NCBI Bookshelf. A service of the National Library of Medicine, National Institutes of Health.
IARC Working Group on the Evaluation of Carcinogenic Risks to Humans. Human Papillomaviruses. Lyon (FR): International Agency for Research on Cancer; 1995. (IARC Monographs on the Evaluation of Carcinogenic Risks to Humans, No. 64.)
1.1. Structure and molecular biology of human papillomaviruses
1.1.1. Structure of the viruses
HPVs form icosahedral non-enveloped particles with a diameter of approximately 55 nm. They contain a double-stranded, circular and covalently closed DNA genome of 7500–8000 base pairs (bp) (about 4950–5280 kDa), which is complexed by cellular histones (Klug & Finch, 1965; Pfister, 1987; Baker et al., 1991; Pfister & Fuchs, 1994). Papillomaviruses are resistant to organic solvents and to heating at 56 °C (Bonnez et al., 1994a). Virus particles have a buoyant density of 1.34 g/ml in caesium chloride and a sedimentation coefficient (S20.W) of 300 (Crawford & Crawford, 1963; Pfister et al., 1977).
Since papillomaviruses cannot be grown efficiently in tissue culture, studies on the architecture of the virus particles were originally restricted to those viruses that can be obtained from naturally occurring tumours, such as bovine papillomavirus (BPV), human papillomavirus type 1 (HPV-1) and cotton-tail rabbit papillomavirus (CRPV) (Crawford & Crawford, 1963; Finch & Klug, 1965; Klug & Finch, 1965; Favre et al., 1975; Pfister et al., 1977). More recently, it was demonstrated that virus-like particles of BPV-1, CRPV, HPV-1, -6, -11, -16, -18 and -33 can be obtained by expression of the structural proteins in recombinant vectors such as vaccinia or baculo-viruses (Zhou et al., 1991a; Kirnbauer et al., 1992; Hagensee et al., 1993; Kirnbauer et al., 1993; Rose et al., 1993; Christensen et al., 1994a; Le Cann et al., 1994; Pfister & Fuchs, 1994; Rose et al., 1994a; Volpers et al., 1994). The major capsid protein, L1, appears to be sufficient for particle formation (Kirnbauer et al., 1993). The antigenic and structural properties of virus-like particles were shown to be similar to those of virions (Hagensee et al., 1993; Christensen et al., 1994b; Rose et al., 1994a,b).
The virus capsid is composed of 72 capsomeres, which are arranged on an icosahedral surface lattice. Sixty of the capsomeres are six-coordinated and the remaining 12 are five-coordinated (Finch & Klug, 1965; Klug & Finch, 1965; Pfister & Fuchs, 1994). Analysis by cryo-electron microscopy has been performed on BPV-1 and HPV-1 particles (Baker et al., 1991). Capsomeres consist of a trunk with distal and proximal thickening. They associate at their base and project radially. Within each capsomere a central cylindrical channel can be visualized. Capsomeres exhibit a fivefold symmetry and, therefore, are composed of five identical molecules—most likely to be molecules of the L1 protein, which makes up about 90% of the total protein (Favre et al., 1975; Doorbar & Gallimore, 1987; Pfister & Fuchs, 1994).
The L1 proteins have a molecular mass of about 55 000 and are highly conserved among all papillomaviruses. Glycosylation of L1 protein of BPV-1 and HPV-16 (expressed by a recombinant vaccinia virus) has been reported, but the role of this modification in particle formation is unclear as yet (Mose Larsen et al., 1987; Browne et al., 1988; Zhou et al., 1993; Pfister & Fuchs, 1994).
The minor structural proteins, L2, have a molecular mass of about 75 kDa and are less-well conserved among papillomaviruses. Their position within the virus particle is not clear but their N-terminus has been shown to bind DNA in a sequence-independent manner, which suggests a role of L2 in packaging of the HPV genome (Komly et al., 1986; Pfister & Fuchs, 1994; Zhou et al., 1994).
1.1.2. Taxonomy
Papillomaviruses have been placed as a subfamily (Shah & Howley, 1992) together with polyomaviruses in the family Papovaviridae. Both groups of viruses have capsids with similar structure and double-stranded, circular, covalently closed DNA genomes. It is now known that the genome size and organization differ between the two virus groups and that there is no significant amount of nucleotide or amino acid sequence homology between individual viral genes, with the possible exception of some small protein domains (Clertant & Seif, 1984; Phelps et al., 1988). For these reasons the two groups should therefore be considered to be unrelated and placed in separate families (for a review, see Bernard et al., 1994a).
There have been several reviews of the classification of papillomaviruses, both by phenotypic aspects and sequence similarities (Chan et al., 1992a; Van Ranst et al., 1992a; Bernard et al., 1994a; Myers et al., 1994; de Villiers, 1994; Chan et al., 1995). Different papillomaviruses are classified as ‘types’. HPV types are defined by genomic analyses and therefore represent genotypes. Currently they are not classified by serology, and, therefore, the term ‘serotype’ is inappropriate (see section 1.2). At present, a novel HPV genome is described as a new HPV type if the nucleotide sequences of its E6, E7 and L1 genes (i.e. about one-third of the genome) differ by more than 10% from those of any previously described HPV type (de Villiers, 1994). Although this definition was established arbitrarily, it appears to be sufficient to define the natural taxonomic units—most recent isolates have been either almost identical to previously described HPV types, differing by less than 2%, or novel. The former are referred to as ‘variants’ or ‘subtypes’ (Gissmann et al., 1983; Deau et al., 1993; Ho et al., 1993a,b; Ong et al., 1993), as defined by nucleotide sequence comparison rather than by phenotypic analyses (see below). Two pairs of HPV types (HPV-34/64, HPV-44/55) differ by less than 10% of their nucleotide sequences, but their historic taxonomic status has not been revised (Chan et al., 1995).
To date, 70 HPV types have been described (Table 1) and their clinical origins and genomic sequences have been reviewed (de Villiers, 1989; Delius & Hofmann, 1994; Van Ranst et al., 1994; de Villiers, 1994). The genomic sequences are available in EMBL (European Molecular and Biological Laboratory) and GenBank databases and are edited by the HPV Sequence Database at the National Laboratory at Los Alamos (Myers et al., 1994). Studies of partial genomic sequences predict the existence of additional HPV types (Bernard et al., 1994a,b; Shamanin et al., 1994a; Berkhout et al., 1995).
Table 1.
Table Taxonomic association and origin of HPV types 1 to 70.
Taxonomic orders based on similarities in sequence of E6, E7 and L1 genes helped to identify groupings of related HPVs (Pfister & Fuchs, 1994). At the level of greatest diversity (‘major branches’), five supergroups of papillomaviruses can be distinguished, of which three contain HPVs (supergroups A, B and E) (Chan et al., 1995). Figure 1 shows these relationships in the form of a phylogenetic tree. This tree is based on nucleotide sequence comparisons and, at least on the level of supergroups, does not strictly reflect phenotypic or pathogenic similarities or differences.

Figure 1.
Relationship among 95 human and animal papillomaviruses, in the form of a phylogenetic tree
The largest supergroup, A, includes 44 HPV types and seven additional but untyped genomes (Chan et al., 1995). These types are phenotypically heterogeneous. In addition to all the HPV types that are found mostly in genital lesions, supergroup A contains several HPV types (e.g. HPV-2), that are normally associated with non-genital lesions such as common warts. The supergroup also includes HPV-7, which is normally found in ‘butcher’s warts' (Orth et al., 1981; Ostrow et al., 1981). Eleven groups can be distinguished within supergroup A, most of which unite phenotypically and pathologically similar HPV types. One of these groups contains HPV-16, -31, -33 and -35 and another contains HPV-18 and -45, the HPV types most frequently reported in association with malignant genital lesions. Yet another group contains HPV-6 and -11, which induce genital warts and other benign genital lesions as well as laryngeal papillomas. Supergroup B contains most of the HPV types associated with epidermodysplasia verruciformis (EV) such as HPV-5 and -8 (but with the exception of HPV-3 and -10, which are associated with verruca plana of EV-patients, although these viruses belong taxonomically to supergroup A). Phenotypically, this group is also not homogeneous as it includes several HPV types, such as HPV-4, that are found in cutaneous lesions of individuals not afflicted by EV. These HPV types are found on a separate branch remotely related to the other EV-HPVs. Recently, numerous novel HPV genomes, which also belong to the supergroup B, have been identified in squamous-cell carcinomas of immunosuppressed patients (Shamanin et al., 1994a; Berkhout et al., 1995).
1.1.3. Genomic variability
Genomic variability has been studied in detail in HPV-5 (Deau et al., 1993), HPV-6, -11 (Kitasato et al., 1994; Heinzel et al., 1995), HPV-8 (Deau et al., 1991), HPV-16 (Icenogle et al., 1991; Eschle et al., 1992; Ho et al., 1993a,b), HPV-18 and -45 (Ong et al., 1993). For these HPV types, the variability of the nucleotide sequence does not exceed 2% in coding and 5% in non-coding regions. The genomes of papillomaviruses evolve at rates at least five orders of magnitude slower than those of some RNA viruses, and 1% of genomic variability may originate over a period exceeding 100 000 years. Some particular genomic variants have been reported throughout the world and other variants appear to segregate according to ethnic groups. These findings strongly suggest that the known HPV types have existed in nearly identical molecular form since the beginning of the human species (Ong et al., 1993). Databases of genomic variation of HPV types are powerful tools for biomedical research because they allow for more refined studies of the epidemiology and pathology of HPV infection (Ho et al., 1993b).
Some nucleotide differences found in HPV-16 variants cause changes in amino acid codons (Icenogle et al., 1991; Chan et al., 1992b; Eschle et al., 1992). Examples of functional and antigenic alterations for L1 or L2 proteins of HPV-6 and -16 have been described (Kirnbauer et al., 1993; Yaegashi et al., 1993). Mutations altering binding sites for the transcription repressor YY1 have been recovered for HPV-16-associated cancers (May et al., 1994). Consistent variation in the HPV-16 E6 protein sequence has been observed in cervical cancers, which alters the peptide epitope for HLA-B7 in a way likely to influence immune recognition by cytological T cells (Ellis et al., 1995).
1.1.4. Host range
Papillomaviruses are highly specific for their respective hosts. No HPV type has been detected in any host other than humans, and, of the ~20 animal papillomaviruses described so far (one isolated from a bird, the others from different mammals; see section 4), most have been found in a single host species. Exceptions are the bovine papillomavirus types 1 and 2, which are also associated with equine sarcoids (see section 4). Hypotheses addressing specific interactions between viral and cellular proteins determining host specificity have been proposed (Shadan & Villarreal, 1993).
1.1.5. Related animal papillomaviruses
Phylogenetic studies suggest that papillomaviruses have co-evolved with their animal hosts. Lesions with a likely papillomavirus etiology are widespread among different taxa (Sundberg, 1987), but so far the genomes of only about 20 mammalian papillomaviruses have been cloned.
Four papillomavirus genomes have been cloned from monkeys and apes (O'Banion et al., 1987; Kloster et al., 1988; Ostrow et al., 1991; Reszka et al., 1991; Van Ranst et al., 1992b), and, as each of them is related to an individual HPV type, they may form the basis for useful animal models for the pathogenicity of HPV (Ostrow et al., 1991).
The molecular biology of two animal papillomaviruses, CRPV (Shope & Hurst, 1933) and BPV-1 (Lancaster & Olson, 1978) have been studied intensively. Methods developed in these studies have become powerful tools for the study of the molecular biology of HPVs (for a review, see Lambert et al., 1988). Although sequence alignments show only a small residual degree of similarity, it is likely that most, if not all, genes of BPV-1 correspond to those of HPV-16 and other genital HPV types. Consequently, the biology of BPV-1 proteins, such as those encoded by the E1, E2, E4, E5, L1 and L2 genes, can be considered as a model to understand HPV proteins. Important phenotypic differences exist, however, with a particularly large divergence of the transforming proteins E6 and E7 and of cis-responsive elements.
1.1.6. Target tissues
All papillomaviruses infect epithelial cells and, with the exception of the ungulate-specific fibro-papillomaviruses (e.g. BPV-1) which also infect dermal fibroblasts, they are restricted to this target cell population. The mechanism for this restriction is not clear since papillomavirus particles attach to and are taken up by cells derived from different tissues and different species (Roden et al., 1994a; Müller et al., 1995a). Epithelial-specific transcription activation is likely to play an important role in the selection of epithelial target cells (Cripe et al., 1987; Gloss et al., 1987).
1.1.7. Genomic structure and properties of gene products
The circular DNA genome of all papillomaviruses can be divided into three segments of unequal size. The long control region (LCR), also called the upstream regulatory region (URR) or non-coding region (NCR), represents about 10% of the genome (see section 1.1.8). The early (E) and late (L) genes are coded by about 50 and 40% of the genome, respectively.
The terms ‘early’ and ‘late’ genes are used for historical reasons rather than meaning a strict sequential expression of genes. For example, the product of the E4 gene seems to play a role late during the course of viral infection. The L genes both code for viral capsid proteins, whereas the E genes encode proteins with a variety of regulatory functions. The two L genes, L1 and L2, each encode a single protein. Of the E genes, three, E4, E5 and E7, apparently encode single polypeptide chains. The other three open reading frames, E1, E2 and E6, give rise to more than one protein through differential splicing (at least in some papillomavirus types). Figure 2 is a schematic representation of a typical papillomavirus genome and indicates the functions of the encoded proteins (for review, see Galloway & McDougall, 1989).

Figure 2.
Genome organization of papillomaviruses
Genes E5, E6 and E7 encode proteins with growth-stimulating functions. The properties of these proteins, which are relevant for the carcinogenicity of the virus, are summarized briefly here and are discussed in detail in section 3.1.3.
The E5 gene is located at the 3′ end of the early region, downstream from the E2 gene, and is probably expressed through a polycistronic mRNA that also encodes E2. E5 proteins are small polypeptides, 44 amino acids in BPV-1 and nearly twice that size in genital HPVs. Although they lack extensive homology they are all extremely hydrophobic and are located within the lipid bilayer of the cellular membranes. They interact with transmembrane domains of receptor kinases, thereby altering their half-life and responses to ligands (Horwitz et al., 1989; Martin et al., 1989; Conrad et al., 1993). E5 proteins bind specifically to a 16 kDa cellular protein, which is a component of vacuolar ATPases (Goldstein et al., 1991; Conrad et al., 1993). The diverse consequences of these interactions include changes in intracellular signalling, which may result in alterations similar to those brought about by mutations of ras genes in tumours that do not contain HPV genomes.
E6 and E7 genes are positioned at the 5′ end of the early region and are expressed in the form of a polycistronic mRNA transcribed from the E6/E7 promoter (P97 in HPV-16; Cripe et al., 1987). The two reading frames are spaced by a short variable distance (2 bp in HPV-16, 8 bp in HPV-18, excluding the termination codon of E6).
E6 proteins of some genital HPVs (size; 158 amino acids in HPV-16) associate with the tumour suppressor protein p53, thereby inducing ubiquitin-dependent degradation of p53 (Werness et al., 1990), which leads to elimination of the p53-dependent control of the cell cycle.
E6 proteins can also repress and activate transcription of heterologous and homologous promoters through interactions with p53 or with the basic transcription initiation complex (Lamberti et al., 1990; Desaintes et al., 1992; Lechner et al., 1992), E6 genes of HPV types involved in malignancy seem to be able to be spliced differentially to shorter proteins (E6*, E6**). The expression and function of E6* and E6** proteins in vivo are not understood, although the mRNAs are detectable and that for E6* is particularly abundant (for review, see Roggenbuck et al., 1991).
The properties of the HPV E7 protein have been reviewed (Münger & Phelps, 1993). The E7 protein of HPV-16 has 98 amino acids, forms a complex with the retinoblastoma tumour suppressor protein (pRB) and interferes with the binding of pRB to E2F transcription factors. A consequence of E7 expression is the release of E2F transcription factors, which activate the expression of several genes involved in the cell cycle (described in section 3.1.3).
E1 proteins initiate the replication of the papillomavirus genome. The E1 open-reading frame is transcribed into polycistronic RNAs that start at the E6/E7 promoter. Additional E1 transcripts of HPV-1, -6 and -11 start within the E7 genes and, in BPV-1, within E1 itself (Stenlund et al., 1985; Baker & Howley, 1987; Chow et al., 1987a,b; Rotenberg et al., 1989). In addition, experiments with BPV-1 point to the relevance of a promoter within the LCR for E1 expression (Stenlund et al., 1987). In spite of this large amount of data, it has not yet been resolved which one of these transcripts serves as the principal mRNA for translation of E1 proteins.
E1 is the largest open-reading frame of papillomaviruses and encodes two known polypeptide products of about 68 and 27 kDa. The function of the smaller product is not known (Hubert & Lambert, 1993). The 68 kDa protein binds to a specific sequence within the replication origin and is functionally necessary for replication in vivo and in vitro (Blitz & Laimins, 1991; Wilson & Ludes-Meyers, 1991; Spalholz et al., 1993) (see section 1.1.9). These E1 proteins have similarities with the ATP-binding sites of SV40/polyoma T antigens and can function as ATPases and helicases (Clertant & Seif, 1984; Bream et al., 1993; Seo et al., 1993; Yang et al., 1993).
E2 proteins regulate transcription and replication of papillomavirus genomes. Expression of the E2 gene is complex and probably occurs in several promoters in each papillomavirus. In BPV-1, the full-length E2 protein is derived from an mRNA initiating at the promoter P2443, a short distance 5′ of the E2-ATG. Two shorter transcripts include only the carboxyl-terminal part of E2. One of them starts at P3080, within the E2 gene. The other one starts upstream of E2 and includes, by differential splicing, an E8 leader peptide (for reviews, see Ham et al., 1991; McBride et al., 1991). The situation is less well understood in HPV-11 and -16, in which transcripts have been reported that initiate immediately upstream of E2 but also internally of E2. Other transcripts originate from the E6/E7 promoter or a promoter within E7 and are spliced in a variety of ways to different parts of E2 (Chow et al., 1987a; Rotenberg et al., 1989; Sherman & Alloul, 1992). Most of these experiments aimed at qualitative rather than quantitative data and, because of this, it is not clear which E2 mRNAs are translated.
The properties of E2 proteins have been reviewed (Giri & Yaniv, 1988; Ham et al., 1991; McBride et al., 1991). E2 genes of papillomaviruses encode proteins that differ in size from 410–430 amino acids in BPV-1, HPV-1 and EV-HPVs to about 370 amino acids in genital
HPVs. The proteins have highly conserved N-terminal transcription activation domains of about 200 amino acids and highly conserved carboxyl-terminal DNA binding domains of about 85 amino acids (Hegde et al., 1992). These two domains are linked by sequence-diverse hinge regions. E2 proteins form dimers through sequences of their carboxyl-terminal domain. E2 dimers are DNA-binding factors and their carboxyl-terminal domains have a high specificity for recognizing the sequence 5′-ACCGNNNNCGGT-3′ (Gauthier et al., 1991). Bound to DNA, E2 proteins can function as transcriptional activators and repressors or as modulators of replication (see sections 1.1.8 and 1.1.9). The two short forms of E2 proteins, which contain the carboxyl-terminal DNA-binding but not the N-terminal transcription-activation domain, function as repressors by competing for binding sites with the large E2 protein. These short E2 proteins are abundant in BPV-1 but are rare in HPV-infected cells.
E4 proteins seem to be involved in maturation and release of papillomavirus particles. In HPV-11-containing lesions, the E4 gene is expressed from an abundant spliced transcript that includes the beginning of the E1 open-reading frame (Nasseri et al., 1987; Stoler et al., 1989). E4 genes overlap with the variable central part of E2 genes, but E4 proteins differ from E2 protein sequences as they are encoded by a different reading frame. E4 proteins of different HPVs have little nucleotide sequence similarity but they share a high proline content (about 15%). E4 proteins form organized filamentous cytoplasmic networks that co-localize with cytokeratin filaments and, at least in vitro in the case of HPV-16, can induce the collapse of the cytokeratin network (Doorbar et al., 1991; Roberts et al., 1993).
L1 and L2 proteins are components of papillomavirus capsids. The late genes L1 and L2 are transcribed in BPV-1 and HPV-8 from a promoter in the LCR (Baker & Howley, 1987; Stubenrauch et al., 1992) and, during the generation of mRNAs, sequences corresponding to the early genes have to be spliced out from the primary transcript. An equivalent promoter has not yet been reported for genital HPVs. In HPV-6 and -11, transcripts starting 5′ of the L2 gene have been detected together with other transcripts that use the E6/E7 promoter or the E7 internal promoter P847. These are spliced to E4 and include the genes L2 and L1 (Chow et al., 1987a). It is not yet clear whether any of these promoters gives rise to the majority of the in-vivo translated L2 and L1 mRNAs. Nuclear localization signals direct the transport of the capsid proteins into the nucleus (Zhou et al., 1991b) where the viral particles are assembled.
1.1.8. Regulation of gene expression
The regulation of gene expression in papillomaviruses is complex—it is controlled by different cellular and viral transcription factors, different promoter usage, differential splicing, differential transcription termination and mRNA stability. In spite of significant insight into the mechanisms operative in vitro, it is still largely unclear how they interplay in vivo and what their relevance is during the normal papillomavirus life cycle. In principle, the mechanisms have to achieve the following: (i) epithelial-specific transcription; (ii) differential expression of papillomavirus genes during the differentiation of squamous epithelia, in particular the switch from early to late genes; (iii) feedback control by papillomavirus gene products, which may play an important role in the persistence of papillomavirus infections; and (iv) response to physiological factors of the infected host on papillomavirus gene expression. Many or all of these phenomena are deregulated during malignant progression of papillomavirus lesions.
Most of these regulatory events are controlled by protein factors bound to cis-responsive elements in the LCR (see Figures 2 and 3). The LCRs of genital HPVs range in size from 800 to 900 bp and are much shorter in many other papillomaviruses, particularly in EV-HPVs. The LCRs of all genital HPVs have a similar organization of cis-responsive elements, which deviates from that of EV-HPVs, BPV-1 and CRPV. This diversity may reflect biologically distinct mechanisms. Most of the following discussion concentrates on genital HPVs.

Figure 3.
cis-Responsive elements in the long control region of HPV-16
Figure 3 is a schematic representation of the HPV-16 LCR. Four E2 binding sites, typical for the LCRs of all genital HPVs, serve as landmarks in its molecular organization. Counting from the 5′ side, the first and second E2 binding sites divide the LCR into three functionally distinct segments.
The 5′ segment is about 300 bp long and is bracketed by the translation termination codon of L1 and the first E2 binding site (often referred to as E2 site No. 4, as it is most distal to the transcriptional start site). This segment contains transcription termination and polyadenylation sites for late transcripts as well as a negative regulatory element acting at the level of late mRNA stability (Kennedy et al., 1991). Additional regulation exerted by this segment may include transcription modulation function (Auborn & Steinberg, 1991). The distal E2 binding site influences transcription from the E6/E7 promoter (Romanczuk et al., 1990) but may also have yet undetected functions.
The central segment of the LCR is about 400 bp long (Gloss et al., 1987) and is flanked by two E2 binding sites. The properties of this segment have been studied in HPV-11,-16 and -18 and findings from these HPV types can probably be generalized to other genital HPV types. This LCR segment functions as an epithelial-specific transcription enhancer (Cripe et al., 1987; Gloss et al., 1987; Chin et al., 1989; Cid et al., 1993), which is probably an important mechanism for the epithelial tropism of HPVs. This enhancer is also modulated by physiological factors such as steroid hormones and by intracellular signalling pathways downstream of membrane-bound receptors. At least nine different cellular transcription factors have been reported to bind about 20 different sites in this part of the LCR in each of the three extensively studied genital HPVs. These factors are activator protein (AP1), papillomavirus enhancer binding factor (PEF1), glucocorticoid and progesterone receptors, nuclear factor (NFI), octamer binding factor 1 (Oct-1), transcriptional enhancer factors (TEF-1, TEF-2) and transcription repressor (YY1) (Gloss et al., 1987; Chan et al., 1989; Gloss et al., 1989; Chan et al., 1990; Chong et al., 1990; Sibbet & Campo, 1990; Chong et al., 1991; Bauknecht et al., 1992; Ishiji et al., 1992; Cuthill et al., 1993; Sibbet et al., 1995).
Several of these transcription factors induce epithelial-specific activation, although they occur in a similar form in cell types where no papillomavirus transcription can be measured in vitro. The enigma of this mechanism is that mutations in binding sites for these factors reduce transcription in epithelial cells, but occupation of the sites does not activate transcription in non-epithelial cells. The following observations may resolve this contradiction: two factors, NFI and AP1, are heterodimers and consist of subunits with antagonistic functions that are expressed differently in epithelial and non-epithelial cells (Thierry et al., 1992; Apt et al., 1993, 1994). In addition, the TEF-1 factor requires a cofactor, which is absent in some non-epithelial cells (Ishiji et al., 1992).
The enhancers of many genital HPVs are activated by glucocorticoid and progesterone receptors (Gloss et al., 1987; Pater et al., 1988; Chan et al., 1989; Cid et al., 1993) with the consequence of increased expression of the E6 and E7 genes. Mechanistically, glucocorticoid and progesterone act through the same cis-responsive elements. Different elements that might mediate responses to oestrogen, testosterone or retinoids have not been found so far. A repressive effect of retinoids on HPV gene expression has been observed (Bartsch et al., 1992) and it is possible that this mechanism is mediated by AP1 binding sites, as AP1 and retinoids are molecular antagonists (Fanjul et al., 1994).
The AP1 transcription factor is a downstream target of intracellular signalling cascades initiated by receptors for extracellular ligands such as epidermal, keratinocyte or tumour growth factors, but it is not known which of these hormones may have effects on HPV gene expression in vivo. E6 and E7 gene expression is strongly stimulated by tumour promoting substances, such as phorbol esters, which mimic some signalling pathways (Chan et al., 1990).
The principal role of the E2 binding site at the 3′ side of the central segment of the LCR is in replication initiation (see below). However, in vitro it can also modulate transcription of the E6 and E7 genes (Thierry et al., 1992).
This E2 binding site and the translation start codon of the E6 gene delineate the 3′ segment of the LCR, which is about 140 bp long. This segment contains a single E1 binding site, which identifies the origin of replication (see below). The transcription start site, which is only about 5 bp upstream of the ATG of E6, is located about 90 bp downstream of the E1 binding site. A segment of about 45 bp within these 90 bp contains a Sp1 transcription factor binding site, two E2 binding sites and a TATA box. These four sites provide a complex means for the modulation of E6/E7 promoter activity. The TATA box is required to establish the basic transcription complex and the Sp1 factor for mediating the long-range effect of the enhancer. The E2 sites overlap with the TATA box and with the Sp1 site in such a way that occupancy by E2 proteins displaces the basic transcription complex and the Sp1 factor (Dostatni et al., 1991; Tan et al., 1994a). As the E2 proteins also have transcription stimulation functions, differential occupancy of the two E2 binding sites leads to modulation of E6 promoter activity rather than to a simple switch. The interruption of negative regulation by E2 after chromosomal integration may play a role in progression of HPV-infected cells (Schwarz et al., 1985). However, integration does not seem to be a necessary prerequisite—many cancers contain episomal HPV copies (Fuchs et al., 1989; Matsukura et al., 1989; Cullen et al., 1991; Snijders et al., 1992a) and somatic cell hybridization of HPV-expressing cells with normal cells results in non-tumorigenic hybrids that continue to express E6/E7 genes from integrated genomes (Saxon et al., 1986; Koi et al., 1989; Bartsch et al., 1992).
The factor YY1 can repress as well as stimulate promoters. Each of the three segments of the LCR of HPV-16 and -18, and possibly of all genital HPVs, has one or multiple YY1 binding sites (Bauknecht et al., 1992; May et al., 1994; Myers et al., 1994; Bauknecht et al., 1995). Some of these binding sites repress E6/E7 transcription. Repression is relieved by mutational change of some YY1 sites in vivo resulting in mutant genomes with increased carcinogenicity (May et al., 1994).
Regulation of expression of the late genes is poorly understood in genital HPVs and, in spite of the cloning of late gene transcripts, no strong transcription start site for these genes has yet been identified. A promoter has been detected in HPV-8 that, in vivo, gives rise to transcripts encoding the late genes and, surprisingly, is stronger in transient transfections in tissue culture than the E6/E7 promoter of this virus (Stubenrauch & Pfister, 1994). This promoter is located in the 5′ part of the LCR of HPV-8, in a region similar to a site where a late promoter has been found for BPV-1 (Baker & Howley, 1987). Since the structures of the LCRs of HPV-8 and BPV-1 differ both from one another and from that of genital HPVs, the existence of a similar regulatory element in genital HPVs cannot be predicted.
1.1.9. Replication
The replication of papillomavirus DNA has been reviewed (Lambert, 1991). After initiation at a single site within the LCR, replication of papillomavirus DNA proceeds bi-directionally (Waldeck et al., 1984). In-vitro studies have shown that the replication start is identified by a single E1 binding site, which is located in the 3′ segment of the LCR. In genital HPVs, it lies approximately half way between the two E2 binding sites at the promoter and the single E2 binding site on the 5′ side of this segment (Mohr et al., 1990; Lu et al., 1993). The E1 protein recognizes A/T-rich motifs with only low sequence conservation, which, in the E1 binding site in HPV-16, has the sequence TAATACTTAAACTACAATAA. E2 protein forms heteromers with E1 protein in solution. These heteromers stimulate replication initiation by modulating E1 binding site recognition through binding of E2 to either of two flanking sites (Sverdrup & Kahn, 1994). Due to the overlap of alternative cis-responsive elements, replication can repress transcription from the E6 promoter (Sandler et al., 1993). The E1 protein/DNA complex initiates replication and requires additional cellular factors similar to those required for replication of SV40 (Seo et al., 1993). Papillomaviruses are able to control the copy number of their genomes in infected cells, a necessary prerequisite for episomal maintenance during persistent infection, and which is best exemplified in tissue culture by the ability of the BPV-1 genome to maintain a constant copy number of about 100 genomes per infected cell during prolonged amplification in mouse C127 fibroblasts (Lambert et al., 1988). The control mechanism is poorly understood, but probably involves control of the extension of the replication fork rather than control of initiation events (Roberts & Weintraub, 1986). Productive infection and replication is restricted to differentiating non-dividing cells.
1.2. Serological response
Further information on the mechanisms of the immune response can be found in section 3.2.
1.2.1. Antigenic properties of HPVs
Papillomavirus capsids contain both type-specific and group-specific antigens. The group-specific antigens appear to be hidden within the intact particle since they become recognized only after disruption of the virions (Jenson et al., 1980). Papillomavirus productive infection can be detected in vivo by staining sections from different lesions with a genus-specific antiserum (Jenson et al., 1980; Woodruff et al., 1980; Braun et al., 1983; Taxy et al., 1989; Lim et al., 1990).
Since papillomaviruses cannot be grown in routine experimental systems, information about the epitopes that are involved in the interaction between the virus and its host cell is limited. Studies on such epitopes have been restricted to those papillomaviruses for which biological assays exist, i.e. BPV-1 (transformation of rodent cells in culture and induction of fibropapillomas in bovine fetal skin xenografts), CRPV (induction of warts in cottontail rabbits) and HPV-11 (infection after xenografting of human tissue). Antisera and monoclonal antibodies tested against complete particles of either HPV-11, BPV-1 or CRPV proved to inhibit infection in a virus-specific manner (Christensen & Kreider, 1990; Christensen et al., 1990; Christensen & Kreider, 1991; Ghim et al., 1991; Jenson et al., 1991; Christensen & Kreider, 1993; Lin et al., 1993; Christensen et al., 1994a); neutralizing activity was found in L1-specific and L2-specific sera (Roden et al., 1994b). In sera of patients with genital warts or laryngeal papillomas, neutralizing antibodies to HPV-11 virions were detected (Christensen et al., 1992).
A variety of different seroreactive regions was identified within the L1 and L2 proteins of HPV-1, -6, -11, -16, -18 and -33 by using either experimentally produced antisera or human sera from individuals with or without clinical HPV-related disease (Table 2). Seroreactive regions within the HPV E4 and E7 proteins have recently been discussed by Viscidi and Shah (1992) and by Dillner (1994). The significance of such epitopes during HPV infection is unknown.
Table 2.
Seroreactive regions in HPV L1 and L2 proteins.
T-cell epitopes in HPV late proteins have been barely studied. Four T-cell determinants in the HPV-16 L1 protein were defined using synthetic peptides to stimulate peripheral blood mononuclear cells from asymptomatic individuals (Strang et al., 1990). This protein was also shown to contain three murine major histocompatibility complex (MHC)-class II-restricted T-cell determinants (Davies et al., 1990). For discussion of T-cell epitopes in HPV early proteins see section 3.2.3.
1.2.2. Immune response to papillomavirus infections
(a) Initial studies
Until recently, only very limited information was available about the humoral immune response to papillomavirus infections. There is no convenient experimental system for the large-scale production of papillomaviruses. Initially, only the structural proteins of those viruses that could be obtained in sufficient amounts (those from warts) were used as antigens. They were used in a variety of serological assays, such as immunoaggregation, immunodiffusion and radioimmunoassay (RIA) (Almeida et al., 1969; Pfister & zur Hausen, 1978; Pyrhönen & Neuvonen, 1978; Viac et al., 1978; Pfister et al., 1979a, 1981a; Kienzler et al., 1983; Steele et al., 1988; Anisimová et al., 1990; Steele & Gallimore, 1990; for review see Spradbrow, 1987). In these studies, HPV-specific antibodies were found to be associated with the presence of warts.
Nevertheless, some important aspects of the biology of papillomaviruses were discovered with the aid of serology. Work by Almeida et al. (1969) was the first to suggest that the papillomaviruses infecting the skin and the papillomaviruses infecting the mucosa are different from one another. The existence of a high prevalence of subclinical papillomavirus infections was first demonstrated through the detection of antibodies to HPV-8 particles in 10% of sera of asymptomatic individuals, in spite of the fact that HPV-8-induced lesions are extremely rare (Pfister et al., 1981a).
(b) Use of genetically engineered or synthetic viral antigens in serological assays
With the expression of recombinant papillomavirus proteins, it became feasible to measure antibodies directed to structural proteins of those HPV types that cannot be prepared from clinical lesions (e.g. the mucosotropic virus types such as HPV-16). In particular, the analysis of the humoral immune response against early proteins was not possible until the development of these genetically engineered reagents. In vitro recombination of the respective genes to heterologous promoters able to drive their expression efficiently and the introduction of such recombinant molecules into appropriate hosts, such as Escherichia coli, yeast, insect cells or mammalian cells, facilitated the production of the individual papillomavirus early or late proteins as well as of virus-like particles (Komly et al., 1986; Matlashewski et al., 1986a; Banks et al., 1987; Bernard et al., 1987; Seedorf et al., 1987; Thompson & Roman, 1987; Tomita et al., 1987a,b; Sekine et al., 1988; Tada et al., 1988; Sato et al., 1989a; Strike et al., 1989; Meneguzzi et al., 1990; Rose et al., 1990; Tommasino et al., 1990; Carter et al., 1991; Zhou et al., 1991a; Ghim et al., 1992; Kirnbauer et al., 1992; Stacey et al., 1992; Wilson & Ludes-Meyers, 1992; Bream et al., 1993; Hagensee et al., 1993; Kirnbauer et al., 1993; Park et al., 1993; Rose et al., 1993; Stacey et al., 1993; Hines et al., 1994). HPV proteins produced in prokaryotic or eukaryotic vector-host systems and synthetic peptides have all been used as antigens in different test systems, such as Western blot analysis, enzyme-linked immunosorbent assay (ELISA) and radioimmunoprecipitation. The different methods have been reviewed by Galloway (1992) and Gissmann and Müller (1994).
Expression of parts of proteins has been taken as a tool to identify seroreactive regions (Müller et al., 1990; Bleul et al., 1991; Jenison et al., 1991). Synthetic peptides also proved suitable for the definition of seroreactive regions (Dillner, 1990; Dillner et al., 1990a; Krchnák et al., 1990; Müller et al., 1990; Suchánková et al., 1992). A compilation of epitopes used by different investigators was published recently (Viscidi & Shah, 1992; Dillner, 1994).
(i) Individuals with unknown disease status
There is limited information about the development of antibodies following natural papillomavirus infection in humans. A few reports indicate the presence of Ig(immunoglobuline)G or IgM antibodies to HPV-16 E4, E7, L1 or L2 or HPV-6 L1 proteins in up to 33% of children one month to 10 years of age and 40% in children 10–20 years of age (Li et al., 1987; Jochmus-Kudielka et al., 1989; Jenison et al., 1990; Cason et al., 1992; Müller et al., 1995b). In view of the rare occurrence of clinically apparent papillomavirus infections in children, the significance of these data in unclear. No cases of seroconversion have so far been published.
Cross-sectional studies on the sera of healthy people demonstrated that antibody prevalence to HPV-16 E7 protein rises with age, whereas the prevalence of antibodies specific to HPV-16 E4 decreases in individuals over 20 (Jochmus-Kudielka et al., 1989; Müller et al., 1995b). Reactivity to HPV-16 virus-like particles was found to decrease in older women without HPV-16-related diseases (Jochmus-Kudielka et al., 1989). There is evidence that antibodies decline after disappearance of warts (Bonnez et al., 1993; Carter et al., 1994) and that antibodies develop more frequently in females than in males (Tachezy et al., 1994; Carter et al., 1995). Veress et al. (1994) reported a peak of seropositivity of anti-HPV (HPV-11 L2 or HPV-16 E2, E7, L1 or L2) secretory IgA antibodies among cytologically normal women aged 25–32.
Lewensohn-Fuchs et al. (1993) investigated the serological responses to HPV-16 E2, L1 and L2 antigens before and after renal transplantation. Antibodies to the late proteins were shown to decrease after transplantation but remained detectable throughout the study (up to three years) whereas the number of patients with E2-specific antibodies increased after transplantation. A significant difference was found between 120 renal transplant recipients and 215 controls in the prevalence of antibodies to HPV-16 E4 (32% versus 10%; odds ratio, 4.7; p < 0.00001) and E7 (15% versus 4%; odds ratio, 6.4; p < 0.00001) (Jochmus-Kudielka et al., 1992).
(ii) HPV-associated benign diseases
Antibodies to viral proteins of HPV-1, -6, -8, -11 or -16 are found in patients with papillomavirus-related benign lesions such as hand warts, condylomata acuminata, recurrent respiratory papillomas as well as in controls (Li et al., 1987; Steele & Gallimore, 1990; Steger et al., 1990; Suchánková et al., 1990; Bonnez et al., 1991, 1992; Carter et al., 1994; Kirnbauer et al., 1994).
The most remarkable differences in detection of antibodies between patients with benign lesions and healthy controls were measured when complete HPV particles were used, although a significant proportion of patients failed to react. In contrast, with synthetic peptides, reactivity was also seen in a proportion of sera obtained from asymptomatic individuals. In some studies applying bacterial fusion proteins or synthetic peptides, no differences were found (Jenison et al., 1990; Tachezy et al., 1994; for review see Viscidi & Shah, 1992).
By Western blot analysis using a bacterial HPV-6b L1 fusion protein, Li et al. (1987) found IgG antibodies in 18/30 sera from patients attending a colposcopy clinic and in 2/20 children below five years of age. Suchánková et al. (1990) detected IgG antibodies to a 17mer peptide shared between the HPV-6 and HPV-11 L2 proteins in the majority of patients with condylomata acuminata but in less than 15% of asymptomatic individuals.
HPV-11 particles produced from the mouse xenograft system were used to detect IgG antibodies by ELISA. The median optical density in patients with genital warts (0.32) or recurrent laryngeal papillomatosis (0.23) was greater than in controls (0.12 and 0.08, respectively) (Bonnez et al., 1991, 1992). It was demonstrated that in condyloma patients who responded to treatment, the median optical density dropped by 0.05% per day; it increased by 0.07% per day in non-responders (Bonnez et al., 1993).
Wikström et al. (1992) reported increased prevalence of serum IgG and Ig A antibodies to HPV-6 L1 and L2 peptides in men with a history of genital warts in comparison to controls (p < 0.05 for both L1 and L2). However, the antibody prevalence was similar to that of controls.
Using a carboxyl-terminal 19mer peptide of the HPV-16 E2 protein and an HPV-16 E7 derived 30mer peptide, IgA antibodies were found more frequently in cervical secretions of 29 patients with genital condylomata than in 28 controls (p < 0.025 and p < 0.005, respectively) (Dillner et al., 1993).
In recent studies, virus-like particles have also been used to detect, by ELISA, antibodies in sera of patients with HPV-related benign lesions. Carter et al. (1994) reported antibodies to HPV-1 capsids in 16 out of 18 women with current foot warts, compared to 38 out of 73 individuals without a history of foot warts (odds ratio, 7.2 (95% CI, 1.5–69.4); p < 0.01). There was a loss of seroreactivity with time since the last appearance of the warts.
Using an HPV-11 virus-like particle-specific ELISA, Rose et al. (1994a) tested sera that had been analysed previously by whole-virion ELISA; they found a significant correlation between the individual seroreactivities obtained in both assays (p < 0.000001).
Antibodies to HPV-6 capsids were found more frequently among women with recurrent genital warts than among female controls without history of genital warts (58% versus 19%, odds ratio, 6.5; 95% CI, 3.0–14,1). This association was even stronger in pregnant women (88% versus 30%; odds ratio, 15.5 (95% CI, 1.8–735.7)) but was essentially absent in males (31% versus 26%; odds ratio, 1.3 (95% CI, 0.6–2.6)) (Carter et al., 1995). These authors also reported a positive association between HPV-6 virus-like particle-specific antibodies and both HPV DNA positivity in cervical swabs and number of sexual partners.
Only a few studies addressing the cell-mediated immune response to HPV antigens have been reported. Lymphoproliferative responses to the HPV-1 E4 and HPV-16 E4, E6, E7 and L1 proteins were observed in human donors, and no differences were found between normal donors and patients with HPV-related diseases (Cubie et al., 1989; Strang et al., 1990; Altmann et al., 1992; Steele et al., 1993; Kadish et al., 1994). Kadish et al. (1994) reported that cell-mediated immune response to a carboxyl-terminal peptide of the HPV-16 E7 protein was significantly associated with infections by HPV-16, -31 or -33 as compared to infections by other HPV types. These authors suggest that cell-mediated immune response may be associated to regression of the disease.
(iii) HPV-associated precancer and cancer
A number of studies have compared the immune response to HPV-associated precancers and cancers. They are discussed in section 3.2.
1.3. Methods for detection of HPV infections
HPV infections are detected by demonstration of HPV genomic sequences in infected tissues and, to a lesser extent, by identification of HPV proteins in tissues. HPVs cannot be propagated or isolated in tissue culture and, therefore, one of the most common methods of virus detection is not available for HPVs. The presence of HPVs is also inferred from cytological, histological, serological and clinical findings.
HPV detection methods have been developed most extensively for genital tract HPVs, because these infections have been linked to cervical cancer. Of the numerous HPVs that infect the genital tract, some types (e.g. HPV-16, -18, -31 and -45) are frequently found in cervical cancers (high-risk HPVs) whereas others (e.g. HPV-6, -11, -42, -43 and -44) are rarely detected in cervical cancers (low-risk HPVs). Therefore, in clinical and epidemiological investigations, diagnosis of HPV aims at identification of specific types of HPVs, particularly those associated with cancer. In addition to the extensive studies on genital HPVs, recently developed polymerase chain reaction (PCR) assays designed to amplify EV-associated HPVs have detected large numbers of novel HPVs in skin lesions.
1.3.1. HPV diagnosis by morphology
The link between koilocytosis in cervical smears and HPV infection was established by cytological and histological investigations in the mid-1970s (Meisels & Fortin, 1976; Purola & Savia, 1977; Delia Torre et al., 1978). The presence of unequivocal koilocytes indicates productive viral infection with high specificity. However, with the advent of techniques for detection of viral genomes, it became evident that cytological and histological features are not sensitive indicators of the presence of HPVs. In a majority of individuals who are positive for HPV DNA, no cytological or histological correlates of HPV infection can be detected (Bauer et al., 1991). In contrast, colposcopic visualization of the cervix after application of acetic acid identifies cervical HPV infection frequently, but the specificity of the procedure is unknown. Therefore, indirect detection of HPV infection by cytology, histology and colposcopy is of limited diagnostic accuracy. Moreover, these methods fail to discriminate between lesions produced by different HPV types.
1.3.2. Detection of HPV proteins in infected tissues
Detection of HPV capsid antigen in affected tissue with a broadly cross-reactive antiserum raised against disrupted viral capsids (see section 1.3.3) identifies productive HPV infection with a high specificity. Detection of capsid antigen is highly correlated with the presence of koilocytes. However, this antigen is seldom detected in individuals who are infected with HPVs but have no cytological abnormalities or in individuals who have high-grade cervical neoplasia or invasive cancer (Jenson et al., 1985; Shah & Gissmann, 1989).
1.3.3. Detection of HPV genomic sequences
HPV genomic sequences are detected either by DNA amplification-based methods, in which targeted viral sequences are first amplified by PCR (Saiki et al., 1988) and then identified, or by direct hybridization of the genomes in the specimens, in which identification takes place without prior amplification. The limitations of the methods are related to their analytical sensitivity, clinical utility, complexity, reliability, ease of performance and commercial availability.
Differences in the methods of specimen collection (e.g. cervical scrapes versus cervico-vaginal lavage; the device used to collect specimens; the composition of transport medium) influence the reliability and analytical sensitivity of HPV diagnosis (Guerrero et al., 1992; Schiffman, 1992a). For adequate diagnosis, non-amplification-based assays require a larger amount of specimen than PCR methods.
(a) PCR-based methods
PCR-based methods are now the most commonly used in HPV investigations. They have the highest analytical sensitivity and can detect as few as 10–100 copies of HPV genomes in the tested portion of the clinical specimens. PCR assays require very small amounts of specimen, and a simple one-step treatment prior to amplification. Initially, HPV type-specific primers were designed with the use of computer-assisted matrix comparison analyses for different parts of the genome. Type-specific primer pairs are very efficient and highly specific for the amplification and identification of the chosen types. However, more than 40 genital HPVs were cloned and had differing oncogenic potential. Therefore, it was useful to devise a strategy to amplify a broad spectrum of HPV types in a single PCR reaction using primer pairs based on highly conserved sequences (consensus or general primers). A single amplification reaction with these primers generates PCR products that are then utilized for type-specific identification of HPVs. Several of the PCR methods in use are suitable for large-scale studies. Epidemiological investigations related to questions of disease etiology, prevalence, persistence and latency of HPVs rely heavily on PCR-based diagnoses. False positive results due to contamination, which were frequent in the early studies, appear to be rare in more recent investigations. Strong laboratory discipline is needed to avoid contamination. A proportion of clinical specimens may be unsatisfactory for PCR assays because the cellular DNA is not amplifiable.
The PCR methods for diagnosis of genital HPVs have been reviewed (Gravitt & Manos, 1992; van den Brule et al., 1993; Walboomers et al., 1994). The main features of the two most widely used methods, as currently used, are shown in Table 3. The modifications in these methods, since their original descriptions (Manos et al., 1989; van den Brule et al., 1990; Snijders et al., 1990), are referenced in the footnotes of Table 3. Both methods target sequences in the L1 gene and detect HPVs in over 90% of invasive cancers (Walboomers et al., 1994; Bosch et al, 1995).
Table 3.
Comparisons of two widely used HPV-PCR methods.
Both of the above methods employ consensus (or general) primers, which amplify a broad spectrum of HPV types in a single reaction. The primers are complementary to conserved sequences in the L1 gene of HPVs. However, the different HPVs do not have identical sequences, even in the most conserved regions, so the goal of amplification of many HPV types in a single reaction is met by the design of primers. The MY09–MY11 primers are degenerate, i.e. they are a mixture of many primers, with nucleotide differences at several positions that render them complementary to the target DNAs of different HPV types. The GP5+/GP6+ primers are non-degenerate, i.e. they are a single set of primers that accept nucleotide mismatches. In the amplification reaction with GP5+/GP6+ primers, the stringency of primer annealing is reduced, so as to allow the primers to anneal to the target DNA, despite the mismatches in nucleotide sequences. The GP5+/GP6+ primers are more efficient in amplification of sequences from archival material where DNA may be degraded, because they target a smaller fragment of the gene.
The HPV sequences in the PCR products are identified to specific types in a number of ways. In both the MY09–MY11 and GP5+/GP6+ systems, type-specific probes for over 20 HPVs are available. These probes are employed to identify specific types in dot blot or Southern blot formats, or in an ELISA format. Alternatively, probe pools can be designed to distinguish high-risk HPVs from low-risk HPVs (Jacobs et al., 1995). PCR products may also be identified by their restriction enzyme digest patterns or by nucleotide sequencing (Lungu et al., 1992; Smits et al., 1992a; Bernard et al., 1994a). The nucleotide sequence of the amplified product can then be matched to the HPV nucleotide sequence database (Myers et al., 1994) in order to make a type-specific identification or to identify new HPV types. For establishment of a novel HPV type, the whole HPV genome must be cloned and evaluated at the HPV reference centre at Heidelberg.
The above two methods, MY09–MY11 and GP5+/GP6+, both amplify a region of the L1 gene of genital HPVs. Lungu et al. (1995) recently described a PCR method with amplification of E6 sequences of genital HPVs. The primers are designed to amplify selectively 10 high-risk HPVs. PCR methods have also been devised for the detection of a broad spectrum of cutaneous HPVs (Tieben et al., 1993; Shamanin et al., 1994a). Use of these methods in the study of skin cancers in renal transplant recipients has revealed that many of these lesions contain novel HPV types related to the EV-associated HPVs (Shamanin et al., 1994a; Berkhout et al., 1995).
(b) ViraPap™ and Hybrid Capture™
ViraPap™ (Digene Laboratories, Silver Spring, MD, USA) was one of the first commercially available HPV diagnostic tests and is approved by the US Food and Drug Administration (FDA). In this test, radioactive RNA probes of seven HPV types (HPV-6, -11, -16, -18, -31, -33 and -35), available as a single pool or as three separate pools (ViraType™—HPV-6 and -11; HPV-16 and -18; HPV-31, -33 and -35), are employed to hybridize cellular DNA of clinical specimens placed on filters that are autoradiographed to reveal the hybrids in a dot blot format. The sensitivity and specificity of ViraPap™ as compared to other assays are discussed below. This test has now been replaced by a second-generation assay, Hybrid Capture™.
Hybrid Capture™ does not employ radioactive reagents and screens for a total of 14 HPVs with two RNA probe pools; probe A for five low-risk HPVs (HPV-6, -11, -42, -43 and -44) and probe B for nine types of HPV that are found in cancer (HPV-16, -18, -31, -33, -35, -45, -51, -52 and -56). The single-strand DNA in denatured specimens is reacted with the A and B RNA probes. The DNA-RNA hybrids are captured and immobilized with a hybrid-specific antibody and the hybrids are detected in an ELISA-type format with the use of a chemiluminescent compound. The presence of the viral DNA in the specimens is expressed as relative light units (RLUs). Higher ratios of specimen RLUs to control RLUs are indicative of a greater amount of viral DNA in the specimens, and in this way the test results allow quantification of viral DNA in the specimens (Cox et al., 1995).
The Hybrid Capture™ assay is available commercially and is approved by FDA. The analytical sensitivity of this test is 105 copies of HPV-16 genome. The negative and positive controls in the kit provide uniform standards for the testing laboratories. In a study of inter-laboratory variation in Hybrid Capture™ results among three laboratories, specimens were tested with probe pools A and B. Kappa values in inter-laboratory pairwise comparisons for positivity to either group A or group B ranged from 0.61 to 0.83. Of specimens that were positive for group B by the reference standard, 74% were positive in all three laboratories. The interlaboratory correlations of HPV quantitative data for probe B types ranged from 0.60 to 0.90. Probable false positive results were occasionally encountered, in less than 3% of the tests (Schiffman et al., 1995).
(c) Southern blot hybridization
In contrast to the PCR-based methods and Hybrid Capture™, which employ crude extracts of the clinical specimens as a starting point, Southern blot hybridization requires the purification of cellular DNA with a series of phenol:chloroform extractions. The purified cellular DNA is digested with restriction enzymes, electrophoresed, denatured, transferred to filters and hybridized at different stringencies with radiolabeled HPV probes. The identification of HPV types is based on the sizes of the hybridizing fragment and the stringency of hybridization.
The analytical sensitivity of Southern blot hybridization is similar to that for Hybrid Capture™. The sizes of the hybridizing fragments provide an internal control of the specificity of the annealing reaction; therefore, faint signals in Southern blot hybridization, if they correspond to the expected positions of the bands, can confidently be interpreted as positive. The test allows identification of viral subtypes and can provide evidence of viral integration.
The technique is labour-intensive and complex and employs radioactive probes. It is therefore not commonly used in large-scale epidemiological investigations. There is a significant inter-laboratory variation in detection and typing of HPVs by Southern blot hybridization. In a study of 40 clinical samples, pairwise agreement between four laboratories ranged from 66% to 97% for HPV detection and from 77% to 96% for typing of positive specimens (Brandsma et al., 1989).
(d) In-situ hybridization
This is the only method in which the viral genome can be identified in topographical relation to the pathological lesion. Cells or tissue sections on slides are hybridized using radioactive or nonradioactive DNA or RNA probes. Tests can be devised to detect viral DNA or viral transcripts of individual viral genes (Dürst et al., 1992; Iftner et al., 1992; Stoler et al., 1992).
The lower limit of detection of in-situ hybridization is 20–25 copies of the viral genome in the cell (Schneider et al., 1991). This limit of detection can be reduced further to one copy per cell by the use of special procedures such as in-situ PCR (Nuovo et al., 1991a,b; Bernard et al., 1994c).
Although labour-intensive, in-situ hybridization is very well suited for studies of molecular pathogenesis of HPV-associated diseases. The method can locate the viral genome in the tumour cells themselves, and correlate expression of specific viral genes with the evolution of the lesion. However, the technique is not suitable for epidemiological investigations, not only because of its complexity, but also because tissue specimens are generally not available from controls.
(e) Filter in-situ hybridization
This was the first HPV test designed for epidemiological study and was employed in several large-scale investigations (Reeves et al., 1989; de Villiers et al., 1992). In this assay, cells are placed on a filter and lysed, and the DNA on the filter is hybridized with radiolabeled probes and autoradiographed (Wagner et al., 1984).
This test is now seldom employed in HPV investigations. The test had a high inter-laboratory variability, and in one study there was no correlation between the results of filter in-situ hybridization and the results of Southern blot hybridization (Schiffman, 1992a).
(f) Comparison of HPV testing methods
Results of selected studies comparing HPV testing methods are summarized in Table 4. ViraPap™ is compared to two reference tests (Southern blot hybridization and PCR) for detection of the seven HPV types included in ViraPap™. Southern blot hybridization is compared to PCR as the reference test. The specificity of both ViraPap™ and Southern blot hybridization was high, but their sensitivities varied over a wide range. The results of these comparisons should be interpreted with caution because the technical details of the tests between laboratories performing the tests were not identical. Also, in some instances, the small amounts of specimens available may have contributed to lower sensitivities of non-amplification-based assays (Guerrero et al., 1992; Schiffman, 1992a).
Table 4.
Comparison of sensitivity and specificity of ViraPap™ and Southern blot hybridization.
1.3.4. Detection of HPV infections and HPV-associated cancers by serological assays
The immunological responses to HPV infections and to HPV-associated cancers have been discussed in section 1.2 and 3.2. Currently, the ELISA antibody assay using virus-like particles as the antigen is the most promising assay for the detection of virus-specific antibody. However, the antibody response is low-titred and not detectable in all patients with documented infections. Likewise, at present, antibodies to E6 and E7 proteins are the most promising markers of HPV-associated invasive cancer.
These assays are now widely employed in HPV investigations. The results of these studies will indicate the extent to which the assays are useful as markers of HPV infection and of HPV-associated neoplasia.
1.4. Epidemiology of infection
Many detailed reviews of the epidemiology of genital HPV infection have been published (Koutsky et al., 1988; Beutner et al., 1991; Moscicki, 1992; Morrison, 1994; Schiffman, 1994; Schneider, 1994). Less is known about the epidemiology of non-genital HPV infections. This section focuses on new information and consistent themes that have emerged from studies of the transmission, incidence, prevalence and natural history of HPV infection. Within each subsection data on genital infections are presented before data on non-genital infections.
1.4.1. Transmission
Results from several observational studies indicate that genital HPVs are transmitted primarily through contact with infected cervical, vaginal, vulvar, penile or anal epithelium. Barrett et al. (1954) reported that genital warts developed in four to six weeks in wives of servicemen who had returned from overseas and who had had genital warts. Oriel (1971) reported that 64% of sexual partners of individuals with genital warts developed genital warts themselves after a mean interval of two to three months. Similar results have been reported by others (Teokharov, 1969; Barrasso et al., 1987a).
In studies of sexually inexperienced young women, HPV DNA and antibodies to genital types of HPV are rarely detected (Fairley et al., 1992; Andersson-Ellström et al., 1994; Gutman et al., 1994; Rylander et al., 1994; Carter et al., 1995; Critchlow & Koutsky, 1995). Additional studies among young women show a positive trend between increasing numbers of recent sexual partners and increasing prevalence of genital HPV infection (Rosenfeld et al., 1989; Moscicki et al., 1990; Ley et al., 1991; Bauer et al., 1993). In a cohort study of 18–20-year-old female university students with a low-risk profile for sexually transmitted diseases (STDs), the overall prevalence of genital HPV infection as determined by PCR-based analysis was 35%. The prevalence at enrolment among the 183 sexually experienced women ranged from 17% of those reporting only one partner to 83% of those reporting more than five partners (Critchlow & Koutsky, 1995).
The above results indicate that, among adolescents and young adults, risk of genital HPV infection with each new sexual contact is high. Unlike the transmission dynamics for certain bacterial STDs (Brunham & Plummer, 1990), core groups (that is, small segments of the sexually active proportion of the population with very high rates of partner change) do not appear to be necessary to sustain high rates of genital HPV infection in communities throughout the. world (Yorke et al., 1978). To what extent the high prevalence of genital HPV infection among populations with relatively low rates of partner change can be attributed to a high rate of infectivity, a long duration of infectivity or a combination of both of these is currently not known.
Statistical evidence of HPV type-specific concordance in couples has been reported in two studies. Among a consecutive series of 50 couples attending an STD clinic, 63% of men and 72% of women were found to have HPV infection, as determined by PCR-based testing of anogenital samples. Of the 50 couples, five were excluded because one or both partners had an inadequate HPV DNA sample. In 20 couples both partners were HPV positive, in 21 couples only one partner was positive, and in four couples both were negative. Thirteen couples had the same HPV type detected, whereas only 8 would have been expected by chance (p = 0.009) (Baken et al., 1995). Among 32 married couples, HPV-16 was detected in both the husband and wife in 8 couples, of whom four demonstrated identical HPV-16 variants (Ho et al., 1993a). Although findings from these two studies support the role of sexual transmission in the epidemiology of genital HPV infection, they also demonstrate that quite often the same HPV type or variant cannot be detected in genital samples from both members of a sexual partnership.
Papillomavirus concordance was also studied in a group of rhesus monkeys, the sexual mating and offspring histories of which were known (Ostrow et al., 1990). Rhesus papillomavirus type 1 (RhPV-1) DNA was detected in cervical specimens from 20 of 30 female monkeys that had mated with one of two male monkeys infected with RhPV-1. None of 11 female monkeys that had not mated with either of the infected males was positive. The ‘index’ male monkey developed a penile carcinoma that metastasized to the lymph node, and two of the female mating partners developed cervical carcinoma. Both tumours and metastatic tissue were positive for RhPV-1.
Infections in the oral cavity of genital types of HPVs and HPV-associated recurrent respiratory papillomatosis (rare conditions) may be related to oral-genital contact (Kashima et al., 1992a). The occurrence of juvenile-onset recurrent respiratory papillomatosis in infants and young children indicates that HPV infections may be transmitted from mother to infant, probably at the time of delivery. There have been reports of HPV DNA detected in amniotic fluid, although in these studies transmission of the virus to the infant was not demonstrated (Sedlacek et al., 1989; Armbruster-Moraes et al., 1993). Age of mother, birth order of the infant and mode of delivery are important determinants of transmission. Most infants who develop juvenile-onset recurrent respiratory papillomatosis are delivered vaginally rather than by caesarean section (Shah et al., 1986), and many are the first-born single or twin infant of women who tend to be younger than other mothers delivering at the same institutions (Kashima et al., 1992a). Rare cases of ano-genital warts in new-borns have been reported (Tang et al., 1978) and HPV DNA has been detected in mucosal scrapes and washes obtained from infants (Roman & Fife, 1986; Jenison et al., 1990; Fredericks et al., 1993; St Louis et al., 1993). However, results from transmission studies of infants are not consistent, and do not provide a clear indication of the rate of infection among neonates who are exposed perinatally. Also, HPV type-specific concordance between mother-infant pairs has been observed only rarely.
Case reports of HPV-16 periungual infections and carcinomas (Moy et al., 1989; Euvrard et al., 1993) and of ano-genital warts in toddlers and children in whom sexual abuse has been ruled out (Handley et al., 1993) indicate that HPV (both genital and non-genital types) may be transmitted digitally from one epithelial site to another. Blood-borne transmission of HPV has not been reported. Studies of children sharing glue pots show that common non-genital warts on fingers and hands may be transmitted by fomites (glue in this instance) (Rowson & Mahy, 1967). Although fomite transmission of genital types of HPV has not been demonstrated, HPV DNA has been detected on medical instruments and in laser plumes (Garden et al., 1988; Ferenczy et al., 1990).
Genital HPV infection may involve large areas of uro-genital, perineal, perianal, anal and scrotal epithelium. In men, a common site of genital warts is the base of the shaft of the penis (Chuang et al., 1984; Cook et al., 1993), a site that is not easily covered by a condom. For this reason, consistent and correct use of condoms probably does not protect against transmission of genital HPV as well as it does against transmission of bacterial STDs and human immunodeficiency virus (HIV).
A study by Mandal et al. (1991) included 105 men (median age, 26 years) without clinical evidence of anogenital warts who were attending an STD clinic. Pooled specimens consisting of exfoliated cells from the distal urethra, penile shaft, glans penis and anorectal junction were used for detection of HPV DNA using dot blot hybridization techniques. HPV DNA was detected in 21 (20%) men. Fifteen men (14%) regularly used condoms and 17 (16%) were circumcized but neither circumstance was associated with the detection of HPV DNA.
As with genital types (Oriel, 1971), transmission of non-genital types of HPV is probably more efficient in the presence of a macerated or abraded epithelial surface. Studies of plantar warts suggest that the virus may be transmitted from the soles of the feet of one individual to a concrete surface (such as found surrounding a swimming pool) to the feet of an unsuspecting individual walking barefoot on this abrasive, HPV-1 laden surface (Rasmussen, 1958; Koutsky et al., 1988). Common warts (verruca vulgaris) are frequently detected on the fingers and hands of butchers and workers who handle fish and poultry (Rüdlinger et al., 1989a; Melchers et al., 1993; Stehr-Green et al., 1993; Keefe et al., 1994a). Transmission of HPV (particularly HPV-7) in these occupational settings may be facilitated by the occurrence of accidental epithelial wounding from sharp objects and by the sharing of equipment and protective gear (Melchers et al., 1993).
1.4.2. Incidence
Few studies have been designed to provide estimates of the incidence of genital HPV infection. In one investigation of the general population of Rochester, MN, USA, the annual age- and gender-adjusted incidence of genital warts increased between the early 1950s and the late 1970s from 13 per 100 000 to 106 per 100 000 (Chuang et al., 1984). This time period corresponds to the years when the rates of other STDs were increasing dramatically in Europe and North America (Aral & Holmes, 1995). Additional studies using data obtained from a sample of private physician offices throughout the USA showed a 4.5-fold increase in the number of first visits for condyloma between 1966 and 1984 (Becker et al., 1987). In the United Kingdom, national STD data suggested a 2.5-fold increase in the incidence of condyloma for males and females between 1971 and 1982 (Koutsky et al., 1988). Incidence data for subclinical genital HPV infections are currently not available.
The incidence of common and plantar warts in the general population has not been studied. It has been suggested, from the data available, that the incidence is higher among children living in institutions than among those living in houses, and higher in children than in adults (Rasmussen, 1958; Massing & Epstein, 1963).
In children between 0 and 14 years of age, recurrent respiratory papillomatosis has an incidence per 100 000 of 0.7 in Denmark, 0.6 in the USA, 0.1 in Japan and of 2.8 in Thailand (Christensen et al., 1984; Ushikai et al., 1994).
1.4.3. Prevalence
Unlike incidence, which measures the rate of new infection in a population during a given time interval, prevalence measures the percentage of a population that has new, persisting or recurring infection at a given point or period in time. For genital infections such as gonorrhoea which are often symptomatic and clear with appropriate therapy, estimates of prevalence and incidence may be comparable. For infections such as HPV, which persist with or without treatment, measures of prevalence and incidence will be different. Host characteristics and clinical manifestations of incident versus prevalent cases are also likely to be different. Prevalence is an important measure of the burden of disease in a population. Measures of incidence are used to identify characteristics of individuals that are at risk of developing disease.
Using PCR-based HPV DNA detection methods, several investigators throughout the world have determined the prevalence of genital HPV infection among populations of women with normal Pap (Papanicolaou) smears (Table 5). Data in Table 5 are presented in descending order of positivity of HPV. However, the results from the various studies are not strictly comparable because Pap smears were evaluated by different criteria, and different HPV DNA assays, primers and methods of epithelial sampling were used (see Table 5 for references). Despite these methodological differences, some important conclusions can be drawn from the presented data. First, younger rather than older women are more likely to have HPV DNA detected in genital tract specimens. Secondly, HPV DNA is rarely detected in genital tract specimens of women who report no previous sexual activity (Fairley et al., 1992; Rylander et al., 1994; Critchlow & Koutsky, 1995). Thirdly, geographical differences in the prevalence of HPV infection are not readily apparent.
Table 5.
HPV DNA detection rates by PCR amplification among women with cytologically negative Pap smears.
Results from these studies also indicate that, as with women with cervical neoplasia (McCance et al., 1985; Lörincz et al., 1990; Bergeron et al., 1992), women with normal Pap smears are more likely to have HPV-16 infection than infection by any of the other classified types of HPV.
Sampling techniques for detecting HPV DNA may be less sensitive when used in men than women. Penile scrapings may yield limited cellular material and, thus, are often negative for HPV DNA when analysed by molecular methods that do not include an amplification step (Grussendorf-Conen et al., 1987; Barrasso, 1992).
In one study (Kataoka et al., 1991), urethral smears were obtained from 105 male Swedish Army recruits between the ages of 18 and 23 years. Their reported mean lifetime number of sexual partners was 1.4. Eighteen (17%) had HPV DNA detected in the urethral specimen. In addition, all those with visible lesions provided penile biopsy specimens for the detection of HPV DNA by a PCR-based method. Of these 39 men undergoing penile biopsy, 17 (44%) had HPV DNA detected in the biopsy specimen. Urethral specimens obtained from these same men were positive for HPV DNA in only 10 (26%).
Genital HPV DNA prevalence was also measured by PCR among 168 men (mean age of 46 years) and 327 women (mean age of 44 years) residing in Spain and 128 men (mean age of 46 years) and 308 women (mean age of 42 years) residing in Colombia (Bosch et al., 1994a). In Spain, 3.6% of men and 4.9% of women were positive for HPV, and in Colombia, 19% of men and 13% of women were positive.
Among men attending STD clinics for various reasons, higher prevalence estimates of genital HPV infection detected by PCR-based methods have been reported (84%, Wikström et al. (1991); 63%, Baken et al. (1995)).
Studies of HIV (human immunodeficiency virus)-infected men and women suggest that the prevalence of HPV infection as determined by PCR-based methods is high (over 75% for cervical infection among women and 54–55% for anal infection among men) (Caussy et al., 1990a; ter Meulen et al., 1992; Kiviat et al., 1993; Seck et al., 1994). The HPV type distribution among HIV-infected populations is similar to the type distribution seen in uninfected populations (Palefsky et al., 1990; Kiviat et al., 1993).
Recent data suggest that there may be geographical or ethnic differences in the prevalence of different variants of HPV. By sequencing segments from isolates of HPV-16 and -18 obtained from various patient populations from five continents, Ho et al. (1993b) and Ong et al. (1993) suggest that the observed distribution of variants of HPV-16 and -18 indicates that, to establish each phylogenetic branch, both of these viruses evolved separately for a period in both Africa and Eurasia. In addition, the investigators speculate that because representatives from both HPV-16 branches are present in American countries, the variants were probably transferred via immigration from Europe and Africa. Whether variants of oncogenic types of HPV are important in predicting increased risk for invasive cancer of the genital tract or anus remains to be determined.
Among adults, the prevalence of common warts among office workers and engineering fitters declined with age (Keefe et al., 1994a). Currently, data concerning the prevalence of subclinical infection by non-genital HPV types are not available.
1.4.4. Natural history
Little is known about the natural history of genital HPV infections. Nevertheless, clinical findings from a variety of studies suggest the following observations: (i) Although most individuals with genital HPV infection do not develop signs or symptoms that are brought to the attention of a clinician (Bauer et al., 1991), it is likely that many infections cause microscopically visible intraepithelial lesions that are never detected. (ii) Within a few years of initial infection, most individuals are clear of the molecular, microscopic and clinical signs of the initial infection (Evander et al., 1995). (iii) Severely impaired cell-mediated immunity appears to enhance replication of the virus, thereby increasing the probability of an individual developing new or recurrent lesions (Frazer et al., 1986; Halpert et al., 1986; Palefsky et al., 1990; Vermund et al., 1991; Kiviat et al., 1993; Maiman et al., 1993). (iv) Only a small percentage of individuals infected with an oncogenic type of HPV develop cancer (zur Hausen, 1990). (v) Cervical cancer is a common HPV-associated malignancy (Krone et al., 1995), perhaps in part because cells capable of proliferation at the junction of columnar and squamous epithelium are exposed to the surface and, therefore, are most susceptible to infection and transformation by HPV.
Additional findings that suggest how different clinical courses have evolved for specific types of genital HPVs include the following: (i) Most macroscopically visible genital warts are caused by HPV-6 or -11; HPV-6 in approximately two-thirds of cases and HPV-11 in one-third (Gissmann et al., 1983). (ii) Virtually all cases of recurrent respiratory papillomatosis are associated with HPV-6 or -11; HPV-11 in approximately two-thirds of cases and HPV-6 in one-third (Abramson et al., 1987). (iii) DNA from HPV-16, rather than DNA from other types of HPV, has been detected in genital tract specimens over the longest period of time (Hildesheim et al., 1994; Shoultz et al., 1994). (iv) High-grade cervical intraepithelial lesions, which often involve HPV-16 or -18, may arise spontaneously without prior development of a low-grade lesion (Koutsky et al., 1992). (v) Most patients with cervical cancers are infected with HPV-16 or -18 (Low et al., 1990; Lörincz et al., 1992; Bosch et al., 1995). (vi) In patients with cervical cancer, HPV-18 DNA is detected more often in adenocarcinomas, adenosquamous-cell carcinomas and small-cell carcinomas than in squamous-cell carcinomas (Barnes et al., 1988; Tase et al., 1988a; Stoler et al., 1991).
Persistence of HPV-16 variants was studied in a cohort of young women. A total of 127 HPV-16 specimens obtained from 40 women were tested for sequence differences in the non-coding region of the genome using single stranded conformational polymorphism of PCR-amplified material. Using this technique, 16 different variants of HPV-16 were detected, but only two variants accounted for over 50% of the infections. All HPV-16 specimens from 24 women who were repeatedly positive over two to six visits showed the same single stranded conformational polymorphism pattern at every visit. Sequencing of clones from a subset of specimens indicated that many women were infected by more than one variant, but that one variant seemed to predominate over time, with other variants detected only intermittently (Xi et al., 1995).
A variety of viral, host and exogenous factors may influence the course of HPV infection. The type(s) or variants of virus, anatomic site of infection and host response define whether a given genital HPV infection will be self-limited and escape detection, produce a clinically evident benign proliferative lesion, or slowly, over the course of several years, on average, induce malignant transformation of infected cells.
1.5. Pathology of HPV genital tract infection and evidence from pathology for progression to malignancy
A number of reviews have been written describing the changes that are associated with HPV infection of the epithelium of the male and female lower anogenital tract (Barrasso et al., 1987a; Fu et al., 1988; Fu & Reagan, 1989; Richart et al., 1992; Wright & Richart, 1992; Richart & Wright, 1994; Wright et al., 1994a). Similar reviews have been written for the putative HPV-related lesions of the skin (Price et al., 1988; Blessing et al., 1989; Jablonska & Majewski, 1994). At other epithelial sites, HPV infection has been described infrequently and few reviews are available linking HPV infection to histological changes at these sites.
1.5.1. Terminology
(a) Dysplasia and carcinoma in situ
By the late 1800s, the histological changes that occurred at the margins of invasive squamous-cell cancers of the cervix had been recognized and described (Williams, 1888). Their significance was not appreciated at the time, but these changes later came to be called carcinoma in situ (CIS), a term first introduced by Schottlander and Kermauner (1912) to describe cervical cancer precursors and later reintroduced by Broders (1932). Cullen (1900) first noted that these changes resembled the cytological and pattern alterations that were present in the adjacent invasive squamous-cell cancer and suggested that they may be precursor lesions.
Originally, only epithelia that contained atypical cells throughout their full thickness were regarded as cervical cancer precursors. However, with the introduction of exfoliative cytology, it became apparent that many cytoiogical atypias reflected histological changes that, although lacking the full thickness de-differentiation of classical CIS, nevertheless shared some of the cytological and histological changes associated with CIS, which was recognized as a cancer precursor. When it became recognized that there was a wide spectrum of changes associated with histological alterations thought potentially to be cervical cancer precursors, it became important to define these minor atypias better, to understand their biology more completely and to devise an expanded terminology with which to identify them. Reagan and Hamonic (1956) introduced the term ‘dysplasia’ to designate cervical epithelia that contained cytologically atypical cells but lacked full thickness de-differentiation. The dysplasias were further divided into mild, moderate and severe grades, depending upon their differentiation (see Table 6). It was implicit in this terminology that the higher the grade, the closer the lesion was in aggregate to invasion. This assumption was based upon the observation that higher-grade dysplasias resembled CIS and invasive cancer more closely than those of lower grade. CIS remained in the clinicians' minds, however, as the only true cancer precursor. Patients with CIS were generally treated by total abdominal hysterectomy, and patients with lesser degrees of epithelial change—the dysplasias—were either treated by cervical conization or followed prospectively without treatment.
Table 6.
Summary of terms used for HPV-related non-invasive cervical abnormalities.
(b) Cervical intraepithelial neoplasia (CIN)
With continuing clinical experience, it became obvious both to pathologists and clinicians that there was extremely poor inter- and intra-observer reproducibility in differentiating CIS from dysplasia. It was particularly difficult for pathologists to distinguish severe dysplasia and CIS, and clinicians became increasingly sceptical of the rationale for therapy dictated by the dysplasia-CIS classification system.
In view of this, and after the completion of a number of laboratory and clinical studies that were begun in the 1960s, it became apparent that severe dysplasia and CIS could not be distinguished reproducibly at any level and that the lesser degrees of atypia—particularly moderate and severe dysplasia—merged imperceptibly in objective measurements with the higher-grade lesions (Richart, 1973).
These observations led to the introduction of the term ‘cervical intraepithelial neoplasia’ (CIN) to designate the spectrum of cervical diseases thought to play a role in cervical carcinogenesis (Richart, 1973). The implication of the CIN terminology was that there was a continuum of change that began with mild dysplasia and ended with invasive cancer after passing progressively through the intermediate stages of intraepithelial disease. The clinical implications of this new terminology were that presumed precursor lesions should be treated based on their size and location—not simply on their histological grade. Although the CIN lesions were graded CIN I, CIN II and CIN III, the CIN III category now included severe dysplasia and CIS (see Table 6), and, in terms of treatment, there was a lower emphasis on hysterectomy in favour of out-patient-directed methods and conservation of the uterus (Richart, 1987).
As molecular data accumulated, it became apparent that the spectrum of atypical epithelial changes that occurred in the female lower genital tract and were etiologically related to HPV could best be described as a two-tiered, rather than a three-tiered, disease process, and the CIN classification was modified appropriately (see Table 6) (Richart, 1990). Those lesions commonly referred to as mild dysplasia, flat condyloma or CIN I, which were thought to be the result of an acute epithelial viral infection, were designated low-grade CIN. Those lesions that contained more severe cytological atypia, which were thought to be true potential cancer precursors and to require treatment, were designated high-grade CIN. The distinction between low-grade CIN and high-grade CIN was based upon an assessment of cytological atypia and the presence or absence of abnormal mitotic figures. However, it was emphasized that the diagnostic decision should be taken at an operational level as well as a morphological level so that the clinician could infer accurately from the diagnosis whether the pathologist believed that the lesion being diagnosed was atrue cancer precursor or not.
In several recent publications (Koutsky et al., 1992; Kiviat & Koutsky, 1993; Wright & Riopelle, 1984), it has been reported that, in incident cases of CIN, the mean age of low-grade CIN and high-grade CIN may be more similar than for prevalent disease and that some high-grade CINs may be the immediate result of high-oncogenic-risk HPV infection. High-grade CINs may occur de novo and may not require a pre-existing productive infection (Kiviat et al., 1992). More data are needed to clarify these observations.
(c) Squamous intraepithelial lesion (SIL)
At this stage, cytological nomenclature was highly disparate, owing to the difficulties in communication and comparison of quality-control procedures between laboratories. Recognizing this, and the problems caused by an extremely low degree of intra-observer and inter-observer reproducibility in cytological diagnoses, a group was convened in Bethesda, MD, USA, to devise a uniform cytological terminology (National Cancer Institute Workshop, 1989; Luff, 1992). This meeting further recognized that the molecular data are most consistent with a two-tiered, rather than a three-tiered, system. This new nomenclature, known as ‘The Bethesda System’ (TBS), introduced the terms ‘low-grade squamous intraepithelial lesion’ (LSIL) and ‘high-grade squamous intraepithelial lesion’ (HSIL) (see Table 6). Low-grade SIL includes CIN I or mild dysplasia, koilocytosis, koilocytotic atypia and flat condyloma. The high-grade SIL designation includes CIN II and CIN III or moderate and severe dysplasia and CIS.
(d) Other organs in the male and female anogenital tract
Intraepithelial lesions of the vagina, vulva, penis and anus are generally diagnosed using a modification of the CIN terminology system and are generally graded in three classifications as in the original CIN nomenclature. The presumed precursor lesions for these organs are referred to as vaginal intraepithelial neoplasia (VaIN), vulvar intraepithelial neoplasia (VIN), penile intraepithelial neoplasia (PIN) and anal intraepithelial neoplasia (AIN).
1.5.2. Temporal and spatial relationships between cervical cancer precursors and invasive cancer
(a) Histological observations
The original observations that suggested the concept of a cervical cancer precursor and that led to the term CIS were made by pathologists who noted that the epithelium overlying or adjacent to cervical cancers contained cytological and pattern alterations that were similar to those found in invasive cancers. This simple, but profound, observation led to the concept that cancers were preceded by a precursor state that could be recognized histologically. The invention of the colposcope by Hinselmann (1925) allowed gynaecologists to recognize clinically alterations in the cervical epithelium that could be diagnosed, by punch biopsy, as CIS. These alterations could then be treated to prevent the development of invasive cancer. However, it was not until Papanicolaou and Traut (1943) published their observations on exfoliated cells that the true implications of these early histological and colposcopical observations could be utilized as part of mass screening programmes and be translated into cancer-prevention schemes. Subsequent observers noted that the mean age of diagnosis of mild, moderate and severe dysplasia, carcinoma in situ and invasive cancer increased progressively and that this increase was accompanied by an increasingly large lesional size. Increasing lesional size was accompanied by an increase in gland and canal involvement, and that, further still, the larger the lesion, the more likely it was to contain areas of invasion. These were compelling observations in support of the progression of CIN to cancer (Koss, 1992).
(b) Microinvasive and early invasive cervical cancers
The most important direct pathological evidence that putative cancer precursors are, in fact, precancerous lesions was the histological observation of invasion arising from such lesions. Tongues of invasion ranging from only one or two cells to larger lesions can be observed to arise directly from surface CIN lesions or from intraepithelial lesions involving the endocervical glands. These tongues of microinvasive carcinoma (Wright et al., 1994b) may be single or multiple and are generally accompanied by a local inflammatory infiltrate and a desmoplastic response. For the cervix, the risk of metastasis depends upon the degree of stromal penetration. Microinvasive cancer with stromal penetration of 3 mm or less rarely metastasizes and such lesions are commonly treated conservatively by cervical conization alone.
(c) Clinical observations
Smith and Pemberton (1934) drew attention to the fact that patients who had invasive cervical cancer were commonly found to have had CIS in their prior biopsies; indeed, when patients with CIS diagnosed by biopsy were followed without treatment, a significant number of them developed invasion. Similar observations were made by Kottmeier (1961) who followed 31 women with CIS prospectively for at least 12 years. Seventy-two percent of these women developed invasive cancer. In a similar study in New Zealand (McIndoe et al., 1984), 131 patients with persistently abnormal Pap smears were followed for 4–23 years. Twenty-nine percent developed invasive carcinoma of the cervix or vaginal vault and 69% had persistent CIS, which was treated subsequently. These observations of the natural history of CIS suggest that, in the majority of patients, once it is established, CIS rarely regresses spontaneously. It is not clear why there is a discrepancy between the direct clinical follow-up observations and the data obtained from the population-based screening programme in British Columbia, which suggested that a high proportion of CIS regress without treatment. The ‘yawning gap’ between the cumulative incidence of CIS and that of invasive cancer which is seen in that study (Miller, 1992) is not concordant with clinical observations. The natural history of precursors of a lesser histological grade than CIS has been studied extensively. These studies have been reviewed by Östör (1993).
1.5.3. Histological changes in HPV-related lesions of the lower female genital tract
(a) Non-productive (latent) HPV infection
HPV is thought to infect the basal or parabasal cells of the squamous epithelium and to give rise either to a non-productive (latent) or a productive infection. The non-productive HPV infection is defined as one in which the virus's replication is synchronized with the cell cycle but in which none of the cytopathogenic effects of HPV can be detected—the epithelium appears normal cytologically and histologically. Although there is no direct evidence for a solely nonproductive HPV infection, there are a number of clinical observations that suggest that it occurs.
(i) HPV DNA can be detected in patients with what appears to be normal cervical epithelium. In HPV DNA-positive/Pap smear-negative patients, the risk of developing an abnormal Papanicolaou smear within two years is substantially greater than that of HPV DNA-negative controls (Koutsky et al., 1992).
(ii) It is a common and widely known clinical observation that patients who have no clinical or cytological evidence of HPV while in the interpartum state may develop HPV-related lesions during the relatively immunocompromised pregnant state, only for such lesions to remit without treatment post partum.
(iii) Patients who had organ transplants may develop HPV-related lesions of the genital tract (Penn, 1986).
(iv) Patients in whom HPV-related lesions have been treated may have detectable HPV DNA despite normal cytological, colposcopical and histological findings. Such patients are at increased risk of recurrence compared to HPV DNA-negative controls (Koutsky et al., 1992).
(b) Low-grade CIN
A number of authors reported that it was possible to distinguish between flat condyloma and a true CIN lesion (Meisels & Fortin, 1976). However, in subsequent studies it was reported that the distribution of HPV types in those lesions designated as flat condyloma and CIN are indistinguishable from one another (Kadish et al., 1986; Willet et al., 1989), and that due to this lack of consistent morphological features, the ability to make such distinctions has extremely low inter- and intra-observer reproducibility. In addition, there are no differences in nuclear DNA content, as both have diploid/polyploid DNA distribution patterns (Fu et al., 1983; Fujii et al., 1984). It is not thought to be possible to separate flat condylomas from low-grade CIN or SIL lesions.
Low-grade CIN (Figures 4 and 5) is, by definition, a lesion that is well differentiated and contains alterations that are characteristic of the cytopathogenic effects of a replicative HPV infection. Operationally, it is a lesion that is thought by the pathologist to be the result of an acute viral infection and not to represent a true cancer precursor. Low-grade CIN lesions can arise through infection by any of the anogenital HPV types and the cytopathogenic effects of one type compared to another are generally reported to be indistinguishable at the level of the light microscope; however, some investigators have reported that HPV-16-induced lesions are more pleomorphic than those induced by other HPV types (Crum & Levine, 1984; Crum et al., 1991)
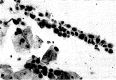
Figure 4.
Pap smear: low-grade CIN (SIL)

Figure 5.
Low-grade CIN
Most low-grade CIN lesions contain a thickened epithelium due to the acanthosis that accompanies epithelial hyperplasia, and many also contain papillomatosis. The basal and lower parabasal layers characteristically have little cytological atypia, are arranged in a uniform fashion on the basal lamina and are not highly disorganized. As viral replication begins in the upper parabasal and lower intermediate layers of the epithelium, it is accompanied by the characteristic cytopathogenic effects of HPV infection and cytological and organizational alterations become evident. The effects that are most characteristic of an HPV infection include binucleation, perinuclear cytoplasmic cavitation with a thickened cytoplasmic membrane, and, most importantly, nuclear atypia. The expression of the E4-encoded proteins in squamous epithelial cells causes the cytokeratin matrix to collapse due to a specific binding to cytokeratin proteins (Doorbar, 1991; Doorbar et al., 1991), possibly leading to the typical perinuclear cavitation, which is one feature of a productive HPV infection. The combination of nuclear atypia and perinuclear halo formation is referred to as koilocytosis or koilocytotic atypia (Koss & Durfee, 1955). These koilocytotic cells are the principal hallmark of a productive HPV infection of the cervical, vaginal or vulvar mucous membrane. It is important to emphasize that perinuclear halos may be produced as a result of vaginal infections or may accompany reparative or metaplastic processes.
The most characteristic histological feature of HPV infection, and the one that is most useful diagnostically, is nuclear atypia. HPV-related nuclear atypia is due to heteroploidy (Fu et al., 1981), which appears to result from mitotic spindle abnormalities and leads to DNA replication without cytokinesis. The precise mechanism responsible for the abnormalities in mitosis and cytokinesis is not clear. The results of the interference with the mitotic process are the formation of bi- and multi-nucleated cells and enlarged atypical nuclei, accompanied by heteroploidization.
In the low-grade lesions, the nuclei are principally diploid and polyploid. Generally, mitotic figures are increased in low-grade lesions though this is generally confined to the lower third of the epithelium as are undifferentiated or basal-type cells. Mitotic figures are characteristically absent from the upper layers of the epithelium in low-grade CIN. Most of the mitotic figures have a normal appearance, but cells with tripolar mitoses or tetraploid-dispersed metaphases may also be seen (Winkler et al., 1984). These two types of abnormal mitotic figures are also commonly found in polyploid lesions in other organs.
(c) High-grade CIN
High-grade CIN lesions (Figures 6–9) are substantially more atypical cytologically than low-grade CIN, have a higher degree of disorganization and have undifferentiated cells that extend past the lower third of the epithelium. This is reflected in the spectrum of HPV types found in low-grade CIN, which differs substantially from that found in high-grade CIN lesions (Matsukura & Sugase, 1995). In high-grade CIN, there is nuclear crowding, substantial pleomorphism, loss of both tissue organization and cellular polarity, and mitotic figures characteristically occur in the middle and upper third of the epithelium, in addition to the lower third. Cytological atypia that are found in high-grade CIN lesions differ substantially from those seen in the low-grade lesions. The nuclei in high-grade CIN are generally larger than those in low grade, their nuclear membranes are more prominent and tend to be convoluted and distorted, and the nuclear chromatin pattern is characteristically clumped, coarsely granular and contains prominent chromo-centers. As the nuclei enlarge, the nuclearxytoplasmic ratio is altered in favour of the nucleus, and the cell borders, which commonly contain visible desmosomes in the low-grade lesions, become indistinct and hard to define. It has been reported that the E6 protein, particularly, and also the E7 protein of HPV-16 induce chromosomal aberrations (White et al., 1994). The characteristic koilocyte of the low-grade CIN is generally absent or markedly attenuated in the high-grade lesions.
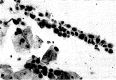
Figure 6.
Pap smear: high-grade CIN (SIL)

Figure 9.
High-grade CIN

Figure 7.
High-grade CIN

Figure 8.
High-grade CIN with glandular involvement
One of the most important features distinguishing high-grade CIN from low-grade CIN is the presence of abnormal mitotic figures (AMFs) (Winkler et al., 1984). Although many different types of AMFs are found in high-grade CINs, the most characteristic one is the three-group metaphase (i.e. chromosomal material on either side of the equatorial chromosomes in the metaphase) (Claas et al., 1992). Other AMFs that are commonly seen include the two-group metaphase, multi-polar mitoses in excess of three, lagging metaphase chromosomes, coarsely clumped chromosomes and highly abnormal, bizarre mitotic figures. AMFs are found in aneuploid lesions (aneuploidy is a marker for cancer or precancer) and have been reported to be the histological marker that best predicts the biological behaviour of CIN (Fu et al., 1981). As virtually all high-grade lesions and invasive squamous-cell cancers of the cervix are aneuploid, and as AMFs are an excellent surrogate marker for aneuploidy (Bergeron et al., 1987a,b; Fu et al., 1988), these mitotic abnormalities serve as a useful objective marker to distinguish between low-grade and high-grade CIN. In the presence of an AMF, a lesion will consistently be aneuploid and will be a true cancer precursor. In the absence of AMFs, the other histological features commonly used to classify these lesions should be taken into account.
(d) Microinvasive and invasive squamous-cell cancer of the cervix
Microinvasive squamous-cell cancer of the cervix ( Figure 10) is a single or multiple irregular tongue of neoplastic squamous epithelium that breaks through the plane of the basal lamina and invades the cervical stroma or epithelial lamina propria. Characteristically, areas of microinvasion are better differentiated than the high-grade CIN from which they most commonly arise. They lack the smooth contour and crisp demarcation from the subjacent stroma that is found in both surface high-grade CIN and high-grade CIN with glandular involvement. Areas of microinvasion infiltrate in an irregular fashion, splitting collagen bundles. Microinvasive foci are commonly accompanied by an inflammatory and desmoplastic response. Microinvasion is defined as an invasive lesion that invades the cervical stroma to a depth of no more than 3 mm, and, frankly, invasive cancer ( Figure 11) has a similar histological appearance to microinvasion but has invaded more than 3 mm into the cervical stroma. There is no convincing evidence that the histological appearance of invasive cancer or the patient's prognosis can be predicted from the HPV type that has produced the lesion.

Figure 10.
Microinvasive squamous-cell carcinoma

Figure 11.
Pap smear: invasive squamous-cell cancer
(e) Vaginal intraepithelial neoplasia
The histological changes in the vaginal mucous membrane associated with HPV infection and HPV-induced neoplasia are similar to the changes that are seen in the cervical mucous membrane.
(f) Vulva
The most characteristic HPV-reiated lesion found on the vulva is the acuminate wart. Condyloma acuminatum, which is almost always caused by HPV-6 or -11 (Gissmann & zur Hausen, 1980; Gissmann et al., 1982; Nuovo et al., 1990; Matsukura & Sugase, 1995), is, by definition, an exophytic lesion. It has cytological and histological features and organizational alterations similar to those seen in the cervical and vaginal mucous membranes, except for the presence of substantial acanthosis and papillomatosis. Condylomata acuminata occurring on the mucous membranes characteristically have the full constellation of HPV-related cytopathogenic effects, including koilocytosis. Warts that occur in the keratinizing epithelium, however, commonly contain minimal cytological atypia, and koilocytes may be difficult to identify, particularly in clinically older lesions.
The intraepithelial lesions of the vulvar skin have a much more complicated histological pattern than those of the mucous membranes of the cervix and vagina (Wilkinson, 1994). It is common to distinguish three different VIN types histologically — basaloid, warty and well-differentiated. The basaloid type, as the name implies, is composed generally of small fairly uniform cells that are hyperchromatic and contain alterations in nuclear chromatin distribution patterns. They tend not to be highly active mitotically, and abnormal mitotic figures are seldom encountered. Warty type VIN is generally a highly pleomorphic lesion with multinucleated cells, cytological atypia, coarse chromatin clumping, large numbers of mitoses and AMFs. The warty type VIN is commonly associated with koilocytosis, and adjacent condylomatous-type changes are frequently seen. The well-differentiated type of VIN is characteristically composed of a complex, proliferative lesion, which is only minimally altered in pattern and contains minimal nuclear atypia. Dyskeratosis is a common feature. High-risk HPV types are found principally in the warty and basaloid types of VIN. They are uncommon in the well-differentiated type.
(g) Vagina, anus and penis
Squamous neoplasms of the vagina are similar in morphology to those of the cervix. Squamous neoplasms of the anus are similar morphologically to those arising in other keratinizing epithelia, including the HPV-related lesions of the vulva. The anus has a squamo-columnar junction and a transformation zone similar to that seen in the cervix. Squamous-cell cancers and their precursors develop at the squamo-columnar junction and in the transformation zone of the anus, as in the cervix.
(h) Adenocarcinoma in situ and adenocarcinoma
Just as the squamous intraepithelial lesions occur on the squamous side of the cervical squamo-columnar junction, so adenocarcinomas in situ and adenocarcinomas occur on the columnar side. They are commonly associated with CIN lesions, particularly those that are high grade (Luesley et al., 1987). The endocervical epithelium appears not to support productive HPV infections, and low-risk HPV types have not been found in endocervical neoplasia (Higgins et al., 1992a).
Adenocarcinoma in situ is characterized by complex gland formation in the distribution of the normal endocervical glands, cytological atypia (Figure 12), an increased mitotic rate and a gland-within-gland pattern. Cytological alterations similar to those seen in other aneuploid cellular populations are present and AMFs are common. Adenocarcinoma in situ is distinguished from invasive adenocarcinoma (Figure 13) by virtue of its pattern and lack of demonstrable invasion.

Figure 12.
Pap smear: adenocarcinoma in situ
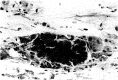
Figure 13.
Pap smear: invasive endocervical adenocarcinoma
1.5.4. Pathology of non-melanoma skin cancer and cutaneous HPV infection
(a) Cutaneous HPV infection
In EV patients, skin wart infection takes the following three clinical forms, each with characteristic clinical and histopathological features: common and plane warts (verruca vulgaris and verruca planar), which are also seen in the general population, and EV-specific lesions, namely red plaque-like lesions and scaly pityriasis-like lesions (Orth et al., 1979). In addition, seborrheic keratoses with typical EV-like histological changes have also been described in some patients (Jacyk et al., 1993a; Tomasini et al., 1993). The pathology of these lesions is reviewed by Jablonska & Majewski (1994).
(b) Non-melanoma skin cancer
Non-melanoma skin cancer refers to basal-cell and squamous-cell carcinoma and, conventionally, to the precancerous lesions actinic keratoses, Bowen's disease and intraepidermal carcinoma. This term also refers to keratoacanthoma, a common cutaneous lesion, which broadly resembles a squamous-cell carcinoma, but whose natural history is benign.
In immunosuppressed transplant patients, both the clinical and histopathological features of non-melanoma skin cancer differ. It is not possible to distinguish reliably keratoacanthoma and squamous-cell carcinoma in transplant recipients, for example, and for management and classification purposes they are referred to collectively as squamous-cell carcinoma. Similarly, in transplant recipients, actinic keratoses, intraepidermal carcinoma and Bowen's disease are not distinct entities. Since they are all thought to be dysplastic precancerous lesions, they are referred to collectively as verrucous keratoses (Blessing et al., 1989). The pathology of non-melanoma skin cancer and the cytological evidence for a putative role for HPV in these lesions has been reviewed (Price et al., 1988; Blessing et al., 1989).
1.5.5. Pathology of HPV-induced changes in the nude mouse system and tissue culture studies
When squamous epithelial cysts established beneath the renal capsule of nude mice are infected with HPV-11, changes occur similar to those seen in condylomata acuminata (Kreider et al., 1986). When human-cultured epithelial cells are transfected with HPV-16 DNA and allowed to differentiate, the morphological changes resemble high-grade CIN (McCance et al., 1988; McDougall, 1994).
1.6. Clinical disease of established HPV etiology (other than precancer and cancer)
Genital HPVs cause condylomata, laryngeal papillomas and some papillomas at other mucosal sites, e.g. the oral or sinonasal cavity and conjunctiva. Cutaneous HPV types and EV HPV types cause skin lesions. HPVs have been reported as being associated with many additional conditions, but the significance of these observations is as yet unclear (Shah & Howley, 1996). This section will only address benign conditions clearly associated with HPV.
1.6.1. Genital area
Condyloma acuminatum and genital wart are synonyms. The prevalence estimates of condyloma vary from 0.24 to 13%, depending mainly on the risk of sexually transmitted diseases (STD) and age distribution in the population examined (Kjaer & Lynge, 1989). The prevalence of condyloma in STD clinic patients was 11% compared with 2% in college students and was highest in the age group 16–24 years (Kiviat et al., 1989). Positivity for HPV DNA, which may reflect subclinical disease, was more than two-times higher than clinical disease in 377 first attendees of a STD clinic; 15% had genital warts, compared with 35% who were positive for HPV by ViraPap™/ViraType™ (Borg et al., 1993).
In women, the vulva, vestibule, vagina, perineum and perianal region are the most common sites for condylomata acuminata. HPV-6/11 was detected by Southern blot hybridization in up to 95% of condylomata acuminata (Gissmann et al., 1982; Johnson et al., 1991; Nuovo et al., 1991b).
Condylomata acuminata are rarely detected on the uterine cervix. HPV-6/11 was identified in 65% and HPV-16/18 in 8% of these lesions by Southern blot hybridization (Mitrani-Rosenbaum et al., 1988). Cervical condylomata may be hyperkeratotic and are sometimes confused with cancer owing to a bizarre pattern of vessels (Coppleson, 1991). The major capsid protein, L1, can be detected more often and in higher quantities in condylomata acuminata of the uterine cervix than in similar lesions of the penis or vulva (35% compared with 12% in a total of 95 cases) indicating a higher content of virus particles (Wools et al., 1994).
Genital warts are rarely observed in children. In a series of 25 children between seven months and 12 years in age, 74% of anogenital warts were HPV-6/11-positive and 17.4% were positive for HPV-2 by Southern blot hybridization. Clinical examination could not differentiate between HPV-2-positive and HPV-6/11-positive genital lesions. All children with HPV-2-positive condyloma also had cutaneous common warts which appeared to be due to auto-inoculation. One female newborn had anal warts one week after delivery and was probably infected in utero (Obalek et al., 1993). HPV-6-positive cutaneous lesions were found on an arm and a leg in a nine-year-old girl who was HPV DNA-negative in the genital tract and had no evidence of sexual abuse (Blauvelt et al., 1992).
In men, penile and urethral condylomata show a distribution of HPV types similar to that of genital warts in women. Lesions are condylomatous, macular, papular and may be keratotic (Barrasso et al., 1987a; Del Mistro et al., 1987; Zhu et al., 1993a; Labropoulou et al., 1994). Acuminate, papular and well-demarcated punctated lesions are most likely to contain HPV (Hippeläinen et al., 1993). In a series of 108 male patients, condylomata were located on the penile shaft in 51%, the shaft and perianal region in 14%, the shaft and scrotum in 2%, the shaft and urethral meatus in 15% and the urethral meatus alone in 18% (Rosemberg, 1991).
1.6.2. Upper respiratory and digestive tract and conjunctiva
HPV-11 is the most prevalent type (50–84%) found in laryngeal papillomas (Gissmann et al., 1983; Ushikai et al., 1994). When analysis is restricted to adult papillomas, HPV-6 is found most commonly (Corbitt et al., 1988). Recurrent respiratory papillomatosis originates in the upper respiratory tract where ciliated columnar and squamous epithelium are juxtaposed (Kashima et al., 1993). In adults with recurrent respiratory papillomatosis, biopsies of normal mucosa adjacent to papillomas were HPV DNA-positive in a majority of patients (Steinberg et al., 1983; Rihkanen et al., 1993, 1994), a finding that possibly explains frequent recurrences.
Oral verrucal-papillary lesions are clinically subdivided into verruca vulgaris, condyloma acuminatum, multiple and single papillomas, and focal epithelial hyperplasia (Scully et al., 1985). All of 10 verrucae vulgares from the lip in one series were positive for HPV-2 DNA (Eversole et al., 1987a), whereas the majority of oral lesions contained DNA from genital HPV types (Premoli-de-Percoco et al., 1993). Oral condylomata acuminata resemble genital lesions with a 85% (17/20) positivity rate for HPV-6, -11 or related types (Eversole et al., 1987b). HPV-13 and -32 are found almost exclusively in focal epithelial hyperplasia; 90% of these lesions are positive for one of these HPV types (Pfister et al., 1983a; Beaudenon et al., 1987). Of 202 cases of benign oral leukoplakia, 2.5% was positive for HPV-6/11 and 3.5% for HPV-16 by in-situ hybridization (Gassenmaier & Hornstein, 1988).
Conjunctival papillomas are positive for genital HPV types (Lass et al., 1983). The prevalence of HPV-6 in conjunctival papillomas detected by in-situ hybridization decreases with age (McDonnell et al., 1987). The authors suggested that HPV-6 infection of the conjunctiva is possibly acquired during passage through an infected birth canal.
1.6.3. Skin
Skin warts are classified according to macroscopic and microscopic morphological criteria. Infection with specific HPV types can be broadly correlated with these lesions (Gross et al., 1982; Croissant et al., 1985; Jablonska et al., 1985; Melton & Rasmussen, 1991).
(i) Typical common or mosaic warts, i.e. rough keratotic papules or nodules, on the hands, knuckles or periungual areas contain HPV-2, -4, -7, -26, -27, -28 or -29. Using PCR on specimens obtained from 111 immunocompetent patients, HPV-2a was found in 36% of the warts, HPV-2c in 23% (now known to be HPV-27; Chan et al., 1994a), HPV-57 in 12%, a variant of HPV-57 in 14%, HPV-7 in 4% and HPV-4 in 1% (one endophytic common wart) (Rübben et al., 1993). HPV-35 was found in a periungual wart of a patient with HPV-35-positive Bowenoid papulosis (Rüdlinger et al., 1989b).
(ii) Butchers warts have the clinical appearance of common warts but occur on the hands of those who work with raw meat, fish, and poultry. Among 60 butchers, HPV-1 was found in 6.7% of warts examined by Southern blot hybridization, HPV-2 in 45%, HPV-3 in 15%, HPV-4 in 10% and HPV-7 in 23% (Orth et al., 1981). A similar distribution of HPV types was seen with PCR analysis; 23/26 lesions were positive for HPV DNA: HPV-2 in 7.5%, HPV-4 in 11.5%, HPV-7 in 27% and unidentified HPV types (possibly containing HPV-1 or -3) in 42% (Melchers et al., 1993). In another series, HPV-7 was found by PCR in 74/112 (66%) warts of men working in meat-processing plants (abattoir workers and butchers) (Keefe et al., 1994b).
(iii) Filiform or papillomatous common warts found most frequently on the face, lips, eyelids or nares contain HPV-1, -2 or -7 (Jablonska et al., 1985; Egawa et al., 1993a). HPV-7 was found in two individuals with generalized or extensive facial warts with filiform appearance (de Villiers et al., 1986a).
(iv) Flat or plane warts, which can appear at different locations on the body and can form a linear arrangement (i.e. Koebner warts), are associated with HPV-2, -3, -10, -26, -27, -28, -29 or -41 (Melton & Rasmussen, 1991).
(v) Deep plantar warts, i.e. hyperkeratotic plaques or nodules on the plantar surface of the foot, are usually positive for HPV-1 or -4 (Jablonska et al., 1985; Rübben et al., 1993). HPV-associated epidermal cysts of the sole of the feet of 33 Japanese patients contained HPV-60 (Kato & Ueno, 1992; Egawa et al., 1994). HPV-63 and -1 were present in the same nucleus of one plantar wart (Egawa et al., 1993b).
Morphological and virological findings of skin lesions found in immunocompromised patients after transplant or in patients with EV are discussed in section 2.5.
1.7. Therapy and vaccination
When treating patients with HPV infections and/or HPV associated neoplasia, the sexual partner may also be infected and lesions may be multifocal or multicentric. Strict therapeutic guidelines have not been established for HPV-related lesions since no valid studies have addressed the issues of treatment of the sexual partner or multiple body sites.
1.7.1. Therapy of benign disease
The choice for treatment of genital warts is chemical or physical destruction. Chemo-destruction of genital warts with 20% podophyllin resin extract results in cure rates of between 35% and 45% (van Krogh, 1981; Rosemberg, 1991). In recent years, podophyllotoxin, the active ingredient in podophyllin resin, has become available for treatment of genital warts (Baker et al., 1990). A 0.5% podophyllotoxin solution reduced the mean number of warts from 6.27 to 1.1, destroyed about 70% of all warts and totally cleared the warts in 29–50% of patients (Baker et al., 1990; Bonnez et al., 1994b). In a randomized trial with 138 men and 67 women, 0.5% podophyllotoxin lotion was compared with 25% podophyllin solution. The former totally cleared 81% of warts compared with 61% by podophyllin (p < 0.001) (Kinghorn et al., 1993). In addition, podophyllotoxin solution is more efficacious than podophyllotoxin cream (Syed & Lundin, 1993). Podophyllin and its compounds should not be used in pregnant patients as it is a teratogen.
Topical trichloroacetic acid (TCA) is the alternative to podophyllin. It is applied topically to warts as a 50–85% solution and can be self-administered. A 85% solution gave a 60–80% eradication rate (Malviya et al., 1987; Menendez Velasquez et al., 1993). The advantage of TCA is that it can be used in pregnancy. The clinical cure rate of TCA in pregnant patients with cervical condylomata was 83% with a 85% solution (Menendez Velasquez et al., 1993).
Five percent 5-fluorouracil (5-FU) ointment is recommended only for treating extensive and recalcitrant HPV-related vaginal lesions (Krebs, 1991). However, care must be taken not to overtreat — chronic vaginal ulcerations have been reported in some patients (Krebs & Helmkamp, 1991). Vaginal stenosis, burning vulva syndrome or dyspareunia may also occur in some women after 5-FU treatment. 5-FU is contraindicated in pregnant women. The neoadjuvant effect of 5-FU after CO2 laser surgery is controversial (Reid et al., 1990) and may result in vaginal adenosis.
Excision, electrosurgical removal or cryotherapy are most frequently used for the treatment of HPV-related cutaneous lesions. After several sessions, cryotherapy or CO2 laser therapy lead to the complete cure of genital warts in the majority of patients (Bergman et al., 1984; Reid et al., 1985; Rosemberg, 1991). Similar success (78/91, i.e. 86% free of disease) is reported with the argon laser after one or two treatment sessions (Rotteleur et al., 1986). The Nd:YAG (neodium:yttrium aluminium garnet) laser cured only 30% of 20 men with urethral condylomata after one course of treatment; to attain successful treatment, 40% received up to five courses (Volz et al., 1994).
The antiviral, immunomodulating and antiproliferative action of interferon appeared to be promising for the treatment of genital warts. Although initial studies with recombinant α-interferon applied systemically or intra-lesionally resulted in significantly higher complete response rates in interferon-treated patients compared with placebo, i.e. 36% versus 17% (Eron et al., 1986) and 62% versus 21% (Friedman-Kien et al., 1988), two recent studies using various recombinant α-interferons found no significant effect (Reichman et al., 1990; Condylomata International Collaborative Study Group, 1993a). After initial promising results on the effect of interferon in an adjuvant setting after laser therapy (Reid et al., 1992), two recent studies showed no advantage when interferon was used after laser or cryotherapy compared with observation alone (Eron et al., 1993; Condylomata International Collaborative Study Group, 1993b).
Similar data have been reported for the adjuvant treatment of recurrent respiratory papillomatosis. A study with 66 patients used α-n1-interferon in a randomized crossover trial as an adjuvant to surgery; it was reported that the papilloma growth rate was slowed by interferon treatment (Kashima et al., 1985; Leventhal et al., 1988). In a study of 123 patients randomly assigned to surgery plus interferon versus surgery alone there was no adjuvant effect of interferon (Healy et al., 1988).
Since recurrent respiratory papillomatosis is a serious, recalcitrant disease that is difficult to treat, several drugs have been tested for their therapeutic efficacy in various small case series: 13-cis-retinoic acid showed some effect in combination with αa-interferon in three recurrent respiratory papillomatosis patients, increasing surgical treatment intervals by a factor of two (Lippman et al., 1994). Ribavirin showed a promising effect in the CRPV model (Ostrow et al., 1992). It was used in four patients with recurrent respiratory papillomatosis. Complete remission was seen in two adults and partial remissions in another adult and one child resulting in a prolonged interval between required surgeries (McGlennen et al., 1993). Photodynamic therapy was used in 33 patients who had moderate to severe recurrent respiratory papillomatosis. These patients were treated with dihaematoporphyrin ether intravenously 48–72 h prior to photo-activation with an argon pump dye laser system; a 50% decrease in papilloma growth rate was seen on average, with three patients remaining free of disease but continuing to be infected latently (Abramson et al., 1992).
1.7.2. Therapy of precancers
Cervical intraepithelial neoplasia (CIN) is the most commonly detected genital precancer in humans. The following section will refer mainly to data regarding cervical disease but the principles apply generally to treatment of HPV-associated precancerous lesions of other body sites.
There are no strict clinical guidelines for the therapy of CIN; interim therapeutic guidelines were established at a recent consensus conference in the USA (Kurman et al., 1994). However, there is general agreement that, prior to treatment, histological verification is mandatory in order to classify the lesion and to exclude invasive cancer. Overdiagnosis and overtreatment of lesions that are not CIN should be avoided. Ideally, therapy should be undertaken by a physician who understands the cytological, colposcopical, virological and histological aspects of cervical neoplasia and who takes into account the patient's physical and mental status as well. Treatment of CIN may be excisional or destructive. The main disadvantage of the destructive methods is that invasive cancer may be missed. The incidental finding of invasive cancer was reported in conization specimens in 0.8% patients in whom invasive cancer was missed by colposcopically directed biopsy preoperatively (Prendiville et al., 1989; Bigrigg et al., 1990; Luesley et al., 1990; Whiteley & Oláh, 1990). If invasive cancer is not detected in the triage procedure prior to destructive therapy, this diagnostic error may lead to recurrence and death (Sevin et al., 1979; Townsend et al., 1981). Destructive therapeutic techniques, such as CO2 laser vaporization or cryotherapy, should be confined to the management of purely ectocervical low-grade lesions confirmed by colposcopy, cytology and histology (Sevin et al., 1979; Baggish, 1982; Andersen &Husth, 1992).
Cold knife conization has been used for decades to treat CIN but is increasingly being replaced by the CO2 laser (Dorsey & Diggs, 1979) or high-frequency electrosurgical excision (large loop excision of the transformation zone, i.e. LLETZ, or loop electrosurgical excision procedure, i.e. LEEP) preoperatively (Prendiville et al., 1989; Bigrigg et al., 1990; Luesley et al., 1990; Whiteley & Oláh, 1990; Wright et al., 1992). Compared to LEEP excision, more tissue is removed by cold knife conization (15.8 mm versus 9.2 mm depth and 5.6 g versus 2.6 g of weight; p < 0.01) (Girardi et al., 1994). This is of special importance in young women since conization has a negative effect on fertility; in a case-control study following 56 patients and 112 controls, the duration of pregnancy was shorter (p < 0.001) and the incidence of premature delivery was higher (p < 0.0001) in women with a history of laser conization (Hagen & Skjeldestad, 1993). Similar data are reported for LEEP, after which 24% of women had babies with a birthweight of less than 2500g as compared with 7% in controls (p = 0.03) (Blomfield et al., 1993). Other studies found no adverse effects of LEEP on fertility or pregnancy outcome (Cruickshank et al., 1995; Ferenczy et al., 1995). In a comparison of LEEP and cold knife conization patients in a case-control study with 43 women in each group, there was a mean operating time of 2.8 min versus 14 min (p < 0.01), blood loss of 3.3 ml versus 79 ml (p < 0.01) and postoperative complications such as haemorrhage in 4.7% versus 20.9% (p < 0.05) (Oyesana et al., 1993a). The same authors compared LEEP with CO2 laser conization in a randomized trial with 150 patients in each group; significant differences were detected in operating time (2.5 min versus 24.2 min; p < 0.001), pain under local anaesthesia (7% versus 45%; p < 0.001), blood loss (2.77 ml versus 27.15 ml; p < 0.001) and thermal artifact on histological evaluation of the cone margins (5 versus 25 specimens; p < 0.01) (Oyesana et al., 1993b). Persistence rates for CIN after excisional treatment is about 4% (Prendiville et al., 1989; Bigrigg et al., 1990; Luesley et al., 1990). Persistence is more likely when the CIN involves the margins of excised tissue (Paterson-Brown et al., 1992; Lopes et al., 1993). The ‘see and treat’ approach using the LEEP technique (Keijser et al., 1992) eliminating histological evaluation prior to treatment should only be performed by experienced specialists who can ascertain whether or not invasive cancer is present. Many physicians prefer to obtain a histological diagnosis prior to treatment. Irrespective of the surgical technique, an endocervical curretage is usually performed following the treatment to verify that residual CIN is not present (Hatch et al., 1985).
Natural and recombinant interferon has been used as mono- and adjuvant therapy for treatment of CIN in a number of studies (Choo et al., 1986; Schneider et al., 1987a; Yliskoski et al., 1990, 1991; Schneider et al., 1995). A comparison of the therapeutic results is difficult since the studies vary in design, dosage, mode and duration of application and measurement of study outcome. Interferon is currently used principally for specific indications such as recurrent or extensive disease. Its therapeutic efficacy in the management of HPV-associated lesions remains controversial.
1.7.3. Vaccination
Therapeutic vaccination may potentially be used to treat patients with established HPV infections or HPV-associated precancer or cancer; prophylactic vaccination may potentially protect against HPV infection. Theoretically, protection can be achieved by induction of virus-neutralizing antibodies prior to exposure, and successful therapeutic vaccination will depend on stimulation of cellular immune response.
In animal models (BPV, CRPV and COPV), neutralizing antibodies produced after either natural infection or immunization with recombinant capsid proteins protect against subsequent viral challenge (Campo, 1991; Christensen & Kreider, 1991; Christensen et al., 1991; Jarrett et al., 1991; Lin et al., 1992; Campo et al., 1993; Chandrachud et al., 1995; Ghim et al., 1995). Antibodies often recognize conformation-dependent epitopes and are specific for individual papillomavirus types. Neutralizing antibodies are found in sera of HPV-positive patients (Christensen & Kreider, 1993; Kirnbauer et al., 1994), but regression or recurrence of HPV-induced lesions have not yet been correlated with previous antibody status. Recently, synthetic virus-like particles have been developed after expression of papillomavirus structural proteins in vitro (Zhou et al., 1991a; Hagensee et al., 1993; Kirnbauer et al., 1994). Studies using such particles in prophylactic vaccines will show whether anti-HPV neutralizing antibodies are able to protect against HPV infection.
Vaccination with non-structural papillomavirus proteins can generate a cellular immune response that may be effective in the treatment of papillomavirus-induced lesions. Regression of papillomas has been achieved in cattle by vaccination with BPV-4 E7 protein (Campo et al., 1993) and in rabbits by vaccination with CRPV E1 and E2 proteins (Selvakumar et al., 1995). In mice, the transforming proteins E6 and E7 of HPV-16 induce specific cytotoxic T cells (Chen et al., 1991, 1992a) or CD4+ delayed type hypersensitivity T cells (McLean et al., 1993), which both mediate rejection of transplanted E6 or E7 expressing cells. Elimination of virus-infected cells by cytotoxic T cells depends on proper MHC (major histocompatibility complex) class I-restricted presentation of virus-derived peptides by the target cell. However, HPV-infected cells may escape immune surveillance since MHC (major histocompatibility complex) class I expression is downregulated in CIN or cervical cancer (Connor & Stern, 1990; Cromme et al., 1993a). Several potential cytotoxic T-cell epitopes of HPV-16 E6 and E7 have already been identified (Kast et al., 1993). Such epitopes as well as the proteins themselves are candidates for therapeutic anti-HPV vaccines. Several first phase trials are already in progress (Muñoz et al., 1995).
- Structure and molecular biology of human papillomaviruses
- Serological response
- Methods for detection of HPV infections
- Epidemiology of infection
- Pathology of HPV genital tract infection and evidence from pathology for progression to malignancy
- Clinical disease of established HPV etiology (other than precancer and cancer)
- Therapy and vaccination
- Human papillomavirus (HPV) infection - Human PapillomavirusesHuman papillomavirus (HPV) infection - Human Papillomaviruses
- XCL2 AND (alive[prop]) (55)Gene
- PSD2 AND (alive[prop]) (932)Gene
- CCR8 AND (alive[prop]) (336)Gene
Your browsing activity is empty.
Activity recording is turned off.
See more...