed ADPtest AUC were associated with reduced odds of CCB. Increasing the ADRENtest AUC was associated with increased odds of CCB. The ROC curve for the model based on the ROTEM and postoperative Multiplate parameters was significantly better than that for the baseline model (0.74 vs. 0.72; p < 0.001). The difference in ROC curves between the ROTEM model and the model with both ROTEM and postoperative Multiplate parameters was significant (0.74 vs. 0.73; p = 0.01), suggesting that the addition of the postoperative Multiplate parameters improved the predictive ability of the model. Inclusion of the early-phase tests as alternatives to the standard test results did not change this final model.

FIGURE 18
Postoperative ROTEM and postoperative Multiplate measurements in relation to postoperative CCB (model 3).
TABLE 28
Postoperative ROTEM and postoperative Multiplate measurements in relation to postoperative CCB (model 3)
Step 4: modelling for preoperative and postoperative test predictors
Model 3 with the TEG and postoperative Multiplate parameters appears to be marginally better than the model based on TEG parameters alone. Step 4 considered whether the inclusion of the preoperative Multiplate measurements could improve this model further. No terms were selected and so the model reported in step 3 remains the best-performing predictive model. The increase in AUC attributable to the inclusion of the postoperative TEG and Multiplate results corresponded to an increase in the correct classification of only 0.98% of participants compared with the baseline model. From the baseline model to the best-performing predictive model, 63 participants were correctly reclassified as having CCB and 45 were incorrectly reclassified, a net improvement of 18 participants (NRI = 0.078; p < 0.001).
Sensitivity analyses of point-of-care test results
We used sensitivity analyses to address uncertainty around the definition of our reference outcome. As stated previously, all components of the CCB definition have limitations. The use of an early pro-haemostatic treatment may overestimate the frequency of coagulopathic bleeding, whereas outcomes such as drain losses or reoperation for bleeding may underestimate the frequency of coagulopathic haemorrhage. We therefore tested the predictive accuracy of the models developed in the base-case analysis using revised definitions of the primary outcome. We repeated the processes for model 1 and models 2A–E, with new models fitted for each sensitivity analysis. The parameters for each model were reselected for each step. The AUCs for these sensitivity analyses are reported in Table 29. The revised CCB definitions were as follows:
- In SA1, those defined as having received early pro-haemostatic treatment but who did not experience excessive bleeding (chest drain < 600 ml at 6 hours post operation) or require reoperation for bleeding were excluded from the analyses. This changed the frequency of the primary outcome as follows: any CCB, 351 out of 1862 (18.9%) participants; postoperative CCB, 268 out of 1652 (16.2%) participants. This revised definition resulted in only marginal improvements in predictive accuracy relative to the base-case analysis (see Table 29). Furthermore, it resulted in a reduction in the best-performing model (model 3).
- In SA2, those defined as having received early pro-haemostatic treatment but who did not experience excessive bleeding (chest drain < 600 ml at 6 hours post operation) or require reoperation for bleeding were included in the analyses but were reclassified as being free from CCB. This changed the frequency of the primary outcome as follows: any CCB, 351 out of 2197 (16.0%) participants; postoperative CCB, 268 out of 1833 (14.6%) participants. The discrimination of all of the models was markedly reduced using this definition (see Table 29).
- In SA3, after reviewing the results of our base-case analysis and the sensitivity analyses, we considered whether a definition of ‘more severe’ CCB would improve discrimination. We hypothesised that any pro-haemostatic therapy beyond the ‘first line’ treatment of bleeding, which in the Bristol Heart Institute consists of 1 unit of pooled platelets and 2 units of FFP, would denote more severe coagulopathic haemorrhage. We also hypothesised that a definition used in previous analyses of > 4 units of red cells transfused within 12 hours of surgery would also denote severe coagulopathy. Ultimately, this latter criterion was not included in the definition. Of the 13 COPTIC participants who received > 4 units of red cells in this period, nine were already classified as having CCB. Three were classified as not having CCB because their reoperation was defined as having a surgical cause. Consequently, one additional participant would now be classified as having CCB; this participant did not have a chest drain loss of > 600 ml at 6 hours, did not have a reoperation for bleeding and did not receive any products other than the > 4 units of red cells. This revised definition of CCB resulted in the following estimates of CCB: any CCB, 396 out of 1832 (21.6%) participants; postoperative CCB, 349 out of 1832 (19.1%) participants. Using this revised definition in a post hoc analysis did not improve the discrimination of any of the models and marginally reduced the discrimination of the best-performing model (model 3; see Table 29).
TABLE 29
Sensitivity analyses: AUCs (95% CIs)
Secondary outcomes
The preoperative Multiplate results and the postoperative Multiplate, ROTEM and TEG results are shown according to the analysis population and occurrence of the secondary outcomes in Appendix 1 (see Tables 103–109). Inclusion of near-patient test results improved the model prediction for any postoperative red cell transfusion (AUC 0.85, 95% CI 0.83 to 0.87 for the baseline model plus postoperative TEG results vs. AUC 0.83, 95% CI 0.81 to 0.85 for the baseline model alone; p < 0.001) and sepsis (AUC 0.74, 95% CI 0.70 to 0.79 for baseline model plus postoperative ROTEM results vs. AUC 0.70, 95% CI 0.65 to 0.75 for the baseline model alone; p = 0.01; Table 30). Inclusion of near-patient test results did not increase the predictive value of the baseline model for the other secondary outcomes (see Table 30).
TABLE 30
Area under the ROC curves for the predictive models for the secondary outcomes
Analysis of the predictive accuracy of laboratory reference tests
Overview
Two questions were considered in this analysis:
- LRT analysis 1 – can the predictive accuracy of a risk model for any CCB derived from baseline clinical factors be improved by the addition of data from automated cell counters used on blood samples obtained preoperatively? This analysis tested the hypothesis that measures of the differential platelet count, immature platelet count and MPV, along with other data obtained from automated cell counters preoperatively, such as the haematocrit, may be used to predict CCB, allowing earlier and more effective intervention.
- LRT analysis 2 – can the predictive accuracy of a risk model for postoperative CCB derived from baseline clinical factors be improved by the addition of all pre- and postoperative test results from automated cell counters and specific LRTs of coagulation using blood samples obtained at the end of surgery? This analysis tested the hypothesis that the use of pre- and postoperative data from automated cell counters as well as postoperative measures of specific components of the coagulation pathway, or pathway activity, may be used to more accurately predict those patients likely to bleed and allow targeted prevention strategies.
Study and analysis cohorts
As with the analyses of the POC tests, the final analysis data set was defined as those with complete baseline characteristics data, primary outcome data and at least one of the reference tests considered [PT, aPTT, D-dimer, fibrinogen, anti-Xa, vWF, ETP, factor XIII, platelet count and haematocrit]. As all reference tests were considered together and not as a comparison across platforms, any participant who had at least one test result was included in the analysis data set.
Table 31 describes the population included in the analysis data set (n = 2226) and those who consented but who were excluded (n = 315) (Figure 19). The mean age of the analysis population was 67 years. The relative proportions of the groups categorised by antiplatelet treatment or by type of surgical procedure were similar to those described in the COPTIC B analysis. There were some differences between the analysis population and the population excluded from the analysis (see Table 31). The proportion of males was lower in the excluded group (68.9% vs. 75.8%; SMD 0.16) and the median age was younger among those excluded (median 67.7 vs. 69.0 years; SMD 0.18). The proportion of patients undergoing valve replacement surgery and receiving aspirin was higher in the excluded group than in the analysis data set (14.0% vs. 7.5%; SMD 0.24). These differences were considered small. There were no other differences between the analysed population and the excluded population and we concluded that the two groups were similar.
TABLE 31
Laboratory reference test analyses: current analysis data set vs. consented participants not in the analysis data set

FIGURE 19
Flow chart for the COPTIC study: LRTs. a, Defined as eligible: surgery, not weekend, not emergency, not bank holiday, not Easter/Christmas study closure dates, aged ≥ 18 years.
Clinical outcomes
The primary outcome of any CCB was recorded in 684 out of 2200 (31.1%) of the reference test cohort for the analysis of preoperative automated cell counter data (LRT analysis 1) (Figure 20).

FIGURE 20
Overlap between components of the primary outcome of any CCB in the reference test analysis population (LRT analysis 1) (participants with missing data for one component of the primary outcome are not included in the Venn diagram).
The primary outcome of postoperative CCB was recorded in 572 out of 2226 (25.7%) of the reference test cohort for the analysis of postoperative automated cell counter data and LRTs of coagulation (LRT analysis 2) (Figure 21).

FIGURE 21
Overlap between components of the primary outcome of postoperative CCB in the reference test analysis population (LRT analysis 2) (participants with missing data for one component of the primary outcome are not included in the Venn diagram).
The proportions of the different components of the primary outcomes were as for the COPTIC B analysis population, as were the secondary outcomes (see Figures 20 and 21 and Table 32).
TABLE 32
Prevalence of primary and secondary outcomes in the LRT analysis population
Baseline characteristics and clinical concern about bleeding
The frequencies of expected baseline risk factors in participants who did or did not develop any CCB (LRT analysis 1) are provided in Table 33. The frequencies of expected baseline risk factors in participants who did or did not develop postoperative CCB (LRT analysis 2) are provided in Table 34.
TABLE 33
Baseline characteristics and any CCB in the LRT analysis population (LRT analysis 1)
TABLE 34
Baseline characteristics and postoperative CCB in the LRT analysis population (LRT analysis 2)
Reference test results and clinical concern about bleeding
Reference test results in participants with and without any CCB (LRT analysis 1) or postoperative CCB (LRT analysis 2) are summarised in Table 35. No formal comparison of these values was performed.
TABLE 35
Standard LRT predictors and CCB in the LRT analysis population
Results of specific analyses of laboratory-based test predictors
Laboratory reference test analysis 1 results
As with the analyses of the early tests, the predictive models were built by first considering the contributions to the prediction of any CCB of the baseline characteristics. The best model for all reference tests considered, after adjusting for baseline characteristics, was then found using a stepwise approach. Non-linearity of the reference test results was considered. Preoperative haematocrit and MPV were all associated with any CCB. Lower haematocrit levels and lower platelet count × MPV increased the likelihood of CCB (Table 36). The final best model developed with these predictors is shown in Figure 22. The AUC for the baseline characteristics model was 0.72 (95% CI 0.70 to 0.74) and for the full model was 0.74 (95% CI 0.71 to 0.76). This improvement in the AUC was small but statistically significant (p = 0.001). The percentage of participants correctly classified in the final model was 74.32% and in the baseline characteristics model was 72.60%.
TABLE 36
Laboratory reference test analysis 1 final model including LRTs: outcome any CCB

FIGURE 22
Predictive accuracy of LRTs for any CCB (LRT analysis 1). AUC baseline = 0.72, AUC baseline plus laboratory tests = 0.74 (p = 0.001).
Laboratory reference test analysis 1 sensitivity analyses
As in the analyses in the COPTIC A and COPTIC B studies, we conducted sensitivity analyses to address uncertainty around the reference outcome, CCB. Using the definition of CCB in SA1, we identified 348 out of 1864 (18.7%) participants in the LRT analysis 1 population who developed any CCB. The coefficients for the reference test predictors from the final model are shown in Table 36. Only the preoperative haematocrit and platelet count × MPV terms were predictors in this model. The AUC for the baseline model was 0.71 (95% CI 0.68 to 0.74) and for the full model including baseline characteristics plus the LRT results was 0.73 (95% CI 0.70 to 0.76). This difference was statistically significant (p = 0.004). ROC curves are shown in Figure 23.

FIGURE 23
Laboratory reference tests in relation to any CCB (LRT analysis 1, SA1).
For the second sensitivity analysis we used the definition of CCB as described in SA2. This identified 334 out of 2200 (15.2%) participants in the LRT analysis 1 population as having any CCB. In this analysis, preoperative haematocrit and platelet count × MPV remained significant predictors of CCB. The AUC for the baseline model was 0.68 (95% CI 0.65 to 0.71) and for the full model including baseline characteristics plus LRT results was 0.6946 (95% CI 0.66 to 0.73). This difference was statistically significant (p = 0.02). ROC curves are shown in Figure 24.

FIGURE 24
Laboratory reference tests in relation to any CCB (LRT analysis 1, SA2).
Laboratory reference test analysis 2 results
The baseline and full models were developed as previously described. Fibrinogen concentration, D-dimer concentration, haematocrit and factor XIII concentration were all associated with postoperative CCB. Lower preoperative haematocrit, lower postoperative fibrinogen levels and lower factor XIII levels increased the likelihood of CCB. Higher D-dimer levels increased the likelihood of CCB (Table 37). The final best model developed with these predictors is shown in Figure 25. The AUC for the baseline characteristics model was 0.6976 (95% CI 0.67 to 0.72) and for the full model was 0.73 (95% CI 0.71 to 0.76). This improvement in the AUC was small but statistically significant (p < 0.001). The percentage of participants correctly classified in the final model was 76.91% and in the baseline characteristics model was 76.28%.
TABLE 37
Laboratory reference test analysis 2 final model including LRTs: outcome postoperative CCB

FIGURE 25
Predictive accuracy of LRTs for postoperative CCB (LRT analysis 2). AUC baseline = 0.70, AUC baseline plus laboratory tests = 0.73 (p < 0.001).
Laboratory reference test analysis 2 sensitivity analyses
We conducted sensitivity analyses to address uncertainty around the reference outcome, postoperative CCB. Using the definition of CCB in SA1 we identified 354 out of 2008 (17.6%) participants in the LRT analysis 2 population who developed postoperative CCB. Only the preoperative haematocrit, fibrinogen level and D-dimer concentration were predictors in this model. The AUC for the baseline model was 0.69 (95% CI 0.66 to 0.73) and for the full model including baseline characteristics plus LRTs was 0.7437 (95% CI 0.71 to 0.77). This difference was statistically significant (p-value < 0.001) ROC curves are shown in Figure 26.

FIGURE 26
Laboratory reference tests in relation to postoperative CCB (LRT analysis 2, SA1).
For the second sensitivity analysis we used the definition of CCB as described in SA2. This identified 354 out of 2226 (15.9%) participants in the LRT analysis 2 population as having CCB. In this analysis the preoperative haematocrit and neutrophil count and postoperative fibrinogen level and D-dimer concentration remained significant predictors for postoperative CCB. The AUC for the baseline model was 0.67 (95% CI 0.64 to 0.71) and for the full model including baseline characteristics plus the LRT results was 0.73 (95% CI 0.69 to 0.76). This difference was statistically significant (p < 0.001). ROC curves are shown in Figure 27.

FIGURE 27
Laboratory reference tests in relation to postoperative CCB (LRT analysis 2, SA2).
Summary of the main findings of the COPTIC predictive accuracy studies
COPTIC A study
We conducted a prospective observational cohort study to examine the predictive accuracy of the addition to standard care of routine preoperative platelet function testing using the Multiplate device. We enrolled a large representative consecutive sample of all adult patients undergoing cardiac surgery at a single UK cardiac centre. Complete data were available for all demographic, baseline, clinical, laboratory and Multiplate parameters for 2197 (91%) participants, of whom 686 (31%) were suspected of having CCB. There was no evidence that the participants excluded from the analysis population had been selected in a non-random manner. We developed a clinical risk prediction model based on baseline factors that had good predictive accuracy for bleeding. We found that participants receiving DAPT were at increased risk of bleeding and this risk was greatest in those participants undergoing CABG or CABG and valve replacement procedures and taking DAPT within 2 days of surgery. After introduction to the model of the preoperative Multiplate results as predictors, only the ASPItest was significantly associated with bleeding; however, the addition of this value to the predictive accuracy model did not significantly improve discrimination. The early ASPItest result (velocity) showed a stronger association with bleeding than the ASPItest AUC value; however, the addition of this to the predictive accuracy model also failed to improve discrimination. On the basis of these results we conclude that preoperative Multiplate testing has low or no predictive accuracy for post-cardiac surgery coagulopathic bleeding beyond that achieved using clinical risk factors alone.
COPTIC B study
In this study the analysis population included 1833 participants, with complete data available for all demographic, baseline, clinical, laboratory and TEG, ROTEM and Multiplate parameters for 71% of participants. Of these, 535 (29%) developed any CCB and 449 (24%) developed postoperative CCB. The clinical prediction models for CCB showed good discrimination, with similarities in the principal clinical determinants of bleeding, as observed in the COPTIC A model. The addition of preoperative Multiplate test predictors did not improve discrimination for any CCB. However, the addition of postoperative test predictors for the Multiplate, ROTEM or TEG resulted in a progressive increase in predictive accuracy for postoperative CCB. The best-performing model for predicting bleeding was the combination of TEG and postoperative Multiplate parameters (AUC 0.75, 95% CI 0.72 to 0.77). Addition of preoperative Multiplate parameters did not show additional benefit. Sensitivity analyses that tested uncertainty around the definition of the reference outcome CCB by excluding components of the outcome that would either over- or underestimate the frequency of coagulopathic bleeding did not improve predictive accuracy. On the basis of these results we conclude that the routine use of postoperative TEG and Multiplate tests alongside a clinical risk prediction score would have greater discrimination than is observed with the clinical score alone. The magnitude of the difference was small, however, and the clinical importance was uncertain.
Laboratory reference test analyses
The analysis population included 2226 participants. For LRT analysis 1, 684 out of 2200 (31.1%) participants were classified as having any CCB. For LRT analysis 2, 572 out of 2226 (25.7%) participants were classified as having postoperative CCB. LRT analysis 1 considered whether the use of data from automated cell counters not otherwise used in standard care may be used to improve the predictive accuracy of baseline (preoperative) clinical prediction models. This demonstrated that the preoperative haematocrit as well as the product of the platelet count and MPV were predictors for bleeding. The inclusion of these variables in the predictive model showed greater discrimination for any CCB than the clinical risk prediction score alone; however, the magnitude of the difference appeared small. In LRT analysis 2, when pre- and postoperative automated cell counter results were combined with LRTs of coagulation, preoperative haematocrit and postoperative fibrinogen, D-dimer and factor XIII activity were found to be predictors for postoperative CCB. As with the other analyses the effect of adding these laboratory test results to the clinical prediction model was small. Sensitivity analyses confirmed that the definition of CCB was not the main explanation for this relatively poor ability of these tests to improve the prediction of CCB.
Health economic analysis
Aim of the cost-effectiveness analysis
The previous section described the clinical analyses of pre- and postoperative POC testing in cardiac surgery patients. The results showed that the addition of a combination of postoperative TEG and Multiplate test values to a clinical risk prediction model offered the greatest predictive accuracy for the prediction of postoperative bleeding. However, the magnitude of the difference between the final model and the clinical risk model alone was small and of uncertain clinical significance. This section considers whether introducing POC tests routinely into clinical practice would be cost-effective in a UK NHS setting. Good practice guidelines on the conduct of economic evaluations were followed101,102 and the analysis is described in accordance with the Consolidated Health Economic Evaluation Reporting Standards (CHEERS) statement.103
Our original intention was to use the clinical analyses to identify the most effective test (or combination of tests) and to perform a cost-effectiveness analysis of the test(s). As the preoperative Multiplate test showed little ability to predict CCB, we have focused on the postoperative tests and postoperative CCB. However, as the predictive accuracy results were similar for the different postoperative tests, we have evaluated the cost-effectiveness of all of the tests. As the Multiplate test indicates the need for platelets only, rather than indicating the need for a range of blood products, it was deemed most clinically plausible to consider its introduction into routine practice in combination with either TEG or ROTEM, rather than on its own. The test options evaluated were therefore TEG and ROTEM used separately and each in combination with the Multiplate test.
For each of these four test options the aim was to evaluate the routine use of POC testing in all patients. Although current practice did not include the routine use of these tests (at the time of the study), the care of some participants may have been guided by the ad hoc use of a POC test; specifically, the TEG test was in clinical use at the Bristol Heart Institute during the study. The comparison was therefore between current practice (which may have included the ad hoc use of a TEG test) and routine postoperative POC testing in all patients undergoing cardiac surgery. The research question was therefore whether or not it is it cost-effective to introduce routine postoperative POC testing in all patients undergoing cardiac surgery.
The predictive accuracy analyses evaluated the performance of the POC tests in predicting CCB, rather than evaluating how they would be used in practice and their effect on treatments given to patients. To evaluate the cost-effectiveness of the tests, it is necessary to consider how they would impact on a patient’s care pathway, rather than on an intermediary outcome such as predicting CCB, and how they would be used in practice: what test result cut-offs would indicate a positive test result and what treatments this could result in. In the cost-effectiveness analyses, POC tests were considered to ‘rule in’ treatments for patients but not to ‘rule out’ treatments. If a POC test result was positive (or an ad hoc TEG test was positive), this was assumed to lead to prophylactic treatment with protamine, platelets, FFP and/or cryoprecipitate. If a test result was negative this was assumed to have no impact on a patient’s care pathway.
Although introducing a test for all patients adds a cost to everyone in the short term, the hypothesis was that this would provide better treatment guidance and could reduce overall costs in the longer term. The aim of routine testing is to prevent severe blood loss, reopening of the chest for bleeding, large-volume transfusion, further pro-haemostatic treatments (second-line FFP, platelets, cryoprecipitate or rFVIIa) and shock and major morbidity (MI, AKI, stroke or sepsis) by prophylactic treatment.104 Patients with a positive test result would receive timely and appropriate prophylactic treatment to prevent the development of CCB and reduce the frequency of adverse events attributable to coagulopathic bleeding. This reduction in adverse events could lead to a reduction in total health-care costs and improve outcomes.
Economic decision model methods
To address the question of whether it is cost-effective to introduce routine postoperative POC testing in all patients undergoing cardiac surgery, an economic decision model was built to simulate the costs and effects of introducing a POC test for all patients compared with current practice. As uncertainty surrounds the performance of the POC tests in practice, a flexible framework was required to facilitate the assessment of uncertainty around parameter estimates. Models provide a simplification of the real world and will never capture every last possible scenario associated with an intervention, but the key consequences need to be included when models are conceptualised and structured.105 In this analysis the model was developed in consultation with clinical experts on the COPTIC study team. Figure 28 highlights the model scenarios. The initial model scenarios are based on the test results and are slightly different for current practice and postoperative POC testing for all. Thereafter, the model scenarios are the same for each arm of the model.
Cost-effectiveness model structure
The model combines the use of decision trees and Markov models and was developed in TreeAge Pro 2013 (TreeAge Software, Inc., Williamstown, MA, USA). All participants start in the ‘year 1’ health state of the Markov model and move through a decision tree, which is used to model the patient pathway after leaving the operating theatre up to 1 year post surgery. At the end of the decision tree, participants move to one of three further health states in the Markov model (alive having had no complications during the index hospital admission, alive having had complications in hospital and dead), which was then used to assess longer-term consequences on an annual basis. The model structure was split into two main arms (branches), with the top branch representing current practice and the bottom branch representing postoperative POC testing for all.
Current practice arm of the model to 1 year
Figure 29 shows the current practice arm of the model for the first year. The decision tree subdivides the starting cohort of participants undergoing cardiac surgery into two, depending on whether participants have an ad hoc TEG test or not. Some participants in the current practice arm had a TEG test and it was assumed that the results were acted on; it is therefore important to account for this in the current practice pathway.

FIGURE 29
Current practice arm of the model to 1 year. RBC, red cell units.
In the current practice arm, participants who have an ad hoc TEG test are then divided into those whose test is positive and those whose test is negative. The next branches in the tree divide participants into those whose POC test (whichever of the four options is being considered) is positive and those whose POC test is negative. Although this information is not used clinically in current practice, it has been incorporated within the structure of the current practice arm to facilitate the population of the model using the COPTIC study data. Participants with a positive ad hoc TEG result will receive first-line prophylactic treatment on the basis of the test result; this is likely to be protamine, platelets and/or FFP. This prophylactic treatment may be successful and CCB may be prevented or CCB may develop despite the prophylactic treatment. Participants with a negative ad hoc TEG test, and therefore no prophylactic treatment, may also go on to develop CCB or not. Participants are divided into those with and without CCB.
In the current practice arm, participants who do not have an ad hoc TEG test are divided into those whose POC test is positive and those whose POC test is negative. As for those with an ad hoc TEG test, this is for ease when populating the tree with COPTIC study data. The next branches in the tree divide these participants into those with CCB and those without CCB.
Regardless of whether participants have an ad hoc test or not (and whether this was positive or negative), the remaining branches in the tree are identical. After CCB or no CCB, participants are divided into those who receive treatment for clotting abnormalities or bleeding in the form of protamine, platelets or clotting factors in the first 12 hours after surgery, a reoperation for bleeding or > 4 units of red cells and those who do not. By definition, the only treatment that participants without CCB would receive is > 4 units of red cells. For participants with a negative ad hoc test or no ad hoc test, this includes any protamine, platelets or clotting factors received; for participants who receive prophylactic treatment, the protamine, platelets and/or clotting factors are in addition to those received as prophylaxis. As red cells may be given for reasons other than bleeding, for example 1 or 2 units of red cells may be given in response to a low haematocrit level or anaemia, it was considered more appropriate to regard large-volume transfusions (> 4 units of red cells) rather than any red cell transfusions as reliably reflecting clinically important bleeding,10 hence the criterion set here.
The remaining branches in the tree consider subsequent key events for participants: whether they develop any of four key complications in hospital (MI, stroke, sepsis or AKI), whether they survive to hospital discharge and whether they are alive at 1 year.
Postoperative point-of-care testing for all
The lower half of the model is shown in Figure 30 and illustrates the intervention arm and the routine use of a postoperative POC test for all participants. The structure of the model is the same regardless of whether the test introduced is TEG or ROTEM or either test in combination with the Multiplate test. The postoperative POC test is conducted and the cohort is split into two groups depending on whether the test result is positive or negative.

FIGURE 30
Postoperative POC test arm of the model to 1 year. RBC, red cell units.
Regardless of whether the POC test is positive or negative, participants are then divided into whether they had an ad hoc TEG test or not under current practice. Those with an ad hoc test are further divided into whether this is positive or negative. Participants are divided into these additional groups for the purposes of populating the model, as described in Model probabilities to 1 year: test-all arm.
If the POC test result is positive, then prophylactic treatment will be given to try to prevent the development of CCB. For participants with a positive POC test result, the following branches then indicate whether this prophylactic treatment is successful or not in preventing CCB. The following branches then indicate whether participants receive further pro-haemostatic treatments, > 4 units of red cells or a reopening for bleeding and, as in the current practice arm, whether they develop complications, their survival status at hospital discharge and 1 year and then the three health states in the Markov model, as described above.
If the POC test result is negative, this has no impact on a patient’s care pathway compared with current practice. The following branches indicate whether participants develop CCB or not and, as above, whether they receive further treatments or not, whether they develop complications and their survival status at hospital discharge and 1 year.
Both arms of the model beyond 1 year
Figure 31 shows the structure of the model beyond 1 year. In each arm, all participants start in ‘year 1’ of the Markov model and move through the decision tree, which models the participant pathway to 1 year, as shown in Figures 29 and 30. From 1 year, the structure of the model is the same in both arms. At 1 year, participants move into one of three health states in the Markov model: alive having had complications in their index hospital admission; alive having not had complications in their index hospital admission; and dead (see Figure 31). In each of the annual cycles thereafter, participants in the alive with complications health state can remain in that health state or die. Similarly, participants in the alive without complications health state can remain in that health state or die. The death state is an absorbing state (i.e. participants remain there). The analysis used a 5-year time horizon as it was anticipated that all major resource use (and outcomes) would occur within this time frame.

FIGURE 31
Model structure for both arms beyond 1 year.
Data for the model
The majority of the parameters in the model were populated with estimates derived from patient-level data from the COPTIC study conducted at Bristol Heart Institute as part of this programme of work, which incorporated the collection of key health economic variables. Analyses of these data (including the POC test results and resource use data) were conducted in Stata version 12. Of the 1833 participants included in the COPTIC B analysis population, 31 were excluded from the cost-effectiveness analyses. These 31 participants had a reoperation with a surgical cause of their bleeding; they were not classed as having CCB but, unlike other participants without CCB, they may have received non-red cell products in the first 12 hours after surgery or have had postoperative blood loss of > 600 ml at 6 hours collected in the chest drain, but these were assumed to be related to the surgical cause of bleeding. The cohort for the cost-effectiveness analyses therefore included 1802 participants. Participants had a mean age of 67 years and 76% were male. Most participants underwent CABG (59%) or valve replacement surgery (22%) and 25% of participants were classed as having postoperative CCB.
The following sections describe how the model transition probabilities, costs and outcomes were estimated to 1 year, largely based on the observational data. The final section describes how model inputs were estimated beyond 1 year. Tables 110–120 in Appendix 1 list the probabilities, costs and outcome estimates used to populate the base-case models. The analysis was conducted from a NHS and personal social services perspective,106 with costs expressed in 2013/14 UK pounds and outcomes as life-years. Costs and effects were not discounted in the first year and thereafter a discount rate of 3.5% per year was applied.107
Model probabilities to 1 year: current practice arm
The probabilities for each branch of the model to 1 year were generated using the patient-level data from the COPTIC study. Of the starting cohort of participants, 16% had an ad hoc TEG test (284/1802). The result of the ad hoc TEG test was not captured in the COPTIC study and so the results from the POC TEG test were used to determine the results. The TEG test produces a number of results, which have published normal ranges.108 In consultation with clinical experts on the study team, and based on 100% of the normal ranges, if any of the criteria in Box 4 was met a participant was considered to have a positive ad hoc TEG test result.
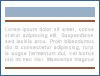
BOX 4
Criteria for a positive ad hoc TEG test result
Participants are then divided according to whether their POC test is positive or negative. Details of the criteria used to determine whether a POC test (or test combination) is considered positive or negative are provided in the following section (Model probabilities to 1 year: test-all arm); these criteria were also used to divide participants into POC test positive or negative in the current practice arm.
Participants were classed as having CCB or not based on the outcomes reported in the COPTIC study, with one adjustment for participants with a positive ad hoc TEG test. Any participant with a positive ad hoc TEG test and who received quantities of protamine, FFP or platelets that could be considered only first-line prophylactic treatment, and who had no other element of the CCB outcome, was reclassified from CCB to no CCB. The protamine, FFP or platelets given that caused the participant to be classified as CCB were considered in this case to be prophylactic and were deemed to have prevented CCB. It was not known what treatments were given to participants specifically as a result of the ad hoc test, but in-hospital treatment data were available. It was assumed that if participants received additional protamine only, or up to 1 unit of platelets and 2 units of FFP but no additional protamine in the first 12 hours after surgery, and no cryoprecipitate in the first 12 hours, and did not have CCB as a result of blood in the chest drain or a reoperation for bleeding, then they had had successful prophylactic treatment. In the base-case analysis, 16 participants were reclassified to ‘no CCB’ on this basis.
The subsequent probabilities of whether participants received treatment in the form of protamine, platelets, clotting factors (FFP, cryoprecipitate or rFVIIa), > 4 units of red cells and/or a reoperation for bleeding and whether they developed complications (MI, stroke, sepsis or AKI) were straightforward to obtain from the COPTIC study. Only complications occurring in the index hospital admission before discharge were captured; it is not known whether additional events occurred after discharge.
It was known from the Patient Analysis and Tracking System database whether participants were discharged from hospital or died in hospital and survival status at 1 year was available for all 1802 participants from the NHS Strategic Tracing Service (NSTS). The probabilities of being discharged from hospital and being alive at 1 year were therefore obtained from the COPTIC study. There were 73 deaths by 1 year, 31 in the index hospital admission and 42 between hospital discharge and 1 year.
Model probabilities to 1 year: test-all arm
Probability of a positive test result and treatments indicated by a positive test result
The TEG, ROTEM and Multiplate POC tests all produce a number of results, many of which have published normal ranges.108–111 In consultation with clinical experts on the study team, and informed by the normal ranges and a published algorithm for ROTEM,112 criteria were set to define positive test results and what treatments would be given as a result of positive tests. For TEG and ROTEM these values were approximately 80% of the normal ranges.
Thromboelastography base-case criteria for a positive test result
If any of the criteria in Box 5 was met a participant was considered to have a positive TEG test result in the base-case analysis. Criterion 1 was considered first and, if this indicated that a participant required protamine, it was assumed that he or she was given 50 mg of protamine and that this resolved the problem and was all that was needed. If the test did not suggest that a participant required protamine, then criteria 2–5 were considered and all treatments indicated were given.
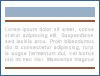
BOX 5
Base-case criteria for a positive TEG test result
The TEG results from the COPTIC study classified 3.6% (65/1802) of the cohort as having a positive test result. The criteria for determining positive ad hoc TEG test results and positive POC test results under a ‘test-all’ strategy were different. An ad hoc TEG test is likely to have been given to participants who clinicians were concerned about and clinical opinion in the study research team was that these test results would be interpreted less conservatively than routine tests for all participants, many of whom give clinicians no cause for concern.
Rotational thromboelastometry base-case criteria for a positive test result
Box 6 describes the criteria for a positive ROTEM test result in the base-case analysis, together with the treatments indicated. As for TEG, if protamine was indicated then it was assumed that no further treatment was required. If protamine was not required, then the other ROTEM criteria (2–5) were considered in their entirety and any treatments indicated were given.
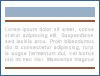
BOX 6
Base-case criteria for a positive ROTEM test result
Note that although TEG and ROTEM are being considered as alternatives, and EXTEM A10 is in some ways comparable to the α-angle or MA in TEG, ROTEM has the ability to discriminate between giving FFP and platelets and giving cryoprecipitate using the FIBTEM values; there is not an equivalent to this available in TEG. The ROTEM results from the COPTIC study classified 3.4% (61/1802) of the cohort as having a positive test result.
Thromboelastography/rotational thromboelastometry in combination with the Multiplate test base-case criteria for a positive test result
Box 7 describes the criteria for a positive Multiplate test result. If any of the criteria was met then platelets were indicated. In the event of a positive Multiplate test result, pooled platelets are transfused; however, the Multiplate test result was not considered on its own.
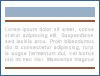
BOX 7
Base-case criteria for a positive Multiplate result
If any of the TEG/ROTEM or Multiplate criteria were met then the combined test result was considered positive. In terms of treatment, if the Multiplate test was introduced alongside TEG or ROTEM it was assumed that, if the TEG or ROTEM test indicated the need for protamine, then that was all that was required and the Multiplate test result was not used. If the TEG or ROTEM test did not indicate the need for protamine, then the Multiplate criteria were considered alongside the other TEG/ROTEM criteria.
If the Multiplate test indicated the need for platelets (even if TEG/ROTEM did not), platelets would be given alongside any other treatments indicated by TEG/ROTEM, as the Multiplate test would be considered more sensitive for platelet defects. If the reverse were true and TEG/ROTEM indicated the need for platelets but the Multiplate test did not, it was assumed that platelets were not given. This is really the added benefit of the Multiplate test in practice; if abnormal TEG/ROTEM parameters indicated the need for FFP and platelets but the Multiplate test result was normal, it was assumed that a participant would be given only FFP and that unnecessary treatment would be avoided.
Test results from the COPTIC study classified 5.0% (91/1802) of the cohort as having a positive test result according to the TEG and Multiplate tests combined and 4.9% (89/1802) as having a positive test result according to the ROTEM and Multiplate tests combined.
For participants who had a positive test result (from TEG or ROTEM or either TEG/ROTEM in combination with the Multiplate test) and who were given prophylactic treatment, an estimate of the probability that the prophylactic treatment was successful and that they would not develop CCB was needed. This is one of the key parameters in the model, but also a major unknown. Although it was not possible to observe this probability, the observational data were used to estimate it. The proportion of participants with CCB who received more than first-line treatment and hence for whom it was assumed that first-line treatment had failed was used as an estimate of the failure rate of prophylactic treatment. Primary treatment was assumed to have failed if a participant received > 1 unit of platelets or > 2 units of FFP, any cryoprecipitate, rFVIIa or a reoperation for bleeding. In total, according to this definition, treatment failed in 154 out of 449 (34.3%) participants with CCB. The base-case probability of prophylactic treatment failing was therefore 0.34 (with the complement of 0.66 signifying success). Alternative failure rates were explored in a sensitivity analysis.
Once participants had been classified according to whether their POC test was positive or negative based on the criteria described above, it was then straightforward to use the COPTIC study data to divide them into those who had an ad hoc TEG test and those who did not. For those who had an ad hoc TEG test, the POC TEG results were used to classify the ad hoc test as positive or negative, using the same criteria as in the current practice arm (described in Model probabilities to 1 year: current practice arm).
As the POC tests conducted during the observational study were not used to guide treatment, it is not possible to directly observe the impact of the test results on patients’ care pathways and on their treatments, costs and outcomes from this point forward in the model. However, at this point in the model, participants are classified into the same 12 groups based on their POC test result, whether they had an ad hoc test and its result (if applicable) as in the current practice arm. This was the reason for structuring the model by dividing participants in the current practice arm according to whether their POC test was positive or negative and dividing participants in the test-all arm according to whether they had an ad hoc test and its result. Participants in both arms of the model are therefore divided into the same 12 groups, but in a different order, as can be seen in Figure 32. In the figure, branches labelled with the same number in each arm include the same categories of participants. For example, participants in group 1 in both arms of the model had a positive ad hoc test and a positive POC test. The probabilities going forward from the branches numbered 1–12 in the test-all arm can then be considered to be as in the current practice arm, but with some adjustments, which are described next. Costs and life-years, which are described later, were also based on these 12 groups, again with some adjustments.

FIGURE 32
Current practice groups of participants used to populate the test-all arm: (a) current practice; and (b) test all.
Looking at the POC test-all arm, participants in groups 1 and 2, 5 and 6 and 9 and 10 all have a positive POC test. Under a test-all strategy, these participants would receive prophylactic treatment, with the aim of preventing CCB. Participants in groups 1 and 2 had a positive ad hoc test and it is therefore assumed that these participants had prophylactic treatment as a result of that test, which is already incorporated into the current practice arm. Therefore, for groups 1 and 2, no additional effects of the POC test are incorporated into the test-all arm.
Participants in groups 5 and 6 had a negative ad hoc test and participants in groups 9 and 10 had no ad hoc test and so none of these participants would have received prophylactic treatment as a result of the ad hoc test. The effect of the POC test therefore needs to be added here. The existing probabilities of CCB in groups 5 and 9 need to be multiplied by the probability that prophylactic treatment fails, thus increasing the probability that participants are in groups 6 and 10, rather than 5 and 9, that is, increasing the probability that they do not have CCB (compared with those groups under current practice).
In reality, there were no participants in group 6 in any of the base-case models and therefore it was not possible to switch participants from group 5 to group 6. However, there were fewer than four participants in group 5 in each of the models and so this will not have had a significant impact on the results. Participants in groups 5 and 6 had a negative ad hoc TEG test but a positive POC test; as less stringent criteria were used to determine whether the ad hoc TEG was positive than the more stringent criteria used to determine whether the POC test was positive, there are very few participants in these groups. In some of the sensitivity analyses of less stringent criteria for a positive POC test result it was possible to switch participants from group 5 to group 6. In the base-case models there were 15–20 participants in group 9 under current practice; these are the patients for whom the POC test could have a positive impact.
A limitation of not having observational data from which to estimate parameters in the test-all arm of the model is that it was not possible to ‘undo’ the effect of the ad hoc tests that are used in current practice. It was not possible to ‘take out’ the effect of the ad hoc TEG test in groups 1 and 2 and add back the effect of the POC test result; the assumption is that care would be the same under both scenarios (a reasonable assumption if the POC test is a TEG test like the ad hoc test, but less realistic if it is a different POC test). Additionally, for participants in groups 3 and 4 in the test-all arm who have a negative POC test but in reality who have had the effect of a positive ad hoc test incorporated into their care, it was not possible to take out the effect of any treatment as a result of the ad hoc test. There were between 95 and 107 participants in groups 3 and 4 in each of the base-case models to whom this applied.
Cost data to 1 year
In the current practice arm, the costs included were health-care costs to hospital discharge, health-care costs from hospital discharge to 1 year and the cost of the ad hoc TEG test (if applicable). In the test-all arm, the costs included were health-care costs to hospital discharge, health-care costs from hospital discharge to 1 year, the cost of the POC test and the cost of prophylactic treatment indicated by a positive test (for participants with positive results). Each of these cost categories are described next. The methods used for costing resource use for participants in the COPTIC study are summarised first.
Costs from the end of surgery to hospital discharge were estimated for each participant in the COPTIC study using the resource use data collected. Although a small number of data were collected on the study case report forms, the majority of the resource use data were obtained from two routine data sets: the Patient Analysis and Tracking Systems database and Innovian, the clinical information system that records hourly data for time spent in the cardiac intensive care unit (CICU). A considerable amount of additional work was undertaken by the team at Bristol to address known limitations of these data sets. For example, because of the number of missing data in the Patient Analysis and Tracking System for serious postoperative complications such as sepsis and AKI, Innovian data were also used to identify these complications, either by the event recorded in the Innovian database or by review of five free-text fields (see Figure 7). Data were available on the number of units of red cells, platelets, FFP and cryoprecipitate given during the CICU stay, any additional doses of protamine given after initial heparin reversal, the time spent in critical care and on a ward and the time of extubation, any complications and the use of rFVIIa. The time of extubation was used to differentiate between the time spent in critical care that should be considered as intensive care (CICU) and the time spent in a high-dependency unit (HDU). Complications captured and costed were stroke, MI, sepsis and AKI (a cost was attached to stage 3 AKI only). Any reoperations and use of an intra-aortic balloon pump (IABP) were also recorded and costed. The Patient Analysis and Tracking System database included variables for gastrointestinal and pulmonary complications, haemodynamic support and arrhythmias; however, > 60% of these data were missing. These complications were not secondary clinical outcomes and therefore additional work was not carried out to address these missing data. The decision was taken not to use these variables in the costings.
For the variables that were used, missing resource use data were assumed to be missing at random and were handled by applying multiple imputation using a series of chained regression equations.113 Twenty values were predicted for each missing data cell, essentially creating 20 different data sets. A method called Rubin’s rule (which acknowledges variability between as well as within imputed data sets) was used to summarise data across the 20 data sets.114 Overall, < 1% of resource use data were missing.
Table 38 summarises the observed resource use to hospital discharge for participants in the cohort. The 16 participants who had a positive ad hoc TEG test and who were deemed to have had successful prophylactic treatment are classified as having no CCB here. Tables 121 and 122 in Appendix 1 show the unit costs (and their sources) used to value this resource use; the resultant expected cost estimates for all participants and according to CCB status are shown in Table 39. The costs of any ad hoc TEG tests used are not included in these data as these costs were added into the model separately.
TABLE 38
Average resource use per participant in the COPTIC study
TABLE 39
Breakdown of the total average cost per participant in the COPTIC study
Populating the model with observed health-care costs to hospital discharge
Health-care costs to hospital discharge for each of the different pathways from the end of surgery to discharge could have been estimated by simply averaging the costs to hospital discharge for participants in that pathway. There are many individual pathways, however, and therefore a large number of cost estimates would have been required (in excess of 35), the majority of which would have been based on only a few participants (< 10). Cost estimates based on only a few participants may not be very accurate or reliable and could be heavily influenced by any outliers occurring by chance. Regression analysis was therefore used to pool participants whose costs were not statistically significantly different, across individual pathways, to increase the sample size for cost estimates; this also reduced the number of cost inputs required.
In Figure 32, 12 groups of participants were identified, based on their ad hoc test and POC test results, six with CCB and six without CCB. These 12 groups of participants were then divided into whether they received treatment in the form of protamine, platelets, clotting factors, > 4 units of red cells or a reoperation, whether they developed complications and whether they were alive at hospital discharge. For each of the eight different combinations of treatment/no treatment, complications/no complications and alive at discharge/died in hospital, regression was used to determine whether the costs to discharge were similar across some or all of the 12 groups of patients and for which groups costs could be pooled.
Initially, ordinary least squares (OLS) regression was conducted, with health-care costs as the dependent variable and dummy variables included for 11 of the 12 groups of participants (see Figure 32). Group 12, patients with no ad hoc test and a negative POC test, was taken as the base group as this was the largest group of patients. Any of groups 1–11 was considered suitable to be pooled with group 12 if its coefficient was not statistically significantly different from that for group 12. If several groups were statistically significantly different from group 12, Wald tests (which test the significance of particular explanatory variables, in this case dummy variables for groups) were conducted to determine whether these groups could be combined.
As the cost data were skewed, alternatives to OLS regression (and the normal distribution) were considered. A gamma distribution is positively skewed and often fits cost data well; a generalised linear model (GLM) with a gamma family and a log link was also conducted. As the results from the OLS and GLM models were not always identical, a modified Parks test was conducted for each regression to determine the most appropriate family115 and a Box-Cox test was performed to determine the most appropriate link function.116 Results from the GLM with the most appropriate family and link function were used to determine which groups could be combined. Generally, many groups could be combined and only one or two pooled cost estimates were required for each of the eight different combinations of treatment, complications and outcome at discharge, thus successfully increasing the sample size for each cost estimate. All cost estimates used in the models are reported in Tables 114–119 in Appendix 1.
Populating the model with observed health-care costs from hospital discharge to 1 year
As resource use data were not collected beyond hospital discharge in the COPTIC study, estimates for health-care costs beyond hospital discharge were sought from secondary sources. All participants who were discharged from hospital were assumed to incur health-care costs between hospital discharge and 1 year. Participants who had complications during their index admission were assumed to incur higher health-care costs during this time period.
Health-care costs from hospital discharge to 1 year were based on the Office of Health Economics estimate of the annual cost per person of NHS care,117 inflated to 2013/14 prices using the Hospital and Community Health Services inflation index,118 multiplied by the proportion of the first year that was not part of the index admission (based on the average LOS of participants alive at 1 year). All participants who survived to 1 year after their surgery were assumed to incur health-care costs of £2116 between hospital discharge and 1 year. Participants who died in the first year were assumed to incur health-care costs of £922 after hospital discharge, based on the same annual cost and the average proportion of 1 year for which they were alive and which was not part of the index admission.
For participants who had complications during their index admission, an additional cost was assigned between hospital discharge and 1 year. MI and stroke were assumed to be associated with continuing care costs. These annual costs were taken from Greenhalgh et al.119 and inflated to 2013/14 prices. Separate costs were provided for disabling and non-disabling stroke; according to Davies et al.,15 58% of strokes are disabling and this percentage was used to weight the two continuing care costs for stroke. An annual cost associated with complications was calculated by weighting estimates of the annual costs of MI and stroke by the number of participants alive at hospital discharge with these complications divided by the number of participants alive at discharge with any complications. Costs associated with complications between hospital discharge and 1 year were then calculated separately for participants alive at 1 year and for those who had died by 1 year, based on the proportion of the year for which they were alive and not in hospital for the index admission. Continuing care costs across all participants with complications were estimated to be £92 for participants alive at 1 year and £35 for participants who had died by 1 year.
Costs of the point-of-care and ad hoc thromboelastography tests
Pricing information was obtained from a published source for the TEG and ROTEM tests23 and from the manufacturer (Roche) for the Multiplate test. The price of capital equipment was converted into an equivalent annual cost to take account of depreciation and discounting, using a lifespan of 7 years for equipment and a 3.5% discount rate. It was assumed that, for any test introduced into routine practice at the Bristol Heart Institute, two analysers would be required. In terms of throughput for the analysers, information was taken from the National Adult Cardiac Surgery Audit (NACSA). According to this audit, 1449 cardiac surgery operations were conducted in 2012/13 at the Bristol Heart Institute.120 It was therefore assumed that each analyser would be used for 725 patients per year. Including both the capital and consumable elements, the cost of a TEG test per patient was estimated to be £37, the cost of a ROTEM test per patient was estimated to be £32 and the cost of a Multiplate test per patient was estimated to be £11.
The cost of an ad hoc TEG test under current practice was estimated to be higher than the cost of a TEG test if it were introduced for all patients. Although the consumable costs were the same as for the POC TEG test, the equipment costs were higher as the throughput for the analysers was lower. Two TEG analysers were in use at the time of the study and, as only 16% of participants received an ad hoc TEG test, each analyser was assumed to be used for 114 patients per year. The cost of the ad hoc TEG test under current practice was estimated to be £64.
Cost of prophylactic treatment indicated by positive point-of-care test results
When blood products were indicated, the following numbers of units were assumed to be given: platelets, 1 unit; FFP, 2 units; and cryoprecipitate, 2 units. A dose of protamine was assumed to be 50 mg.
For each participant in the COPTIC study classified as having a positive POC test result, the cost of the prophylactic treatment indicated by the test result was calculated. For example, 65 participants had a positive TEG test, of whom nine needed protamine, 29 FFP only and 27 platelets and FFP. The average cost [standard error (SE)] of prophylactic treatment for participants with a positive TEG test was £135 (£14). Similarly, the average cost (SE) of prophylactic treatment was £309 (£19) for participants with a positive ROTEM test, £96 (£8) for participants with a positive TEG/Multiplate test and £277 (£14) for participants with a positive ROTEM/Multiplate test.
Life-years to 1 year
Outcomes for the cost-effectiveness model were measured in life-years, as EuroQol-5 Dimensions (EQ-5D) data were not collected and therefore it was not possible to present the results using QALYs. Life-years to 1 year were calculated for each participant in the COPTIC study and participants alive at 1 year accrued 1 life-year in that time. For participants who died by 1 year, either in hospital or after discharge, the date of death was captured and therefore it was possible to calculate the number of days to death from the operation date and divide by 365 days to calculate the proportion of a life-year accrued. To populate the model, life-years were pooled in a similar way to costs to hospital discharge. OLS regression was used to determine whether there were any differences in the life-years accrued by the 12 groups of participants identified in Figure 32, for each combination of treatment/no treatment, complications/no complications and died in hospital/died after discharge but by 1 year. Frequently, life-year estimates could be pooled across all of the groups.
Populating the model beyond 1 year
No data were available from the COPTIC study beyond 1 year (follow-up was until hospital discharge or death and survival data were captured from the NSTS at 1 year) and so secondary sources of information were sought to populate the model. For each annual cycle beyond 1 year, estimates of survival and annual health-care costs were required.
Survival beyond 1 year
At 1 year, participants are split into three health states: alive having had no complications during the index hospital admission, alive having had complications in hospital and dead. In terms of survival, age- and sex-adjusted population life tables were used as the starting point,121 but these needed to be adjusted to account for the increased risk of death in participants who have had cardiac surgery compared with the general population. A further adjustment was required for participants who experienced complications, as these participants are known to be at an increased risk of death compared with participants without complications.
Adjusting for increased mortality after cardiac surgery
Data on the proportion of patients surviving each year after cardiac surgery from the NACSA were used to adjust for the increased risk of mortality after cardiac surgery compared with that in the general population.120 Using the separate survival curves for CABG, mitral valve surgery and aortic valve surgery available on the audit website, the proportion of patients surviving each year after surgery (up to 12 years) was read off the graphs. A weighted average of the proportion of patients surviving each year after surgery was calculated for all cardiac surgery, based on the proportions of the different types of surgery included in the audit.
To estimate the additional risk of mortality associated with having had cardiac surgery, the odds of dying each year after surgery were calculated for the NACSA population and for an age- and sex-matched population from life tables. The OR of dying in the cardiac surgery population compared with the general population was then calculated for each subsequent year after surgery. The OR was estimated to be 3.0 in the second year after surgery and it gradually reduced to 2.2 by 5 years after surgery (the OR for the first year after surgery was not required as survival to 1 year was available for participants in the observational study). These ORs were used to adjust the life table estimates for the increased risk of mortality after cardiac surgery for all participants in the model; life table probabilities were converted to odds to be adjusted and were then converted back to probabilities.
It is recognised that this will likely underestimate survival slightly for patients without complications, as the NACSA data include patients with and without complications. However, this underestimation will affect both the current practice arm of the model and the test-all arm and so should not affect the differences between treatment options.
Adjusting for increased mortality in the presence of complications after cardiac surgery
A second adjustment to survival was required for participants who experienced complications. Of the 777 participants alive at 1 year who experienced complications during their index admission, 749 had AKI (of whom 543 had stage 1, 113 had stage 2 and 93 had stage 3 AKI according to Acute Kidney Injury Network criteria for AKI122), 83 had sepsis, 17 had a stroke and 15 had a MI (these are not mutually exclusive events). As the data are dominated by AKI, rather than adjusting mortality for a number of different complications, mortality was adjusted for AKI only.
As individual patient data were available for survival to 1 year, it was important that estimates of increased mortality in participants with AKI (compared with those without AKI) from other sources did not include mortality in the first year after surgery as well as longer-term mortality, otherwise mortality in the first year would be double counted. In a review of long-term outcomes after AKI, Sawhney et al.123 identified two studies that compared mortality with and without AKI and looked at the timing of outcomes. Of the two studies reported, one was not specific to cardiac surgery124 but the other reported hazard ratios (HRs) for mortality at 12, 24, 36 and 60 months after cardiac surgery with and without AKI.125 HRs for group III (50–99% creatinine increase from baseline) in the study by Ishani et al.125 corresponded to participants with stage 1 AKI here. This slightly underestimated mortality for COPTIC participants with AKI, as they have a mix of stage 1–3 AKI. A sensitivity analysis was therefore conducted using HRs for group IV (> 100% creatinine increase from baseline) from the study by Ishani et al.125 HRs were used to adjust survival for participants who experienced complications (compared with those who did not); survival probabilities were converted to rates and adjusted using the HRs and were then converted back to probabilities.
Health-care costs beyond 1 year
Health-care costs beyond 1 year costs were calculated using the unit costs used to estimate health-care costs between hospital discharge and 1 year (described earlier). Annual health-care costs for participants alive in a subsequent year were estimated to be £2173; an additional annual cost of £99 was assigned to participants alive in a subsequent year who experienced a complication during their index admission.
Model analysis
For each model, once the structure had been set up in TreeAge and all probabilities, costs and outcomes entered for current practice, the expected costs and life-years associated with this arm were obtained for the first year, along with the probabilities of being in each of the three Markov states at the end of the first year. These costs, life-years and probabilities were calculated separately in Stata and therefore provided a robust check that all of the inputs had been entered correctly into TreeAge. Once the current practice arm of the model was set up, this was used to set up the test-all arm of the model. Separate calculations were conducted in Stata and Microsoft Excel® 2013 (Microsoft Corporation, Redmond, WA, USA) to estimate the differences between the strategies at 1 year, again as a check that all inputs in TreeAge were correct.
Costs and life-years beyond 1 year were discounted at a rate of 3.5%107 and a half-cycle correction was applied (which adjusts costs and deaths to occur half-way through each annual cycle, rather than at the beginning of the year).126 Cost-effectiveness analyses were run and expected costs and life-years associated with current practice and the test-all arm were calculated. The incremental cost-effectiveness ratio (ICER) was derived from the differences in costs and life-years between the test-all arm and current practice. The ICER is the cost per life-year gained of introducing a POC test compared with current practice. Analyses were conducted separately for TEG, ROTEM and each of TEG and ROTEM in combination with the Multiplate test.
Sensitivity analyses
Both one-way sensitivity analyses and probabilistic sensitivity analyses were conducted.
One-way sensitivity analyses
One-way sensitivity analyses were used to assess the sensitivity of the base-case results to key uncertainties in the model. Two key uncertainties were the probability of a positive POC test result and the probability that prophylactic treatment indicated by a positive test result was successful and prevented the development of CCB. Table 40 summarises the sensitivity analyses conducted.
TABLE 40
Sensitivity analyses around key parameters
The rationale for the sensitivity analyses is described in the following sections.
Probability of a positive test result (SA1)
For the POC tests there is uncertainty around the thresholds at which test parameters indicate a positive test and the need for prophylactic treatment. These thresholds still need to be validated. Less conservative thresholds for treatment were therefore considered in sensitivity analyses. The base-case thresholds used were approximately 80% of the normal ranges; 100% of the normal ranges were used to consider less conservative thresholds for treatment.
For TEG, the less conservative criteria in Box 8 were used to indicate positive test results (these are the same criteria used to identify positive ad hoc test results).
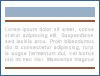
BOX 8
Criteria for a positive TEG test result used in sensitivity analyses
Based on these criteria, 32.8% (591/1802) of participants were classified as having a positive TEG test result and the average (SE) cost of their prophylactic treatment indicated was £222 (£3).
For ROTEM, the less conservative criteria in Box 9 were used to indicate positive test results.
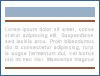
BOX 9
Criteria for a positive ROTEM test result used in sensitivity analyses
Based on these criteria, 10.6% (191/1802) of participants were classified as having a positive ROTEM test result and the average (SE) cost of their prophylactic treatment indicated was £262 (£12).
For the Multiplate test, the base-case criteria were increased by 25% to give the less conservative criteria in Box 10 for determining positive test results.
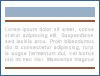
BOX 10
Criteria for a positive Multiplate test result used in sensitivity analyses
Based on these less conservative criteria, 34.1% (615/1802) of participants were classified as having a positive TEG/Multiplate test result and the average (SE) cost of their prophylactic treatment indicated was £67 (£2); 12.5% (225/1802) of participants were classified as having a positive ROTEM/Multiplate test result with an average (SE) cost of prophylactic treatment of £263 (£11).
Probability that the prophylactic treatment prevents the development of clinical concern about bleeding (SA2)
There is considerable uncertainty around the probability that the prophylactic treatment indicated by a positive test result is successful and prevents the development of CCB; this is a key parameter in the model. The base-case failure rate of 34.3% was varied by ± 25% in sensitivity analyses.
Probability of clinical concern about bleeding for participants in the test-all arm with a positive ad hoc thromboelastography test and a negative point-of-care test (SA4)
In the test-all arm it was not possible to ‘take out’ the effect of a positive ad hoc TEG test for participants who had a negative POC test. This was crudely attempted in a sensitivity analysis by shifting some of these participants from no CCB to CCB on the basis that they would not have received prophylactic treatment as a result of a positive ad hoc test and more of them may have developed CCB. Specifically, the probability of being in the no CCB group for participants with a positive ad hoc test but a negative POC test (group 4 in Figure 32) was multiplied by the probability that the prophylactic treatment failed.
To quantify the uncertainty around these one-way sensitivity analyses, parameters were assigned distributions (rather than fixed values) so that SEs and 95% CIs could be estimated; this was carried out in the same way as for the probabilistic sensitivity analyses that were conducted.
Probabilistic sensitivity analyses
As the majority of parameters in the model were estimated from the COPTIC study rather than the whole population of patients undergoing cardiac surgery, uncertainty exists around these estimates. Parameter uncertainty was investigated using probabilistic sensitivity analysis, which enabled the joint impact on the cost-effectiveness results of all of the uncertain parameters in the model to be assessed simultaneously.126–128 Rather than taking fixed values, model parameters were assigned probability distributions to describe the range of plausible values that they could take based on the precision of the estimates available. The type of distribution assigned was matched to the nature of the parameter, for example a beta distribution lies between 0 and 1 and is therefore appropriate for parameterising the probability of two mutually exclusive events and a gamma distribution is positive and continuous and was used to parameterise costs. The type of distribution assigned to each parameter is shown in Table 110 in Appendix 1.
Randomly selected values from each distribution for each model parameter were simultaneously generated using Monte Carlo simulation; this was repeated 1000 times and the results were calculated for each run of the model, generating a distribution of costs and effects that was used to calculate 95% CIs around the cost and life-year differences between strategies, enabling the effect of the parameter uncertainty on conclusions to be investigated. The results are presented on the cost-effectiveness plane and are also expressed in terms of a cost-effectiveness acceptability curve, which indicates the likelihood that a POC test is cost-effective for different amounts that health-care decision-makers are willing to pay for an additional life-year.102
Results of the economic evaluation
Base-case results
The expected costs and life-years gained under current practice and for each of the POC test options are shown in Table 41, together with the cost-effectiveness results for each POC test compared with current practice. For each of the POC test options, the MDs in costs and life-years compared with current practice are small and not statistically significant; the largest difference in costs is £33, approximately 0.2% of the total costs, and the largest difference in life-years is 0.0043, equivalent to 1.6 days.
TABLE 41
Base-case results from the cost-effectiveness model assessing the introduction of POC tests for all participants
Based on the point estimates of the ICER, TEG and TEG plus the Multiplate test are dominated by current practice as they are both more costly and less effective, but ROTEM and ROTEM plus the Multiplate test are likely to be considered cost-effective. However, there is great uncertainty around all of these results, as shown in plots of the 1000 simulated cost and life-year differences from the probabilistic sensitivity analysis for each POC test (Figure 33). In each figure a large number of points are in two or three quadrants of the cost-effectiveness plane. The black dot is the point estimate of the cost and life-year difference and in each figure is close to the origin.

FIGURE 33
Plots of 1000 simulated cost and life-year differences from the probabilistic sensitivity analysis for test all vs. current practice: (a) TEG; (b) ROTEM; (c) TEG and Multiplate; and (d) ROTEM and Multiplate.
The cost-effectiveness acceptability curves in Figure 34 show the probability that each POC test is cost-effective for a range of willingness-to-pay thresholds. If a decision-maker is willing to pay £20,000 for an additional life-year, then the probability of the test-all strategy being cost-effective is 0.38, 0.83, 0.32 and 0.74 for TEG, ROTEM, TEG plus the Multiplate test and ROTEM plus the Multiplate test respectively. Across willingness-to-pay thresholds from £0 to £100,000, TEG and TEG plus the Multiplate test are less likely to be cost-effective than current practice. As the willingness-to-pay threshold increases, ROTEM and ROTEM and Multiplate are more likely to be cost-effective than current practice. There is, however, much uncertainty around these findings. The dotted lines at 0.1 and 0.9 indicate the 80% confidence limits for the probability that a POC test is cost-effective. As these horizontal lines do not cut the curve at any point on any of the graphs, the 80% confidence limits on cost-effectiveness do not exist.

FIGURE 34
Cost-effectiveness acceptability curves for test all vs. current practice: (a) TEG; (b) ROTEM; (c) TEG and Multiplate; and (d) ROTEM and Multiplate.
Sensitivity analyses
The results of the sensitivity analyses are shown in Table 42. In three of the sensitivity analyses (SA1, 3 and 4) interpretation of the point estimates of cost-effectiveness was different from that in the base case for one or more of the POC tests. In all cases, however, differences in costs and life-years were very small and the uncertainty around the results remained.
TABLE 42
One-way sensitivity analysis results
Sensitivity analysis 1, which considered less stringent criteria for determining whether a POC test result was positive, had the greatest impact on the results for TEG and TEG plus the Multiplate test. Using the less stringent criteria, the point estimates suggest that these tests are more costly than current practice, as in the base case, but are now more effective; the ICERs are < £6000 per life-year, suggesting that the tests would be considered cost-effective. Neither the difference in costs nor the difference in life-years is statistically significant and the uncertainty around the results remains.
Under an extreme sensitivity analysis (SA3), assuming that prophylactic treatment is always successful, ROTEM became marginally less costly than current practice (by £2) and the point estimate of the ICER suggests that ROTEM dominates current practice, but there is huge uncertainty around this result, as demonstrated by the CIs around the cost and life-year differences compared with current practice.
When an attempt was made to ‘take out’ the effect of a positive ad hoc test result for participants in the test-all arm with a negative POC test result (SA4), the costs under each of the POC strategies increased, life-years decreased and ROTEM and ROTEM plus the Multiplate test were no longer likely to be considered cost-effective.
All other sensitivity analyses had very little impact on the results. In most cases there was little impact on the average costs and life-years in each group and therefore little impact on the differences between groups and the ICERs. Altering bed-day costs by ± 25% did alter average costs by approximately £1300, but this effect was seen under all strategies and therefore there was virtually no change in the MD in costs between the POC tests and current practice. Based on the point estimates, TEG and TEG plus the Multiplate test remain dominated by current practice, whereas ROTEM and ROTEM plus the Multiplate test are likely to be cost-effective (all ICERs are < £8000 per life-year except for SA4); however, as in the base case, differences are very small and the results are very uncertain.
Summary of the health economic analysis
There was very little difference between any of the POC tests and current practice in terms of either costs or life-years and there was great uncertainty around the cost-effectiveness results. Mean life-years to 5 years were 4.6 for all strategies considered; the greatest difference in life-years of 0.0043 is equivalent to 1.6 days. Costs were approximately £16,100 under each strategy and the largest MD compared with current practice was £33. Point estimates suggested that TEG and TEG plus the Multiplate test were more costly and less effective than current practice and therefore dominated by it and that ROTEM and ROTEM plus the Multiplate test were more costly and more effective than current practice; if a decision-maker was willing to pay £8000 for an additional life-year, then they would be considered cost-effective, according to the base case and all point estimates in the sensitivity analyses (except for the extreme case in SA4). However, given the small differences in costs and life-years, there was much uncertainty around these results. There was no clear difference in the cost-effectiveness of any of the POC test options compared with current practice.
Systematic review
Aims and objectives
Existing NICE guidance23 supports the routine use of POC tests for the management of bleeding patients in cardiac surgery. However, the results of the COPTIC study suggest that viscoelastic tests have limited or no benefits for the management of these patients. We have also identified limitations in the Health Technology Assessment (HTA) study26 that was used to inform the NICE guidance. Clinical uncertainty as to the utility of viscoelastic devices is reflected by variability in their use; the 2011 UK audit of blood transfusion in adult cardiac surgery concluded that these devices were not in common use in as many as 25% of units sampled.9 To address this uncertainty, and the limitations of the evidence used to support the current NICE guidance, we undertook a systematic review of RCTs assessing the clinical efficacy of viscoelastic tests in cardiac surgery. Unlike the HTA assessment,26 we included the results of a pragmatic multicentre trial that enrolled 7402 participants in 12 Canadian hospitals and which was published in 2016,129 we assessed important clinical outcomes including mortality, kidney injury, stroke and prolonged respiratory support and we summarised the evidence using the GRADE approach.
Methods
Search strategy and selection criteria
Search methods, data extraction, assessment and presentation were performed as recommended by the Cochrane Handbook for Systematic Reviews of Interventions (version 5.1.0).130 The analysis was specified in advance and registered on the PROSPERO international prospective register of systematic reviews (reference number CRD42016033831) on 31 January 2016. This review is reported in accordance with the Preferred Reporting Items for Systematic Reviews and Meta-Analyses (PRISMA) statement (see Appendix 1).131
Eligibility criteria
Randomised controlled trials, irrespective of blinding, language, publication status, date of publication and sample size, were considered eligible for this study. Participants of any age undergoing cardiac surgery for acquired or congenital disease or aortovascular disease, with or without CPB, were considered eligible. No age restriction was applied. There were no exclusion criteria.
Type of intervention
Eligible trials were those evaluating the risks and benefits of viscoelastic POC testing devices for coagulopathy [ROTEM, TEG or the Sonoclot® Coagulation & Platelet Function Analyzer (Sienco Inc., CO, USA)], alone or combined with platelet function testing.
Comparator
The comparator was represented by a combination of clinical judgement and/or SLTs including PT, aPTT, ACT and plasma fibrinogen levels. We did not distinguish between these comparators for the purpose of this review.
Types of outcome measures
The primary outcome was 30-day or hospital all-cause mortality. The secondary outcomes were adverse events including reoperation for bleeding, red cell, platelet and FFP transfusion, AKI, cerebrovascular accident (CVA), MI, ventilation time and ICU and hospital LOS.
Information sources
Potentially eligible trials were identified by searching the Cochrane Central Register of Controlled Trials (CENTRAL) (Internet), MEDLINE (PubMed, 1946 to present) and EMBASE (Ovid, 1975 to present) and the Cumulative Index to Nursing and Allied Health Literature (CINAHL) Plus (1979 to present) using a combination of subject headings and text words to identify relevant trials. The following MEDLINE search strategy was adapted as appropriate for other databases: ((Cardiopulmonary Bypass) OR (Cardiac Surgery) OR (Extra Corporeal Circulation) OR (Perioperative Morbidity) OR (Bleeding) OR (Coagulopathy) OR (Coagulopathic bleeding)) AND ((Thrombelastography) OR (Thromboelastometry) OR (Transfusion) OR (Viscoelastic testing devices)). The last search was run on 3 December 2016. In addition to searching databases we searched trial registries and checked reference lists of included studies.
Risk of bias assessment
The quality of the studies was assessed using the Cochrane Collaboration’s tool for assessing risk of bias.132 The items assessed were (1) sequence generation, (2) allocation concealment, (3) blinding of outcome assessor, (4) incomplete outcome data and (5) selective outcome reporting. The risk of bias was graded as unclear, high or low.
Study selection and data abstraction
Two authors (GJM, GFS) independently screened the search output to identify records of potentially eligible trials examining the outcomes, the full texts of which were retrieved and assessed for inclusion. Excluded studies and the reasons for exclusion were recorded.
A standardised form was used to extract data from the included studies for assessment of study quality and evidence synthesis. Extracted information included:
- year and language of publication
- country of participant recruitment
- year of conduct of the trial
- study setting: university teaching hospital, non-university teaching hospital
- study population and inclusion and exclusion criteria
- sample size
- participant demographics
- baseline characteristics
- type of surgery
- outcomes and times of measurement
- information for assessment of the risk of bias.
Data extraction forms were completed by one author (GFS) and checked by a second author (GJM). Similarly, quality assessment was carried out by one author (GFS) and checked by a second (GJM).
Assessment of reporting bias
When ≥ 10 studies were identified for each outcome, publication was assessed by the visual assessment of funnel plots and Egger’s test.133
Measures of treatment effect
For dichotomous variables we calculated the RR with 95% CI. For continuous variables such as hospital stay we calculated the MD with 95% CI; for quality of life (when different scales were used) we calculated the SMD with 95% CI.
Missing data
We performed an intention-to-treat analysis when possible. For dichotomous data presented only as percentages we estimated frequencies using reported sample sizes for these outcomes. For continuous outcomes, if the mean and the standard deviation (SD) were not available from the trial report, we sought this information from the trial authors. When this information was still not available, we calculated the mean and SD from the median and IQR using the software available in Review Manager version 5 (The Cochrane Collaboration, The Nordic Cochrane Centre, Copenhagen, Denmark).
Assessment of heterogeneity
We anticipated that major sources of clinical heterogeneity would be associated with different patient groups, the use of different goal-directed algorithms, the use of co-interventions such as restrictive transfusion thresholds and differences in the methodology used to assess coagulative dysfunction. We explored heterogeneity within each meta-analysis using a chi-squared test, with significance set at a p-value of 0.10, and we expressed the percentage of heterogeneity due to variation rather than chance as the I2 value.134 We defined heterogeneity as follows:
- I2 0–40%: no or mild heterogeneity
- I2 40–80%: moderate heterogeneity
- I2 > 80%: severe heterogeneity.
In the presence of severe heterogeneity, meta-analysis was not performed.
Data synthesis
Meta-analyses were performed using the software package Review Manager version 5.2 and in accordance with the recommendations of the Cochrane Handbook for Systematic Reviews of Intervention.130 For the primary analysis we compared the results of a random-effects model with those of a fixed-effects model to assess the effects of small studies. For continuous outcomes we pooled MDs or SMDs using the inverse variance method.
Subgroup analyses
Subgroup analyses were performed for different viscoelastic POC testing devices and by participant group: CABG compared with non-CABG. Tests for subgroup differences were carried out using Review Manager, with a p-value of < 0.05 considered statistically significant.
Sensitivity analyses
Sensitivity analyses were carried out excluding trials with a high risk of bias for random sequence generation, allocation concealment, blinding of participants, health-care providers or outcome assessors, incomplete outcome data or attrition, or with other sources of bias, including source of funding.
Summary of findings
The main results of the review are presented in a summary of findings table. We included the following outcomes:
- risk of mortality
- risk of reoperation and bleeding
- risk of red cell, FFP and platelet transfusion
- resource use: ICU and hospital LOS.
We used GRADEpro software to prepare the summary of findings table. We judged the overall quality of the evidence for each outcome as ‘high’, ‘moderate’, ‘low’ or ‘very low’ using the GRADE approach. We considered the following:
- impact of the risk of bias of individual trials
- precision of the pooled estimate
- inconsistency or heterogeneity (clinical, methodological and statistical)
- indirectness of the evidence
- impact of selective reporting and publication bias on the effect estimate.
Results
A total of 5125 abstracts were retrieved from the searches (Figure 35), of which 4570 articles were excluded as they were duplicate publications, were studies carried out in animals or were reviews and 533 articles were excluded on the basis of title and abstract. In total, therefore, 22 potentially relevant publications were retrieved for further assessment. Fifteen trials that randomised a total of 8737 participants met the inclusion criteria and were included in the analysis.112,129,135–147 Two of the included studies were published in abstract form.139,144 The two review authors (GJM, GFS) were in agreement on the selection of included studies. The key characteristics of the individual studies are described in Table 43.

FIGURE 35
Preferred Reporting Items for Systematic Reviews and Meta-Analyses (PRISMA) flow diagram.
TABLE 43
Characteristics of the included studies
Included studies
Of the 15 trials identified in our search, 13 enrolled adults undergoing CABG (n = 2),135,136 mixed cardiac surgery (n = 10),112,129,139,140,142–147 or surgery on the thoracic aorta (n = 1)138 and two enrolled children undergoing surgery for congenital disease.137,141 The sample size ranged from 22143 to 7402129 participants. The largest trial,129 which enrolled more participants (n = 7402) than all of the other trials, used a multicentre stepped-wedge cluster RCT design. To adjust for the stepped-wedge cluster design we recalculated the effective sample size for this trial as per the recommendations in the Cochrane Handbook for Systematic Reviews of Interventions130 using the intracluster coefficient calculation of 0.095 stated in the trial methods.129
All of the trials evaluated the efficacy of blood management algorithms based on viscoelastic test results. One trial140 evaluated the ROTEG device, seven trials evaluated ROTEM,112,129,138,139,141,143,144 and seven trials evaluated TEG.135–137,142,145–147 Blood management in control groups was at the clinicians’ discretion in combination with SLTs in eight trials,135,138,140,142,144–147 according to SLTs alone in six trials 112,129,136,139,141,143 and according to clinical judgement alone in one trial.137 Ten trials provided data on the length of follow-up,112,129,136,138,140,141,143,144,146,147 which ranged from 24 hours to 3 years.
Excluded studies
Seven trials that met our inclusion criteria were excluded after review of the full manuscript (Table 44). Four studies were excluded because of insufficient data to judge the study design.149–152 In the trial by Agarwal et al.,148 viscoelastic testing algorithms were applied in both the treatment group and the control group. Traverso et al.153 performed the randomisation in abdominal aortic aneurysm. Two trials (NCT00772239 and NCT01218074) were published only as protocols, with no data available.
TABLE 44
Characteristics of excluded studies
Risk of bias in included studies
The overall quality of the studies was evaluated based on the major sources of bias (domains), as described earlier. None of the parallel-group randomised trials could be classified as being at low risk of bias. The various bias domains are presented in Figure 36.

FIGURE 36
Risk of bias summary: (a) all studies; and (b) individual studies. +, low risk of bias; –, high risk of bias; ?, unclear risk of bias.
Risk of bias in the stepped-wedge cluster randomised controlled trial
The trial by Karkouti et al.129 was judged to be at low risk of bias for the domains of recruitment bias, baseline imbalance, loss of clusters and incorrect analysis.
Sequence generation
Random sequence generation was adequate in four trials112,138,141,146 and unclear in eight trials.135–137,139,140,143,144,147 Two trials had a high risk of bias for this domain.142,145
Allocation concealment
Allocation concealment was unclear in 13 trials.112,135–141,143–147 One trial had a high risk of bias for this domain.142
Blinding of participants and personnel
There was evidence of blinding of participants and clinical staff caring for participants in two trials,135,146 unclear evidence in five trials139–141,143,145 and evidence of lack of blinding in seven trials.112,136–138,142,144,147 There was evidence of blinding of outcome assessors in two trials,136,146 unclear evidence of blinding of outcome assessors in seven trials,135,139–141,143,145,147 and a high risk of detection bias in five trials.112,137,138,142,144
Incomplete outcome data
Nine trials reported completeness of follow-up for the primary outcome.112,135,136,138,141–143,146,147 All of them reported < 10% loss to follow-up. Three trials that failed to report completeness of follow-up were considered to be at high risk of attrition bias.137,144,145 Insufficient reporting of attrition/exclusion to permit a judgement was detected in two trials.139,140
Selective reporting
Three trials were published only in abstract form;139,143,144 in these trials some outcomes were not reported or treatment effects were not reported and thus they were considered to be at high risk of bias for selective reporting. All of the others trials but one129 were considered to be at unclear risk of bias because we were unable to retrieve the original protocols of the trials or evidence of publication in a trial registry.
Other potential sources of bias
Nine trials disclosed the funding source.112,129,136,137,140,141,143,144,146 Of these, three trials were at risk of funder bias.112,129,141 For the remaining studies, the funding source was defined as unknown. Sample size calculations were reported in eight trials.112,129,135,136,138,142,145,146
Effects of interventions
A summary of the findings for the main comparison between TEG or ROTEM and usual care for patients undergoing cardiac surgery is reported in Table 45.
TABLE 45
Summary of findings for all primary and secondary outcomes
Primary outcome
Hospital mortality was reported in seven trials.112,135,138,141,143,145,146 Mortality was lower in participants treated with TEG- or ROTEM-guided algorithms (12/350) than in participants receiving usual care (23/339); however, this was not statistically significant (RR 0.55, 95% CI 0.28 to 1.10; I2 = 1%, chi-squared test for heterogeneity p = 0.40, random effect) (Figure 37).

FIGURE 37
Mortality. df, degrees of freedom; M–H, Mantel–Haenszel.
Secondary outcomes
Bleeding and transfusion
Red cell transfusion
Eleven RCTs reported data on the number of participants transfused with allogenic red cells.112,129,135–138,140,141,143,146,147 Red cell transfusion was reduced in participants treated with TEG- or ROTEM-guided algorithms, with some heterogeneity (RR 0.88, 95% CI 0.79 to 0.97; I2 = 43%; chi-squared test for heterogeneity p = 0.06, random effect) (Figure 38). Exclusion of the trial by Karkouti et al.129 did not alter the summary effect estimate or the heterogeneity (RR 0.86, 95% CI 0.76 to 0.96; I2 = 52%; chi-squared test for heterogeneity p = 0.03, random effect).

FIGURE 38
Red cell transfusion. df, degrees of freedom; M–H, Mantel–Haenszel.
Fresh-frozen plasma transfusion
Eight RCTs reported data on the number of participants receiving FFP.112,129,135,136,138,141,143,146 The summary effect estimate for TEG- or ROTEM-guided algorithms compared with controls was a RR of 0.68 (95% CI 0.46 to 1; I2 = 79%; chi-squared test for heterogeneity p = 0.0001, random effect) (Figure 39). Exclusion of the trial by Karkouti et al.129 did not significantly alter the summary effect estimate or reduce the heterogeneity (RR 0.62, 95% CI 0.38 to 0.99; I2 = 84%; chi-squared test for heterogeneity p = 0.05, random effect).

FIGURE 39
Fresh-frozen plasma transfusion. df, degrees of freedom; M–H, Mantel–Haenszel.
Platelet transfusion
Ten RCTs reported data on the number of participants receiving platelet transfusions.112,129,135–138,140,141,143,146 The use of viscoelastic tests resulted in a significant reduction in the number of participants receiving platelet transfusions (RR 0.78, 95% CI 0.66 to 0.93; I2 = 0%; chi-squared test for heterogeneity p = 0.60, random effect) (Figure 40). Exclusion of the trial by Karkouti et al.129 did not alter these findings (RR 0.76, 95% CI 0.63 to 0.91; I2 = 0%; chi-squared test for heterogeneity p = 0.003, random effect).

FIGURE 40
Platelet transfusion. df, degrees of freedom; M–H, Mantel–Haenszel.
Reoperation (for bleeding)
Nine trials reported the number of participants who required reoperation for bleeding.112,135,136,138,139,142,143,145,146 The use of viscoelastic tests resulted in a reduction in the of number of reoperations for bleeding; however, this effect did not reach statistical significance (RR 0.82, 95% CI 0.55 to 1.23; I2 = 0%; chi-squared test for heterogeneity p = 0.63, random effect) (Figure 41).

FIGURE 41
Reoperation for bleeding. df, degrees of freedom; M–H, Mantel–Haenszel.
Morbidity
Acute kidney injury
Four trials reported the frequency of severe AKI.112,135,138,143 The use of viscoelastic tests reduced the frequency of severe AKI compared with usual care, with moderate heterogeneity (RR 0.42, 95% CI 0.20 to 0.86; I2 = 26%; chi-squared test for heterogeneity p = 0.02, random effect) (Figure 42).

FIGURE 42
Acute kidney injury. df, degrees of freedom; M–H, Mantel–Haenszel.
Cerebrovascular accident
Two trials138,146 evaluated the number of participants with CVA. There was no difference between the viscoelastic test group and the control group with respect to the frequency of stroke (RR 1.61, 95% CI 0.45 to 5.81; I2 = 0%; chi-squared test for heterogeneity p = 0.47, random effect) (Figure 43).

FIGURE 43
Cerebrovascular accident. df, degrees of freedom; M–H, Mantel–Haenszel.
Respiratory support
Three trials135,138,143 provided continuous data on the ventilation time in each group. Meta-analysis demonstrated a shorter ventilation time in the viscoelastic test group; however, this was not statistically significant (MD 0.28, 95% CI –0.66 to 1.23; I2 = 0%; chi-squared test for heterogeneity p = 0.56, random effect) (Figure 44).

FIGURE 44
Ventilation time (hours). df, degrees of freedom; IV, inverse variance.
Resource use
Intensive care unit length of stay
This outcome was reported in three studies.135,138,143 The ICU LOS was shorter in participants randomised to TEG- or ROTEM-guided algorithms than in those receiving usual care; however, this difference was not statistically significant (MD –31.76 hours, 95% CI –94.68 to 31.17 hours; I2 = 59%; chi-squared test for heterogeneity p = 0.32, random effect) (Figure 45).

FIGURE 45
Intensive care unit LOS (hours). df, degrees of freedom; IV, inverse variance; VE, viscoelastic test group.
Hospital length of stay
This outcome was reported in three studies.135,138,143 Hospital LOS was shorter in participants randomised to TEG- or ROTEM-guided algorithms than in those receiving usual care; however, this difference was not statistically significant and there was moderate heterogeneity (MD –3.11 days, 95% CI –9.57 to 3.34 days, I2 = 69%; chi-squared test for heterogeneity p = 0.34, random effect) (Figure 46).

FIGURE 46
Hospital LOS (days). df, degrees of freedom; IV, inverse variance; VE, viscoelastic test group.
Publication bias
For two outcomes (red cell transfusion and FFP transfusion) a sufficient number of trials provided data (≥ 10 studies identified for each outcome) for the evaluation of publication bias. The funnel plot of the SE compared with the RR for red cell transfusion showed an asymmetrical distribution and a regression test for the funnel plot asymmetry model [z = –2.21; p < 0.026 (random-effects version of Egger’s test)] that indicated likely publication bias (Figure 47a). The funnel plot of the SE compared with the RR for platelet transfusion showed a symmetrical distribution and a regression test for the funnel plot asymmetry model [z = 0.22; p = 0.818 (random-effects version of Egger’s test)] that indicated no publication bias (see Figure 47b). Exclusion of the trial by Karkouti et al.129 did not alter these findings (p = 0.015 for red cell transfusion and 0.724 for platelet transfusion).

FIGURE 47
Funnel plots: (a) red cell transfusion; and (b) platelet transfusion.
Sensitivity analyses
Sensitivity analysis was not conducted because none of the included trials was considered to be at low risk of bias.
Subgroup analyses
Results of the subgroup analyses for TEG algorithms compared with ROTEM algorithms, TEG algorithms compared with other algorithms and CABG compared with non-CABG are shown in Table 46.
TABLE 46
Subgroup analyses
GRADE summary
The results of the GRADE summary of the evidence are provided in Table 47. There was no difference between POC testing and usual care for mortality, red cell transfusion, bleeding and major morbidity, with the exception of severe AKI. The quality of the evidence was low or very low for all of the outcomes assessed, implying a high level of uncertainty with respect to these results.
TABLE 47
Summary of the main findings of the systematic review and GRADE assessment of the trial results
Discussion
Main findings
Predictive accuracy study
We recruited a large cohort of consecutive adult patients undergoing cardiac surgery, of whom approximately 30% developed CCB, a composite of objective criteria indicative of bleeding that is likely to lead to patient harm. We found that clinical risk factors were able to accurately predict CCB and that the clinical risk prediction model was dominated by the complexity of the operative procedure and treatment with P2Y12 receptor blockers close to the time of surgery. We demonstrated that the addition of preoperative platelet function tests to this model did not improve predictive accuracy. In contrast, postoperative POC tests, including the results of the Multiplate, TEG and ROTEM tests, improved predictive accuracy. The best predictive model was achieved by the combination of postoperative TEG and Multiplate values. Although statistically significant, the magnitude of the incremental increase in predictive accuracy was small and of uncertain clinical utility.
Health economic analysis
We evaluated the cost-effectiveness of the introduction of POC tests for coagulopathy into routine clinical care using decision-analytic modelling. For each of the POC test options considered, the MDs in costs and life-years compared with current practice were small and not statistically significant; the largest difference in costs was £33, approximately 0.2% of the total costs, and the largest difference in life-years was 0.0043, equivalent to 1.6 days. Based on the point estimates of the ICER, TEG and TEG/Multiplate test were dominated by current practice as they were both more costly and less effective. ROTEM and ROTEM/Multiplate test were more costly and more effective than current practice and would be considered cost-effective if a decision-maker was willing to pay £8000 for an additional life-year. However, there was great uncertainty around all of these results because of the very small differences in costs and life-years.
Systematic review
A systematic review of trials of viscoelastic POC tests in cardiac surgery identified 15 RCTs, of which none was judged to be free from potential bias. Pooled effect estimates from the trials showed that a TEG- or ROTEM-guided algorithm for the management of coagulopathic haemorrhage reduced the number of patients requiring transfusion, but had no effect on mortality, stroke, prolonged intubation and emergency reopening for bleeding or on ICU and hospital LOS. There was a significant reduction in the frequency of severe AKI in four trials in which this was reported. The GRADE assessment concluded that the quality of the evidence was low or very low for all of the outcomes.
Strengths and limitations
Predictive accuracy study
The COPTIC study has several important strengths. The study design reduced the likelihood of bias observed in previous studies that have considered the predictive accuracy of POC tests in cardiac surgery.25 It evaluated POC tests in an unselected consecutive cohort of patients in whom these devices are in common clinical use and recruited 70% of all eligible patients undergoing surgery at a single large cardiac centre over a 2.5-year period. Data on demographics, baseline clinical characteristics and laboratory and POC test results were missing in a proportion of participants; however, our analysis suggested that ‘missingness’ occurred at random and was unlikely to have introduced bias. The index tests were performed in a dedicated laboratory by trained staff, with careful quality controls and standard operating procedures in place to ensure data quality. The use of the tests was timed to coincide with their common use in the patient pathway and test predictors were evaluated that are used in clinical practice, increasing the likelihood that the results of our analyses are relevant to the clinical setting. The predictor tests were also conducted independently of the clinical setting and did not influence clinical care or the reference outcome. We used a reference outcome that was a composite of objective clinical measures of bleeding that on balance we considered to accurately reflect bleeding likely to cause harm. We tested uncertainty over the accuracy of this reference outcome in a series of sensitivity analyses by using alternative definitions of CCB that we considered would either underestimate or overestimate the frequency of severe bleeding or identify only very severe bleeding; however, this did not alter the results. A final strength is that the COPTIC study is the largest ever study to consider the predictive accuracy of POC tests in bleeding patients. To date, it recruited more participants than all of the previous predictive accuracy studies evaluating Multiplate or viscoelastic tests, including TEG and ROTEM, combined.
The limitations of the COPTIC study are important considerations when interpreting the results. First, the COPTIC study was a single-centre study and therefore our findings may not be generalisable to other centres. Our clinical risk prediction score, for example, had only moderate discrimination when externally validated in a multicentre cohort of participants from the TITRe2 trial.4 This is a limitation of almost all centre-specific risk prediction scores for bleeding (see Chapter 3). It also suggests that blood management in Bristol may differ from that in other centres. This was also suggested by the findings of the 2011 UK audit of blood transfusion in adult cardiac surgery,9 in which the Bristol Heart Institute had rates of red cell (44%, UK mean 53%), platelet (22%, UK mean 28%) and FFP (15%, UK mean 24%) transfusion and a reopening rate (4.6%, UK mean 4.9%) below the national averages. In the national transfusion audit,9 differences in red cell transfusion and reopening rates persisted after adjustment for baseline clinical characteristics (platelet and FFP transfusion were not analysed after risk adjustment). This implies that existing blood management protocols are applied effectively at the Bristol Heart Institute, which is supported by the use of ad hoc POC tests in 15% of participants in this study. This could reduce the likely cost-effectiveness of POC testing as an intervention. In contrast, the implementation of effective blood management protocols would also tend to increase the predictive accuracy of POC tests as the unnecessary use of non-routine pro-haemostatic treatments would be less frequent and the objective measures of CCB used in the study would be more likely to accurately represent coagulopathic bleeding.
Second, the number of missing data in the COPTIC B analysis is a limitation. We have shown that ‘missingness’ occurred at random; however, our analysis population included 1833 (72%) of the total cohort. This is a reflection of the complexity of the study and the limitations of the POC platforms, which can be unreliable and often provide incomplete panels of results. Because of the size and overall costs of the study we elected to perform the POC tests for each sample in singlicate. All results were then immediately reviewed by the laboratory technician. When the panel of results was incomplete the tests were repeated; however, reproducibility was limited by the maximum interval allowed between sampling and testing, which we specified as 120 minutes for ROTEM and TEG and 240 minutes for the Multiplate test. No previously published predictive accuracy study of POC tests has reported the degree of incomplete test results and we are therefore unable to compare the degree of missing data in this study with that found in other studies. This is less likely to limit the use of these devices in clinical practice because of the redundancy of multiple measures of similar processes (e.g. α-angle = K time = MA).
Third, there is no consensus definition of coagulopathic bleeding and the outcome measure of CCB was developed by the clinical team to capture the different ways in which bleeding might be apparent. The resulting definition, which effectively defined coagulopathic haemorrhage by its treatment, is imprecise and may lead to over- or underestimation of the disease prevalence. In other screening programmes, for example for breast cancer, a patient with a positive screen would have a biopsy taken and a definitive diagnosis would be obtained. Here, there is no gold standard test for coagulopathy. Moreover, coagulopathy manifests clinically as a heterogeneous condition, in which multiple defects in platelet function and coagulation may co-exist. In the COPTIC B study we attempted to address this complexity using a series of LRTs of coagulation factors and coagulation pathway function. Fibrinogen concentration and D-dimer levels were, as expected, predictors of coagulopathic haemorrhage; however, the effect size of these measures was modest, implying that other unmeasured factors may also be important. Until the pathogenesis of coagulopathy is better defined we must accept that the predictive accuracy of the POC tests is unclear.
Health economic analysis
Our health economic analysis had important strengths. This was the first cost-effectiveness analysis of POC tests in cardiac surgery for which the majority of the model inputs (probabilities, costs and life-years) were estimated directly from an observational cohort of patients, rather than from published secondary sources. In contrast, the health economic analyses of POC tests in cardiac surgery26 used to inform current NICE guidance23 were based on estimates of resource use and outcomes from published sources. The health economic analysis in the COPTIC study also benefited from the insights gained by the researchers in related economic evaluations undertaken for the TITRe24 and PASPORT154 trials. The cost-effectiveness analysis evaluated predictive test thresholds that are in current clinical use.112 The robustness of these thresholds was tested in a series of sensitivity analyses and the likelihood of cost-effectiveness was investigated in probabilistic sensitivity analyses.
There were also limitations of the health economic analysis. First, the limitations of the predictive accuracy study are also limitations of the cost-effectiveness analysis. Specifically, the definition of the reference outcome by its treatment prevented determination of whether transfusion of FFP or platelets was an effective intervention to prevent CCB or whether it occurred as a consequence of CCB. Sensitivity analysis in the predictive accuracy study used alternative definitions of CCB, including a definition in which only participants who received > 2 units of FFP, > 1 unit of platelets or > 2 units of cryoprecipitate were adjudged to meet the CCB criteria or definition in which CCB was defined solely by blood loss and emergency re-sternotomy. These alternative definitions did not materially alter our findings. It is therefore unlikely that definitions of more severe CCB would alter the results of the cost-effectiveness analysis. The lack of a gold standard definition of the primary outcome is also a limitation. Typically, the sensitivity and specificity of diagnostic tests would be incorporated into the decision model; however, as stated above, the true discrimination of these tests is unclear. In sensitivity analyses we modelled the effect of using different treatment thresholds for each of the POC tests evaluated; again, this did not materially alter our results.
Second, there were a number of participants in the COPTIC cohort who underwent an ad hoc TEG test and whose care pathway was assumed to have been influenced by a positive result. This may have biased the results in favour of the POC tests in the POC test arm of the model as it was not possible to ‘take out’ the effect of the ad hoc test; the tests were much less favourable in a sensitivity analysis that crudely attempted to take out the effect of a positive ad hoc TEG test. Although data on the ad hoc use of TEG in theatre and in the CICU were collected in the COPTIC study, the results of these TEG tests were not recorded and it is not known how or whether or not results were acted on. With hindsight it would have been helpful to capture further information here to more accurately reflect what happened to these participants.
Third, there were relatively few positive POC test results (compared with the number of participants with CCB in the COPTIC study) and therefore relatively few participants for whom the positive POC test could impact positively on their pathway (between 15 and 20 participants). Even if the POC tests had a significant impact for these few participants, it is perhaps not surprising that, across a cohort of 1802 participants, the differences in resulting costs and life-years between strategies were small. Recognising that there are only small differences between the strategies also explains why the majority of the sensitivity analyses had very little impact on the cost and life-year differences between the POC tests and current practice; to a very large extent the same changes were made to each strategy.
Fourth, ideally quality of life as well as quantity of life would have been considered and QALYs used as the outcome measure. QALYs can be compared across a broad range of treatments, interventions and clinical areas. However, it was not possible to collect information on quality of life as part of the observational study and so life-years were used as the outcome measure. It is therefore more difficult to draw conclusions about the value for money of the POC tests, particularly in comparison to other treatments and interventions in health care, although comparisons can be made with other studies that report life-years.
Fifth, if a POC test were introduced in practice, it is likely that any patients who continued to bleed after a positive test result and initial prophylactic treatment would be retested to identify whether or not further treatment was required. Retesting patients was not taken into account in this model.
Sixth, there were limitations around the complications data available for participants in the COPTIC study. Although Innovian data were used to supplement the Patient Analysis and Tracking System data for postoperative complications that were secondary clinical outcomes in the study to reduce the number of missing data, there were additional complications (such as pulmonary and gastrointestinal complications) that were not included as secondary outcomes in the clinical analyses but which ideally would have been costed. Data from the Patient Analysis and Tracking System for these complications were missing for > 60% of participants and data on these complications were deemed not useable, although we consider it likely that the impact of these complications will have been partially captured by other measures of resource use, for example ICU stay.155
Seventh, although information was available on survival status at 1 year, resource use was captured only until hospital discharge. Although secondary sources were used to make assumptions about resource use beyond hospital discharge, it would have been beneficial to have individual patient data on, for example, hospital readmissions or further health-care resource use beyond hospital discharge up to 1 year (and even beyond).
A final limitation is that our cost-effectiveness analysis was based on observational data. The cost-effectiveness of a clinical intervention is best estimated from measures of resource use and outcomes obtained from appropriately designed RCTs.
Systematic review
The principal limitation of the systematic review is that the findings and interpretations are constrained by the quality and quantity of the available evidence. We judged that all of the 14 parallel-group trials had significant limitations in terms of methodological quality. The risk of procedural bias was high in these trials as there was little or no allocation concealment or blinding of clinical personnel. The potential to produce summary effect estimates was limited by the wide variety of outcomes reported and a lack of standardisation of the way in which these were measured. The assessment of heterogeneity was limited by the relatively small number of studies that contributed to each meta-analysis. Our assessment of likely bias differed from that in a recent Cochrane review.156 For example, in the Cochrane review the use of sealed envelopes was considered to represent a low risk of bias for allocation concealment. However, Cochrane guidance132 states that only sealed opaque envelopes may be considered as evidence of allocation concealment. When the term opaque was omitted we therefore classified the risk of bias as unclear rather than low. Similarly, the Cochrane reviewers assessed several studies as having a low risk of reporting bias, despite the absence of prospective trial registration or the availability of trial protocols for reference.156 We judged that these trials should be categorised as having unclear evidence of reporting bias; hence, we found no trial to be at low risk of bias in our analysis. These limitations notwithstanding, the current meta-analysis provides the most comprehensive evaluation of viscoelastic POC tests in cardiac surgery to date. It considered all of the trials identified in two recent meta-analysis,26,156 along with the results of a recent large cluster randomised trial not included in these previous analyses.129 This trial by Karkouti et al.129 enrolled 7402 participants, almost five times the total number of participants enrolled in the previous RCTs identified in our search. We evaluated the effects of including/excluding this study from our primary analysis in terms of its influence on the effect estimates. This analysis demonstrated that the inclusion of this trial did not substantially alter the summary effect estimates for those outcomes for which data were available, specifically, that viscoelastic tests reduced the numbers of participants receiving red cell and platelet transfusions.
Clinical importance
Clinical risk prediction for clinical concern about bleeding
A principal finding of our study was that preoperative clinical risk assessment has good predictive accuracy for coagulopathic bleeding. Key preoperative risk factors included the use of P2Y12 receptor blockers shortly before surgery and planned longer complex procedures. Interestingly, a low preoperative haematocrit was found to be a risk factor for bleeding. This suggests a link between chronic inflammatory diseases, the main cause of preoperative anaemia in cardiac surgery,27 and bleeding that is not well recognised. This may be an area for future study. Clinical risk prediction scores are not widely used despite class I recommendations that they are included in standard care.24 This is because existing scores have been developed in small single-centre cohorts and, when they are externally validated, they are often found to lack discrimination or calibration. Scores developed in multiple centres in multiple countries are more likely to have generalisability. This is the subject of Chapter 3.
Point-of-care tests
The COPTIC study addressed uncertainty over the predictive accuracy of platelet function testing in cardiac surgery. We showed that preoperative Multiplate testing did not improve discrimination beyond clinical risk assessment. This contrasts with the recommendations of established blood management guidelines in cardiac surgery24 and a recent consensus statement from UK researchers recommending routine preoperative platelet function testing.92 Corredor et al.25 reviewed studies that had considered the predictive accuracy of preoperative platelet function testing in cardiac surgery. The evidence for preoperative testing was inconsistent and positive studies considered only patients receiving P2Y12 receptor blockers. In the largest of these studies, Ranucci et al.157 evaluated the predictive accuracy of preoperative Multiplate values for subsequent bleeding in 352 participants receiving DAPT within 7 days of surgery. They found that ADPtest AUC values of < 22 U had a negative predictive value for bleeding of 94% and a positive predictive value of 20%. The ROC AUC was 0.6. When combined with a TRAPtest cut-off of < 75 U, these tests identified seven of the 27 participants in the study who experienced severe bleeding.157 These thresholds were very low, however, as the normal range for the ADPtest AUC is 51–75 U. This study showed that preoperative platelet function testing had only moderate predictive accuracy, even in selected participants receiving P2Y12 receptor antagonists. Together with the results of the COPTIC study we conclude that clinical risk prediction alone, that is, knowing how recently P2Y12 receptor blockers have been stopped, in combination with other determinants of bleeding, can provide good predictive accuracy and that preoperative platelet function testing has no added benefit.
The COPTIC analyses identified only small incremental increases in predictive accuracy with the use of postoperative POC viscoelastic tests. The limitations of the COPTIC analyses notwithstanding, it is difficult to reconcile evidence of limited predictive accuracy with limited trial evidence of efficacy as shown by the systematic review and the widespread use of these devices in clinical care. In a recent RCT by Weber et al.,112 the use of an integrated ROTEM and Multiplate algorithm resulted in a reduction in mortality compared with a treatment algorithm based on SLTs. This study was noteworthy for several reasons. First, the POC-based management algorithms were applied to bleeding patients, thereby increasing their potential clinical effectiveness. This is different from the COPTIC analyses, which considered the routine use of POC devices in all patients, in whom their clinical effectiveness would be expected to be less. Second, the bleeding algorithm was complex and required clinical insight and knowledge of the limitations of the testing platforms. We modelled our health economic analysis algorithm for the ROTEM and Multiplate tests on the published Weber et al.112 algorithm, but this may not have adequately captured the clinical decision-making process in complex bleeding patients. Third, the Weber et al.112 study allowed repeat testing in bleeding patients; this was not a feature of the COPTIC analysis, although, for a significant number of patients in the COPTIC analyses, ad hoc TEG tests were used to manage active bleeding. The difficulties in evaluating the effects of these interventions in the COPTIC analyses have been described earlier (see Economic decision model methods). Fourth, the Weber et al.112 study enrolled only 100 participants and was stopped prematurely because of the difference in mortality between the groups, limiting the precision of the estimates in the trial. A final comment, however, is that the COPTIC health economic analysis, discussed in more detail below, identified ROTEM, as well as the combination of ROTEM and the Multiplate test, as being potentially cost-effective, although the effect was small and of uncertain significance. These points serve to highlight the uncertainty around the clinical effectiveness and cost-effectiveness of POC testing.
Given the evidence of poor predictive accuracy and limited clinical efficacy demonstrated in the COPTIC study and other recent analyses it is interesting to speculate why these devices remain in widespread use. The main reason is that there are no alternatives; there have been no significant developments in this technology for several decades. Moreover, if these devices are licensed for use and are supported by treatment guidelines there are few financial incentives for the development of new devices. This also reflects the limitations of our understanding of coagulopathy. The COPTIC trial enrolled 2542 participants and was the largest predictive accuracy study of cardiac surgery coagulopathy ever conducted. All previous predictive accuracy studies that have evaluated POC tests in cardiac surgery have enrolled a total of 1338 participants. As discussed earlier, many of these studies were at high risk of bias, but they have been used to support the widespread use of these devices, for example the preoperative use of platelet aggregometry, for which we have found no evidence. The scale of this research effort is also relatively modest; bleeding potentially affects every patient undergoing cardiac surgery, with > 1 million procedures performed worldwide per year. Research into the pathogenesis of coagulopathy and the development of new technology and diagnostic tests are clearly warranted.
Laboratory reference tests
We evaluated the predictive accuracy of a range of LRTs that may be used to diagnose common acquired coagulopathies. Although none of these tests is available currently as a POC test, evidence of utility would be a driver towards the development of such a test. Our findings confirmed that SLTs, PT and aPTT did not increase predictive accuracy beyond that of our clinical risk score. Reference tests for vWF, factor Xa activity and ETP also failed to improve discrimination. These results address conflicting evidence from previous small studies that have considered the interaction between these measures and bleeding,77,158,159 although all of these studies were at high risk of bias. A low recombinant factor XIII level has also been shown to be associated with coagulopathic bleeding in previous studies.160,161 This was associated with bleeding in some of our models but not others. A recent RCT has shown that recombinant factor XIII administration does not reduce bleeding or transfusion rates.162 A consistent finding in all studies is the association between low fibrinogen concentrations and bleeding. In a study in 4202 cardiac surgery patients, Karkouti et al.163 observed a fibrinogen threshold of 2 g/dl below which blood loss increased. Several small RCTs have also shown that fibrinogen administration reduces bleeding in cardiac surgery164,165 and a Cochrane review of cardiac and non-cardiac surgery trials reported a similar finding.87 No previous study has evaluated the predictive accuracy of low fibrinogen levels. In the COPTIC study we confirmed that this added to the discrimination of the model; however, the effect was small. FIBTEM values were also predictive; however, this was omitted from the ROTEM model because of collinearity with the MA value, which can also reflect fibrin deficiency. The dominance of low fibrinogen levels in these analyses is of interest as it provides an alternative explanation for the apparently conflicting results of the COPTIC study and NICE analyses23 in that tests that do not have predictive accuracy have clinical efficacy when evaluated in clinical trials. This explanation is that it is the treatment algorithm and not the diagnostic test that provides the clinical benefit. In the RCT by Weber et al.,112 in which a ROTEM/Multiplate-based algorithm resulted in reductions in bleeding, transfusion and mortality, the treatment algorithm was characterised by early fibrinogen administration. Similar clinical benefits were not observed in trials in which platelets were administered along with FFP as initial treatment.26 We suggest that low fibrinogen levels may contribute to bleeding in a significant proportion of patients and that this group may derive a benefit from prompt fibrinogen administration. Future work will consider whether a hierarchical clustering analysis of the COPTIC data may permit early identification of this group of patients.
Health economic analysis
To inform recent NICE guidelines23 a HTA study26 considered the introduction of POC tests in several clinical areas, including cardiac surgery. In the literature review of cost-effectiveness analyses of POC tests, only one study was identified, a model conducted for the Scottish NHS.93 This study was largely based on a previous decision tree used to assess methods for minimising perioperative allogeneic blood transfusion and which considered the relationship between transfusion and subsequent complications and adverse events.15 Whiting et al.26 also used a decision tree to assess the cost-effectiveness of POC tests in cardiac surgery, based on these previous studies but updating many of the parameter estimates with data from their clinical effectiveness review. Whiting et al.26 compared TEG and ROTEM with standard laboratory testing and their cost-effectiveness results indicated that POC testing was dominant; each POC test was less costly and more effective than standard laboratory testing.26 Their conclusions were robust to various sensitivity analyses conducted. Across a broad range of willingness-to-pay thresholds, the probability of the POC tests being cost-effective was approximately 0.80.
The results of the Whiting et al.26 analyses differ from the results of the COPTIC cost-effectiveness analyses. This may be explained by differences between the studies and by their limitations. Whiting et al.26 concluded that there was evidence of efficacy of POC test-based algorithms based on evidence from RCTs, which constitutes a higher level of evidence than the COPTIC study. The main outcome measure in the Whiting et al.26 study was the proportion of patients receiving a red cell transfusion under a POC testing strategy compared with standardised laboratory testing. However, the RCTs in the Whiting et al.26 analyses were considered to be at high risk of bias and we have assessed the quality of this evidence as being low or very low using the GRADE approach. Moreover, the reduction in red cell transfusion demonstrated by Whiting et al.26 is particularly susceptible to performance bias in unblinded trials. Furthermore, our own analysis has indicated that the reporting of this outcome is likely to be subject to publication bias. The interpretation of the economic analysis was further limited by the extrapolation of these results to calculated differences in clinical outcomes based on the estimated risks of transfusion obtained from older published observational studies94 that are now known to be attributable to bias.32 For example, the probabilities of adverse events attributed to red cell transfusion were based on an analysis that demonstrated that the HR of death for transfused compared with non-transfused patients was 6.69 (95% CI 3.66 to 15.1). This estimate was based on our earlier work.94 A more recent meta-analysis of RCTs has shown that more liberal transfusion strategies that increase the risk of transfusion (OR 1.7) also reduce the risk of death (HR 0.61) in patients with cardiovascular disease.32 However, all of the differences in clinical outcomes in the Whiting et al.26 analysis were based on the transfusion rates; patients were assumed not to have complications such as MI, stroke and renal dysfunction if they did not receive a transfusion. In contrast, in the COPTIC analysis, in which clinical outcomes were measured rather than estimated, we observed that in many cases COPTIC participants who did not have a red cell transfusion experienced complications. Furthermore, we observed no difference between POC testing and standard care in a meta-analysis of trial data with respect to death, major morbidity or resource use. Although in the meta-analysis there was a reduction in severe AKI associated with viscoelastic tests, this was based on an analysis of 62 events in four trials, all of which were at high risk of bias. There was also a reduction in major bleeding attributable to viscoelastic testing in the Karkouti et al. trial;129 however, this was a composite end point and the frequencies of individual components of the endpoint were not available, despite a direct request to the authors, limiting our ability to evaluate these results. The trial by Karkouti et al.129 also failed to demonstrate any difference in overall complication rates, although again the exact complications and their frequencies were not specified. Overall, the results of this trial mirrored the findings of the 2016 Cochrane review,156 notably that the use of viscoelastic tests reduced transfusion rates but had no benefit with respect to objective clinically important end points.
The COPTIC study was observational and therefore the analyses cannot prove cause and effect. However, the COPTIC study was at low risk of bias for many of the domains considered in the quality assessment of predictive accuracy studies.166 It also included more participants than the combined number of participants in all of the trials that were included in the systematic review by Whiting et al.26 and considered measured differences in the frequencies of important clinical end points rather than estimates derived from published risks attributable to blood transfusion. The results of the COPTIC study along with the results of our systematic reviews of RCTs evaluating the efficacy of POC devices do not support the current NICE recommendations.23,26
Summary and conclusions
What is known on this topic?
Severe coagulopathic bleeding is a common and severe complication of cardiac surgery. A recent technology evaluation by NICE23 concluded that the use of POC tests for the diagnosis and treatment of coagulopathic bleeding reduced the rate of transfusions and was cost-effective in cardiac surgery, resulting in a recommendation for their use. However, systematic reviews of the evidence supporting this guidance have shown inconsistencies with regard to whether these tests have predictive accuracy for bleeding or whether their use results in important clinical benefits. Importantly, the existing NICE guidance was based on estimates of resource use and outcomes from studies that have subsequently been superseded by new evidence.
What does this study add?
We performed a prospective observational cohort study that evaluated the predictive accuracy of POC tests that are in common use for the management of coagulopathic bleeding in cardiac surgery. We also evaluated a range of LRTs to establish whether the addition of these tests would increase predictive accuracy beyond that of existing POC tests. Our results showed that preoperative Multiplate testing did not improve predictive accuracy beyond the use of a clinical risk prediction score. Postoperative POC tests, including combinations of ROTEM or TEG plus Multiplate testing resulted in small incremental improvements in discrimination, as did measures of fibrinogen concentration or fibrinolysis. Although statistically significant, these changes were of uncertain clinical significance. We addressed this by undertaking a cost-effectiveness analysis of the introduction of these tests into routine clinical care using direct measures of resource use and survival from the COPTIC cohort and secondary sources to extend the time horizon to 5 years. We concluded that there was no clear difference in the cost-effectiveness of any of the POC test options compared with current practice. In a systematic review of RCTs that have evaluated the clinical effectiveness of POC test-based algorithms we concluded that there was no evidence of clinical benefit beyond reductions in transfusion. These findings are not congruent with the conclusions of a recent HTA of POC tests in cardiac surgery26 and the NICE guidelines based on these conclusions.
Future research
Ongoing and future RCTs comparing POC testing with the current standard of care in cardiac surgery are required to reconcile prevailing uncertainty over the clinical efficacy and cost-effectiveness of POC devices in current clinical use.
- Point-of-care coagulation and platelet laboratory testing in cardiac surgery: a ...Point-of-care coagulation and platelet laboratory testing in cardiac surgery: a predictive accuracy study with linked health economic analysis and parallel systematic review of efficacy trials of viscoelastometry - Diagnostic and therapeutic medical devices for safer blood management in cardiac surgery: systematic reviews, observational studies and randomised controlled trials
Your browsing activity is empty.
Activity recording is turned off.
See more...