NCBI Bookshelf. A service of the National Library of Medicine, National Institutes of Health.
Breman JG, Alilio MS, Mills A, editors. The Intolerable Burden of Malaria II: What's New, What's Needed: Supplement to Volume 71(2) of the American Journal of Tropical Medicine and Hygiene. Northbrook (IL): American Society of Tropical Medicine and Hygiene; 2004 Aug.
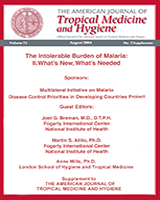
The Intolerable Burden of Malaria II: What's New, What's Needed: Supplement to Volume 71(2) of the American Journal of Tropical Medicine and Hygiene.
Show detailsAnemia is one of the commonest and most intractable public health problems in Africa. This paper illustrates how, in areas of stable malaria transmission, anemia is apparent from the first few months of life, with the highest prevalence towards the end of the first year. The antenatal and postnatal factors predisposing to anemia in infants and young children are discussed, together with the interventions that are available for prevention. The paper stresses the need to target interventions at pregnant women and infants, the groups at highest risk of anemia, and to develop an integrated, non disease-specific approach to this complex problem.
Introduction
Anemia (hemoglobin level < 11 g/dL) remains one of the most intractable public health problems in malaria-endemic countries of Africa. It affects more than half of all pregnant women and children less than five years old,1, 2 and has serious consequences since severe anemia (hemoglobin level < 5 g/dL) is associated with an increased risk of death,3 while iron deficiency and anemia may impair cognitive and motor development,4– 6 growth,7 immune function,8 and physical work capacity.9 The insidious nature of its presentation means, however, that mild-to-moderate degrees of anemia frequently remain undetected and untreated by health care workers and in the community,10, 11 while blood transfusion for severe anemia may be prescribed on the basis of inaccurate hemoglobin measurement,12 thus exposing the patient unnecessarily to the risk of infection with human immunodeficiency virus (HIV) and other blood-borne pathogens.13 Prevention is clearly of critical importance, yet current coverage with anti-malarial interventions and micronutrient supplementation is poor in many African countries.14 In these settings, the targeted delivery of interventions against anemia to high-risk groups (pregnant women and young children) may be an appropriate use of limited economic and human resources.
Age Pattern of Anemia in Areas of Stable Malaria Transmission
Measurement of hemoglobin on capillary blood using the HemoCue hemoglobinometer (HemoCue AB, Angelholm, Sweden) has been recently introduced as part of nationally representative household-level Demographic and Health Surveys (DHS) (ORC Macro. http://www.measuredhs.com). To date, hemoglobin measurement has been or is currently included in surveys from 13 countries in tropical Africa. Data on children less than five years old from surveys conducted in Benin (n = 2,568), Uganda (n = 6,003), Mali (n = 3,192), and Madagascar (n = 2,272), is shown in Figure 1 (courtesy of Dr. E. Korenromp, Roll Back Malaria Department, World Health Organization, Geneva, Switzerland). The proportion of the population exposed to four or more months of malaria transmission per year ranges from 55.5% (Madagascar) to 86.4% or higher (Uganda, Mali, and Benin).15 Figure 1a shows that hemoglobin levels continue to decline after the physiologic decrease that normally occurs in the first 2–3 months of life, reaching a nadir towards the end of the first year. Data from Benin and Uganda (Figure 1b) demonstrate that more than 80% of infants 10 months of age are anemic, and approximately one-third have hemoglobin levels less than 8 g/dL. Strikingly similar patterns have been reported from community cross-sectional and cohort studies conducted in areas of stable, perennial malaria transmission in Tanzania,11, 16 Kenya,17– 19 (Figure 2), and Malawi.20

Figure 1
Age patterns of anemia in Demographic and Health Surveys (DHS). a, mean hemoglobin (Hb) concentration (g/dL). b, prevalence of an Hb concentration less than 11 g/dL and 8 g/dL.
The Complex Etiology of Anemia
Anemia is usually multi-factorial in origin, and although malaria plays a key etiologic role in endemic countries, it is clear that poor nutritional status, micronutrient deficiencies, intestinal helminths, HIV infection, and hemoglobinopathies make important additional contributions. A number of factors account for the progressive fall in hemoglobin that is observed during the first year of life in areas of stable malaria transmission.
Antenatal factors
Placental malaria
Sequestration of malaria parasites in the placenta, a consequence of infection with Plasmodium falciparum during pregnancy, is associated with an increased risk of intrauterine growth retardation (IUGR), premature delivery, maternal and infant anemia, and infant mortality.19, 21– 23
Poor maternal nutrition and micronutrient deficiencies
Poor nutritional status in pregnancy has adverse consequences that can persist from one generation to the next, since women who are underweight or stunted are at risk of delivering premature or low birth weight infants, who are themselves at risk of poor growth and development and anemia in childhood and adolescence.24 Iron deficiency is a common cause of anemia in pregnant women in malaria-endemic areas,25– 28 and a recent study from Malawi demonstrated an absence of stainable iron in bone marrow aspirate, the most definitive method for determining iron status, in 44% of pregnant women with a hemoglobin levels less than < 10.5 g/dL.28 Multiple micronutrient deficiencies contribute to anemia in pregnancy, and deficiencies of vitamin A, folate, and vitamin B-12 were found in approximately 40%, 30%, and 25%, respectively, of pregnant Malawian women with anemia.28
Transfer of iron from the mother to the fetus is regulated by the placenta,29 with approximately two-thirds of fetal accretion occurring during the third trimester.30 A recent study from Zimbabwe has shown that maternal anemia and low birth weight are significant predictors of low total body iron (TBI) in infants, with the odds of subsequent anemia at 6, 9, and 12 months of age being more than three times higher ininfants in the lowest TBI quartile compared with those in the highest quartile.31 The combination of maternal iron deficiency and placental malaria therefore places infants born to pregnant women in malaria-endemic areas at particularly high risk of developing iron-deficiency anemia during the first year of life.
Human immunodeficiency virus
Infection with HIV in pregnancy is associated with an increased risk of IUGR, premature delivery, and anemia in the pregnant woman and her infant.19, 32– 36 There is increasing evidence of adverse interactions between malaria and HIV,37 which are likely to exacerbate the risk of anemia in the first year of life that arises from either factor independently. Human immunodeficiency virus increases the risk of placental malaria,35, 36 and recent evidence suggests that placental malaria may increase the risk of mother-to-child transmission of HIV,38 particularly if the density of placental malaria infection is high (Ayisi J. and others, unpublished data).
Intestinal helminths
Infection with hookworm and other intestinal helminths causes gastrointestinal blood loss, malabsorption, and inhibition of appetite, thereby exacerbating micronutrient deficiencies and maternal anemia. Intervention studies suggest that even relatively light hookworm infection in pregnancy may cause decreased fetal growth and weight gain.39
Postnatal factors
Malaria
Infants are vulnerable to malaria from the age of approximately three months, when immunity acquired from the mother is wearing off. Hospital series show that in areas of intense transmission, most cases of severe malarial anemia, blood transfusions, and deaths occur in infants40– 42 and children less than five years old.43– 45 Malaria causes anemia through hemolysis and increased splenic clearance of infected and uninfected red blood cells and cytokine-induced dyserythropoeisis.46– 48 A single overwhelming episode of malaria,49 or repeated episodes due to reinfection or failure to adequately clear parasitemia as a result of antimalarial drug resistance50 may result in life-threatening anemia, metabolic acidosis,51 and, if untreated, death. Severe anemia probably accounts for more than half of all childhood deaths from malaria in Africa,52 with case fatality rates in hospitals between 8% and 18%.40, 41, 45, 53– 55 Case fatality from severe anemia in the community is likely to be much higher, since the majority of hospital cases will have received a lifesaving blood transfusion.56
Human immunodeficiency virus
Infants infected with HIV and malaria are at particular risk of anemia during the first year of life. In a study from an area of high perennial malaria transmission in Western Kenya, a cohort of infants born to HIV-positive and HIV-negative mothers were monitored monthly from birth to one year of age.19 Mean hemoglobin levels were within the normal range for the first 12 weeks of life, but continued to decrease until 32 weeks of age, when they reached a nadir of 9.9 g/dL. The HIV-infected infants had lower mean hemoglobin levels and significantly more anemia during the first year of life than the uninfected infants. Figure 2 shows the effect of concurrent malaria by age, stratified by HIV status of the infant. Anemia was particularly common in HIV-infected infants with parasitemia at or after 16 weeks, and hemoglobin levels in these infants were significantly lower than those of HIV-uninfected infants or HIV-infected infants without parasitemia (P< 0.01).19 However, early detection and treatment of these infants with antimalarials and iron/folic acid failed to prevent anemia in the majority of cases.19 Anemia in these infants may be the result of cytokine-mediated inflammation, causing iron sequestration in macrophages, and decreased iron absorption in the small intestine (anemia of inflammation, previously known as anemia of chronic disease).57– 61
Intestinal helminths
The health consequences of chronic intestinal helminth infections, namely undernutrition, iron deficiency anemia, stunted growth, and impaired cognition,62, 63 are roughly proportional to the intensity of infection.64 School age children, who harbor the greatest number of worms, and pregnant women are therefore the main focus for helminth control programs.65, 66 In recent surveys, however, the prevalence of intestinal helminth infections in children less than 24 months old has ranged from 2% to 80%.67 In coastal east Africa, approximately one third of preschool children have hookworm infections, although the intensity of infection is relatively light.68, 69 In populations with a high prevalence of iron deficiency, even light infections may be sufficient to cause anemia.70
Poor child nutrition and micronutrient deficiencies
Of the more than 10 million deaths that occur each year in children less than five years old in developing countries, the majority are due to five conditions: malaria, HIV/acquired immunodeficiency virus (AIDS), acute respiratory infections, diarrhea, and measles, and more than half have been attributed directly or indirectly to malnutrition.71 Poor nutrition and micronutrient deficiencies may exacerbate the severity of any infectious disease,72, 73 and there is increasing evidence that they play an important role in the pathogenesis of malaria and malarial anemia.74– 76
Many African children live in a state of precarious iron balance. Relatively large amounts of iron are required for erythropoeisis in the first few months of life, and by the age of 4–6 months iron stores are marginal or depleted. Infants with a low TBI as a consequence of low birth weight or maternal iron deficiency31 are particularly prone to iron deficiency and anemia during this period, and early introduction of cereal-based weaning foods, from which iron absorption can be as low as 5%, may exacerbate the situation further. Iron demand may be further increased by chronic blood loss from the intestine, a result of intestinal helminth infections.
A close association between vitamin A deficiency and anemia has been demonstrated in many nutritional surveys, and a number of intervention studies have documented the impact of improved vitamin A status on hemoglobin levels and anemia.77 Vitamin A appears to protect against anemia through diverse biologic mechanisms, including the enhancement of the growth and differentiation of erythrocyte progenitor cells, modulation of immunity to infectious diseases, and mobilization of iron stores from tissues.77 Use of provitamin A carotenoids appears to be increased in children with severe malarial anemia.78
Zinc is required for normal immune function, and is essential for the production of interferon-γ, IgG, and tumor necrosis factor-α, all of which are involved in resistance to malaria.79 Cross-sectional studies in young children in Papua New Guinea and pregnant women in Malawi have demonstrated an association between low zinc status and P. falciparum parasitemia.79, 80
Folate is a central component of erythropoeisis, and hemolysis due to P. falciparum stimulates erythroid hyperplasia, making malaria a risk factor for folate deficiency.81
Riboflavin deficiency is widespread in populations consuming little milk or meat products, and a high prevalence of biochemical deficiency has been observed in studies from different parts of the developing world.74, 82 Riboflavin deficiency appears to protect against malaria,83, 84 and may impair iron mobilization, globin synthesis, and iron absoption.82
Increased production of reactive oxygen species85 during malaria infection in the presence of inadequate oxidative defense may damage the erythrocyte membrane and contribute towards anemia.86, 87 Alphatocopherol is the principal anti-oxidant in cell membranes, and reduced levels in erythrocyte membranes have been documented in children with malarial anemia.88 Vitamin C has antioxidant properties, and also facilitates the absorption and mobilization of iron.82 Evidence from in vitro and animal studies suggests that vitamin C deficiency may exacerbate malaria.74
Interventions to Prevent Anemia
Antimalarial interventions
Results obtained from malaria intervention studies provide compelling evidence that malaria contributes substantially to anemia in endemic regions. A recent review of 29 community-based studies of insecticide-treated nets (ITNs), antimalarial chemoprophylaxis, and insecticide residual spraying found that among children less than five years old exposed to between one and two years of malaria control, the mean relative risk for a hemoglobin level less than < 11 g/dL was 0.73 (95% confidence interval [CI] = 0.64–0.81) and the mean relative risk for a hemoglobin level less than < 8 g/dL was 0.40 (95% CI = 0.25–0.55) compared with control groups not exposed to malaria interventions (Korenromp E and others, unpublished data).
Insecticide-treated nets
A series of randomized controlled trials of ITNs conducted in areas of stable transmission in Africa has demonstrated that use of ITNs can reduce all-cause child mortality by approximately one-fifth, saving an average of 6 lives for every 1,000 children 1–59 months old protected each year.89 A recently published trial from a high transmission setting in western Kenya found that the protective efficacy of ITNs was highest in infants 1–11 months old compared with older children.90 The ITNs delayed the median time to first parasitemia from 4.5 to 10.7 months, and reduced the incidence of both clinical malaria and anemia by 60%, the reduction being greatest in infants 1–3 months of age.91 Infants sleeping under ITNs experienced better height and weight gain. The odds of a hemoglobin level less than < 9 g/dL increased with distance from the nearest netted village, indicating that persons not sleeping under ITNs, but living in the immediate vicinity of a netted village, also derived some benefit.92 When used by women during their first four pregnancies, ITNs reduced maternal parasitemia and placental parasitemia by 35%, and low birth weight by 28%.93 Mean hemoglobin levels were 0.6 g/dL higher in pregnant women sleeping under ITNs compared with the control group. At an annual cost (1996 rates) of US $25 per life-year gained, ITNs represent a highly cost-effective use of scarce health care resources.94 Despite these clear health benefits, ITN coverage is still poor in malaria-endemic countries of Africa, and surveys carried out between 1998 and 2001 indicate that 5% of pregnant women and less than 2% of children less than five years old were sleeping under ITNs.14, 95 The challenge is to now increase coverage, particularly among infants and pregnant women in areas of high transmission. This will require large-scale expansion of supply and distribution, strategies to reduce the price of ITNs, and the development of long-lasting insecticidal nets (factory pretreated nets that require no further treatment of their expected lifespan of 4–5 years).96
Chemoprophylaxis and intermittent preventive treatment
Pregnant women
The use of antimalarial drugs for chemoprophylaxis to prevent P. falciparum infection in pregnant women was first reported from Nigeria in 1964.97 Subsequent studies in several African countries have confirmed the beneficial impact of chemoprophylaxis on birth weight98 and maternal hemoglobin levels.99 On the basis of these trials, the World Health Organization (WHO) previously recommended that all pregnant women resident in areas of moderate or high malaria transmission be given chemoprophylaxis with chloroquine throughout the second and third trimesters of pregnancy. However, the effectiveness of this intervention has been seriously compromised by problems of compliance with a weekly drug regimen, the emergence of chloroquine-resistant P. falciparum malaria, and by concerns about increasing drug pressure from sub-therapeutic dosing. The WHO now recommends that intermittent preventive treatment (IPT), which provides similar benefits to chemoprophylaxis but reduces some of its risks, be given to pregnant women in areas of stable malaria.100
Use of IPT involves the administration of a full therapeutic dose of an antimalarial drug to pregnant women at specified intervals in the second and third trimesters, regardless of whether they are infected. Use of a single-dose drug such as sulfadoxine-pyrimethamine allows all doses to be given under direct observation in the antenatal clinic, and avoids the compliance problems associated with chemoprophylaxis. Presently, sulfadoxine-pyrimethamine is the only antimalarial for which data on efficacy and safety is available from controlled clinical trials. Studies in areas of Kenya and Malawi with low resistance to sulfadoxine-pyrimethamine have shown that IPT with sulfadoxine-pyrimethamine reduces maternal anemia (hemoglobin level less than < 8 g/dL),101 placental malaria,102 and low birth weight103 by approximately 40%. Sulfonamides and pyrimethamine are considered safe in the second and third trimesters of pregnancy.104 In areas of Africa where resistance to sulfadoxine-pyrimethamine is intensifying, alternative drugs for IPT in pregnancy require urgent evaluation. Since it is not known whether IPT achieves its effect primarily through clearance of parasites or through the long-acting prophylactic effect of sulfadoxine-pyrimethamine, there is also a need to evaluate antimalarials with shorter half-lives for use as IPT.105 At a cost of US $11 (1997 rates) for the prevention of each disability-adjusted life year due to low birth weight,106 IPT with intermittent sulfadoxine-pyrimethamine is one of the most cost-effective strategies for preventing morbidity and mortality associated with malaria.107 Although the proportion of women attending antenatal clinics who receive IPT varies from < 5% to > 70% in different countries,14, 95 experience from Malawi suggests that improved education on the benefits of IPT and modifications to the scheduling of antenatal clinic visits can markedly improve coverage.14
Infants
The efficacy of chemoprophylaxis in children was first reported in 1956 from a trial in The Gambia.108 Children who were given chloroquine weekly from birth until the age of two years had fewer episodes of malaria, better growth, and higher hemoglobin levels than the control group. In Liberia, monthly chloroquine given to children 2–9 years old reduced the number of episodes of clinical malaria by 50% and was associated with a significant improvement in hemoglobin levels.109 A study conducted in an area of intense transmission in southern Tanzania demonstrated a 60% reduction in episodes of clinical malaria and anemia in infants given weekly pyrimethamine plus dapsone between the ages of 2 and 10 months, although rates increased in the 11 month period after stopping chemoprophylaxis,110 raising the question as to whether IPT could have a beneficial effect on malaria and anemia without the rebound associated with weekly chemoprophylaxis.
A randomized controlled study from the same study site in Tanzania showed that a single dose of sulfadoxine-pyrimethamine given to asymptomatic infants attending for routine vaccination at two, three, and nine months of age reduced episodes of clinical malaria by 59% and episodes of anemia by 50% during the first year of life.111 Similar results were obtained from a study conducted in northern Tanzania using amodiaquine.112 Use of IPT in infants (IPTi) is a particularly attractive strategy, since sustainable delivery may be achieved through the Expanded Program on Immunization (EPI), but a number of important questions need to be addressed before it can be considered for inclusion in national malaria control policies. Will it work in other epidemiologic settings? Is it safe? Might it have an adverse impact on serologic responses to EPI vaccines or on the development of malarial immunity? Is it operationally feasible and cost-effective? The IPTi Consortium, comprising a number of research groups in Africa, Europe, and the United States, together with WHO and the United Nations Children’s Fund, has been established to ensure that these issues are addressed in a systematic and timely manner, and has received support from the Bill and Melinda Gates Foundation.
Prompt, effective treatment of malaria infections
Prompt treatment of malaria infections with effective, fast-acting antimalarial drugs rapidly reduces symptomatic high density parasitemia and clears parasites from the blood, allowing erythrocyte numbers to be restored49, 50, 113 and reducing the risk of anemia. The recent spread of antimalarial drug resistance, which has reduced drug efficacy and increased recrudescent parasitemia and anemia,114, 115 is likely to have contributed to the increase in malaria-specific mortality that has been observed in African children over the last decade.116, 117 The rapid action and anti-gametocyte properties of the artemisinin derivatives make them a particularly promising treatment option, and WHO strongly recommends that malaria-endemic countries changing antimalarial drug policy because of increasing drug resistance consider adopting artemisinin-based combination therapy as a first-line treatment for P. falciparum malaria.118 The high cure rates achieved with these combinations119 and the prospect of sustained efficacy is likely to markedly reduce anemia due to parasite recrudescence.
Intestinal helminths
There is increasing evidence that very young children may benefit from de-worming.69, 120 In a recent study from Zanzibar, the prevalence of moderate anemia (hemoglobin level < 9 g/dL) and wasting (weight-for-height <-1 Z-score and mid upper arm circumference < 5th centile) was significantly reduced in children less than 24 months old with light worm infections who had been treated with mebendazole every three months for a year.69 In the same study, low dose daily iron supplementation improved iron status and appetite, but had no impact on anemia. First-time helminth infections at this age may induce proinflammatory mediators that are detrimental to protein metabolism, appetite, and erythropoiesis.121, 122 Mebendazole and albendazole can be safely used in young children,67 and the WHO now recommends that in areas with a high prevalence of intestinal helminths, de-worming three times per year should start from the age of 12 months.123
Human immunodeficiency virus
Endogenous release of proinflammatory cytokines (interferon-γ, tumor necrosis factor-α, interleukin-6) and altered iron metabolism are thought to contribute to anemia in HIV-infected individuals.57, 59, 124 Increasing use of antiretroviral treatment to prevent mother to child transmission of HIV125, 126 may reduce the prevalence of anemia in infants in populations in which HIV seroprevalence is high.
Micronutrient deficiencies
Iron
The role of iron in the prevention and treatment of anemia in malaria-endemic regions remains a highly contentious issue. Iron is a key functional component of a wide range of biologic systems, and is therefore an essential element for nearly all living organisms.127 Excessive iron can, however, cause tissue damage, since it has the ability to catalyze the generation of reactive free radicals. Regulation of iron metabolism within the body is therefore kept under tight homeostatic control.128
Anemia can result from the alterations in iron metabolism that occur as a response to many infectious diseases. Infections and inflammatory diseases decrease iron absorption in the small intestine, and induce iron sequestration in macrophages, the hallmark of anemia of inflammation. It is assumed that the iron sequestration response may increase resistance to infections by restricting the availability of iron to microbes.60 In the past three years, enormous progress in the understanding of this process has been made by the discovery of hepcidin, an iron-regulatory peptide made by hepatocytes.61 Exposure of hepatic Kupffer cells to microbes causes the release of interleukin-6, and possibly other cytokines, which induces the synthesis and secretion of hepcidin.129 Plasma hepcidin inhibits iron uptake in the duodenum and iron release from macrophages in the spleen and elsewhere. The continuing debate on the role of iron in environments where there is a high degree of exposure to infectious diseases (malaria, HIV, bacterial pathogens) is therefore fuelled by the following paradox: although iron deficiency adversely affects growth, immune function, and cognitive development and can cause anemia, the administration of iron for the treatment of anemia may exacerbate infectious disease.
A number of recent reviews have assessed the impact of iron, administered during randomized controlled trials to prevent or to treat anemia, on malaria and other infectious diseases.8, 130, 131 Administration of iron has a beneficial, although variable, impact on hemoglobin levels, but appears to increase the risk of diarrhea (incidence rate ratio = 1.11 [95% CI = 1.01-1.23, P= 0.04]).131 The risk of malaria parasitemia is not increased by iron supplementation, once baseline parasitemia is taken into account.130, 131 However, the clinical significance of these findings is unclear since the component studies were not designed and not powered to assess the impact of iron on morbidity and mortality from malaria.
What practical conclusions can be derived from these findings? Iron deficiency is highly prevalent in many malaria-endemic regions of Africa, and it is clear that many infants, particularly those born prematurely or with low birth weight, have low total body iron, and are at particular risk of iron deficiency during the first six months of life. Malaria and other infectious diseases have an adverse impact on hemoglobin levels from the age of approximately three months, and the prevalence of all grades of anemia is highest in the second half of infancy. A short period of iron supplementation in the first few months of life might replenish iron stores at a time when there is less pressure from infectious diseases such as malaria. Among a group of 411 Tanzanian infants who received iron supplementation or placebo between the ages of two and six months as part of a trial to prevent malaria and anemia, and who were followed-up over a period of four years, there was no increase in clinical malaria or outpatient attendance among the iron-supplemented infants (Menendez C, unpublished data). There was a 29% reduction in anemia (packed cell volume < 25%) at the age of one year in infants who had received iron in early infancy,110 and the cumulative risk of anemia in this group over the four-year period of follow-up was reduced by 18%. An advantage of this strategy is that iron supplementation may be delivered though the EPI,111 which enhances sustainability and cost-effectiveness.132 Concurrent delivery of ITNs and, in the future, IPTi, would reduce exposure to malaria during and after the period of iron administration.
Other micronutrients
Although there is a considerable body of experimental evidence to suggest that micronutrient deficiencies may play an important role in the pathogenesis of malaria and malarial anemia, the complex pathways through which micronutrients may influence malaria parasites and host morbidity are poorly understood.74 A limited number of trials have assessed the impact of micronutrient supplementation on malaria and malarial anemia.
Vitamin A supplementation reduced episodes of clinical malaria in children in Papua New Guinea,133 although there was no impact on anemia at cross-sectional survey. No impact on clinical malaria was observed in trials of vitamin A supplementation in Ghana and Tanzania.134, 135 However, vitamin A supplementation has been shown to reduce child mortality,136 and routine supplementation of pregnant and postpartum women and young children is now recommended.137
Although zinc supplementation reduces episodes of diarrhea and pneumonia in young children, the three studies that have assessed the impact of zinc supplementation on the prevention of malaria have yielded conflicting results. A study from Papua New Guinea138 reported a 38% reduction in episodes of clinical malaria in preschool children given a daily zinc supplement for 11 months, but episodes of clinical malaria were not significantly reduced by zinc supplementation in preschool children in The Gambia139 and Burkina Faso.140
The development of innovative ways of increasing the sustainable delivery of individual and multiple micronutrients is of considerable current interest. Food fortification,141 the use of complementary food supplements (micronutrient sprinkles, fortified spreads),142 and the breeding of crops with an increased content of specific micronutrients143 are strategies that appear particularly promising. Increased communication and collaboration between the nutrition, agriculture, and development sectors is an essential prerequisite of improving dietary quality for poor populations.144
Putting It All Together: The Need for Anemia Prevention Programs
Despite the magnitude of the anemia problem, and the constantly expanding body of research findings relating to pathogenesis, risk factors, and efficacious interventions, coverage of interventions to prevent anemia in malaria-endemic countries remains poor.14 This is due largely to the fact that all of the currently available interventions against anemia fail to fulfill one or more of the following criteria that help to determine whether a health care strategy is successfully introduced at country level. First, there must be convincing data relating to efficacy and safety. A balance must be struck between the need for further research, and the timely development of clear policy recommendations. The translation of research into policy should be an iterative process, enabling policy guidelines to be modified in relation to relevant new research findings.145 Second, an intervention must be cost-effective and affordable. “Affordability” is a function of political and financial commitment on the part of national governments and donor agencies. Third, it must be readily available at health facilities, which requires detailed planning and functional health systems, and, lastly, it should be delivered through systems that are sustainable in the long term. The complex, multifactorial nature of anemia in malaria-endemic regions of Africa means that it is best tackled by means of an integrated, non-disease-specific approach. This approach is more likely to be successful if interventions are targeted at the groups at highest risk of anemia, namely pregnant women and their infants, and if sustainable systems, namely antenatal clinics and the EPI, are used for their delivery. National survey data from 28 African countries indicate that in general, antenatal clinic attendance exceeds 70%.14 The EPI coverage, although variable, reaches 60% or more in the majority of African countries. Both systems could be used for the delivery of ITNs, IPT, pre-packaged antimalarial drugs for emergency use at home, de-worming, micronutrient supplementation, dietary advice, and, potentially, antiretroviral therapy. Although it is clearly important to avoid overloading existing systems, this approach might have the advantage of increasing attendance at these routine points of contact with health services. The substantial increase in financial and human resources that will be necessary to achieve the current goal of rapidly increasing access to antiretroviral drugs in Africa146 should be viewed as an excellent opportunity for improving the prevention and treatment of anemia, and for strengthening maternal and child health services. Establishing strong linkages between relevant programs (Maternal and Child Health, EPI, Roll Back Malaria, HIV/AIDS, and others) at national, regional, and international level will be an important part of this process.
Although prevention is of utmost importance, there are additional challenges that must be addressed. More emphasis needs to be placed on increasing awareness of anemia in the community and by health care workers, since mild and moderate degrees of anemia may, if unrecognized and untreated,10, 11 progress to severe, life-threatening anemia. Efforts should be made to ensure that laboratories at district hospital level use an accurate, user-friendly, and, ideally, inexpensive method for measuring hemoglobin, since blood transfusions that are prescribed on the basis of faulty hemoglobin measurement12 expose the patient unnecessarily to the risk of HIV and other blood-borne diseases.13 Hospital clinicians need to be trained to recognize the clinical features that are associated with increased mortality from severe anemia,147 and there is a need to develop transfusion guidelines that are based on clinical criteria, and not solely on the level of hemoglobin.148 Although blood transfusion for severe anemia can be life-saving,56 the optimal speed and volume of transfusion remain to be determined.51 The spread of HIV infection increases the urgency of exploring alternatives to blood for the treatment of severe anemia, and the recent development of modified hemoglobin blood substitutes149 is therefore timely.
Conclusions
It is clear that an integrated, non disease-specific approach is essential if the intolerable burden of anemia that currently exists in malaria-endemic regions of Africa is going to be reduced. It will be important to involve health programs as diverse as malaria, nutrition, reproductive and child health, HIV/AIDS, helminth control, and laboratory and blood transfusion services. There needs to be communication and collaboration with other disciplines, particularly environmental health and agriculture.144, 150 Research institutions, nongovernmental organizations and the media have important roles to play. Political will is essential: ministers of health and finance need to understand that anemia control is cost-effective and yields substantial health benefits.
References
- 1.
- DeMaeyer E, Adiels-Tegman M. The prevalence of anaemia in the world. World Health Stat Q. 1985;38:302–316. [PubMed: 3878044]
- 2.
- Administrative Committee on Coordination/Sub-Committee on Nutrition (ACC/SCN), 2000. Fourth Report on the World Nutrition Situation. Geneva: World Health Organization. ACC/SCN in Collaboration with the International Food Policy Research Institute.
- 3.
- Brabin BJ, Premji Z, Verhoeff F. An analysis of anemia and child mortality. J Nutr. 2001;131(2S-2):636S–645S. [PubMed: 11160595]
- 4.
- Lozoff B, Jimenez E, Hagen J, Mollen E, Wolf AW. Poorer behavioral and developmental outcome more than 10 years after treatment for iron deficiency in infancy. Pediatrics. 2000;105:E51. [PubMed: 10742372]
- 5.
- Grantham-McGregor S, Ani C. A review of studies on the effect of iron deficiency on cognitive development in children. J Nutr. 2001;131:649S–666S. [PubMed: 11160596]
- 6.
- Sherriff A, Emond A, Bell JC, Golding J. Should infants be screened for anaemia? A prospective study investigating the relation between haemoglobin at 8, 12, and 18 months and development at 18 months. Arch Dis Child. 2001;84:480–485. [PMC free article: PMC1718808] [PubMed: 11369562]
- 7.
- Lawless JW, Latham MC, Stephenson LS, Kinoti SN, Pertet AM. Iron supplementation improves appetite and growth in anemic Kenyan primary school children. J Nutr. 1994;124:645–654. [PubMed: 8169656]
- 8.
- Oppenheimer SJ. Iron and its relation to immunity and infectious disease. J Nutr. 2001;131:616S–633S. [PubMed: 11160594]
- 9.
- Haas JD, Brownlie TT. Iron deficiency and reduced work capacity: a critical review of the research to determine a causal relationship. J Nutr. 2001;131:676S–688S. [PubMed: 11160598]
- 10.
- Phillips-Howard PA, Wannemuehler KA, ter Kuile FO, Hawley WA, Kolczak MS, Odhacha A, Vulule JM, Nahlen BL. Diagnostic and prescribing practices in peripheral health facilities in rural western Kenya. Am J Trop Med Hyg. 2003;68(Suppl 4):44–49. [PubMed: 12749485]
- 11.
- Schellenberg D, Schellenberg JR, Mushi A, Savigny D, Mgalula L, Mbuya C, Victora CG. The silent burden of anaemia in Tanzanian children: a community-based study. Bull World Health Organ. 2003;81:581–590. [PMC free article: PMC2572520] [PubMed: 14576890]
- 12.
- Bates I, Mundy C, Pendame R, Kadewele G, Gilks C, Squire S. Use of clinical judgement to guide administration of blood transfusions in Malawi. Trans R Soc Trop Med Hyg. 2001;95:510–512. [PubMed: 11706662]
- 13.
- Lackritz EM. Prevention of HIV transmission by blood transfusion in the developing world: achievements and continuing challenges. AIDS. 1998;12(Suppl A):S81–S86. [PubMed: 9632988]
- 14.
- WHO, 2003. The Africa Malaria Report. Geneva: World Health Organization.
- 15.
- Mapping Malaria Risk in Africa/Atlas du Risque de la Malaria en Afrique, 1995. Modelled Maps of Malaria Transmission in West Africa.
- 16.
- Kitua AY, Smith TA, Alonso PL, Urassa H, Masanja H, Kimario J, Tanner M. The role of low level Plasmodium falciparum parasitemia in anaemia among infants living in an area of intense and perennial transmission. Trop Med Int Health. 1997;2:325–333. [PubMed: 9171840]
- 17.
- Bloland PB, Ruebush TK, McCormick JB, Ayisi J, Boriga DA, Oloo AJ, Beach R, Hawley W, Lal A, Nahlen B, Udhayakumar V, Campbell CC. Longitudinal cohort study of the epidemiology of malaria infections in an area of intense malaria transmission I. Description of study site, general methodology, and study population. Am J Trop Med Hyg. 1999;60:635–640. [PubMed: 10348240]
- 18.
- McElroy PD, ter Kuile FO, Lal AA, Bloland PB, Hawley WA, Oloo AJ, Monto AS, Meshnick SR, Nahlen BL. Effect of Plasmodium falciparum parasitemia density on hemoglobin concentrations among full-term, normal birth weight children in western Kenya, IV. The Asembo Bay Cohort Project. Am J Trop Med Hyg. 2000;62:504–512. [PubMed: 11220768]
- 19.
- van Eijk AM, Ayisi JG, ter Kuile FO, Misore AO, Otieno JA, Kolczak MS, Kager PA, Steketee RW, Nahlen BL. Malaria and human immunodeficiency virus infection as risk factors for anemia in infants in Kisumu, western Kenya. Am J Trop Med Hyg. 2002;67:44–53. [PubMed: 12363063]
- 20.
- le Cessie S, Verhoeff FH, Mengistie G, Kazembe P, Broadhead R, Brabin BJ. Changes in haemoglobin levels in infants in Malawi: effect of low birth weight and fetal anaemia. Arch Dis Child Fetal Neonatal Ed. 2002;86:F182–F187. [PMC free article: PMC1721412] [PubMed: 11978749]
- 21.
- Reed SC, Wirima JJ, Steketee RW. Risk factors for anemia in young children in rural Malawi. Am J Trop Med Hyg. 1994;51:170–174. [PubMed: 8074250]
- 22.
- Menendez C, Ordi J, Ismail MR, Ventura PJ, Aponte JJ, Kahigwa E, Font F, Alonso PL. The impact of placental malaria on gestational age and birth weight. J Infect Dis. 2000;181:1740–1745. [PubMed: 10823776]
- 23.
- Steketee RW, Nahlen BL, Parise ME, Menendez C. The burden of malaria in pregnancy in malaria-endemic areas. Am J Trop Med Hyg. 2001;64(Suppl 12):28–35. [PubMed: 11425175]
- 24.
- Steketee RW. Pregnancy, nutrition and parasitic diseases. J Nutr. 2003;133(Suppl 2):1661S–1667S. [PubMed: 12730482]
- 25.
- Massawe SN, Urassa EN, Mmari M, Ronquist G, Lindmark G, Nystrom L. The complexity of pregnancy anemia in Dares-Salaam. Gynecol Obstet Invest. 1999;47:76–82. [PubMed: 9949275]
- 26.
- Verhoeff FH, Brabin BJ, Chimsuku L, Kazembe P, Broadhead RL. An analysis of the determinants of anaemia in pregnant women in rural Malawia basis for action. Ann Trop Med Parasitol. 1999;93:119–133. [PubMed: 10474637]
- 27.
- May J, Falusi AG, Mockenhaupt FP, Ademowo OG, Olumese PE, Bienzle U, Meyer CG. Impact of subpatent multi-species and multi-clonal plasmodial infections on anaemia in children from Nigeria. Trans R Soc Trop Med Hyg. 2000;94:399–403. [PubMed: 11127243]
- 28.
- van den Broek NR, Letsky EA. Etiology of anemia in pregnancy in south Malawi. Am J Clin Nutr. 2000;72(Suppl 1):247S–256S. [PubMed: 10871590]
- 29.
- Harris ED. New insights into placental iron transport. Nutr Rev. 1992;50:329–331. [PubMed: 1488157]
- 30.
- Singla PN, Gupta VK, Agarwal KN. Storage iron in human foetal organs. Acta Paediatr Scand. 1985;74:701–706. [PubMed: 4050416]
- 31.
- Miller MF, Stoltzfus RJ, Mbuya NV, Malaba LC, Iliff PJ, Humphrey JH. Total body iron in HIV-positive and HIV-negative Zimbabwean newborns strongly predicts anemia throughout infancy and is predicted by maternal hemoglobin concentration. J Nutr. 2003;133:3461–3468. [PubMed: 14608059]
- 32.
- Antelman G, Msamanga GI, Spiegelman D, Urassa EJ, Narh R, Hunter DJ, Fawzi WW. Nutritional factors and infectious disease contribute to anemia among pregnant women with human immunodeficiency virus in Tanzania. J Nutr. 2000;130:1950–1957. [PubMed: 10917907]
- 33.
- Dreyfuss ML, Msamanga GI, Spiegelman D, Hunter DJ, Urassa EJ, Hertzmark E, Fawzi WW. Determinants of low birth weight among HIV-infected pregnant women in Tanzania. Am J Clin Nutr. 2001;74:814–826. [PubMed: 11722965]
- 34.
- van Eijk AM, Ayisi JG, ter Kuile FO, Misore A, Otieno JA, Kolczak MS, Kager PA, Steketee RW, Nahlen BL. Human immunodeficiency virus seropositivity and malaria as risk factors for third-trimester anemia in asymptomatic pregnant women in western Kenya. Am J Trop Med Hyg. 2001;65:623–630. [PubMed: 11716125]
- 35.
- Ayisi JG, van Eijk AM, ter Kuile FO, Kolczak MS, Otieno JA, Misore AO, Kager PA, Steketee RW, Nahlen BL. The effect of dual infection with HIV and malaria on pregnancy outcome in western Kenya. AIDS. 2003;17:585–594. [PubMed: 12598779]
- 36.
- Ticconi C, Mapfumo M, Dorrucci M, Naha N, Tarira E, Pietropolli A, Rezza G. Effect of maternal HIV and malaria infection on pregnancy and perinatal outcome in Zimbabwe. J Acquir Immune Defic Syndr. 2003;34:289–294. [PubMed: 14600573]
- 37.
- Rowland-Jones SL, Lohman B. Interactions between malaria and HIV infection-an emerging public health problem? Microbes Infect. 2002;4:1265–1270. [PubMed: 12467769]
- 38.
- Brahmbhatt H, Kigozi G, Wabwire-Mangen F, Serwadda D, Sewankambo N, Lutalo T, Wawer MJ, Abramowsky C, Sullivan D, Gray R. The effects of placental malaria on mother-to-child HIV transmission in Rakai, Uganda. AIDS. 2003;17:2539–2541. [PubMed: 14600529]
- 39.
- Torlesse H, Hodges M. Albendazole therapy and reduced decline in haemoglobin concentration during pregnancy (Sierra Leone). Trans R Soc Trop Med Hyg. 2001;95:195–201. [PubMed: 11355560]
- 40.
- Slutsker L, Taylor TE, Wirima JJ, Steketee RW. Inhospital morbidity and mortality due to malaria-associated severe anaemia in two areas of Malawi with different patterns of malaria infection. Trans R Soc Trop Med Hyg. 1994;88:548–551. [PubMed: 7992335]
- 41.
- Schellenberg D, Menendez C, Kahigwa E, Font F, Galindo C, Acosta C, Schellenberg JA, Aponte JJ, Kimario J, Urassa H, Mshinda H, Tanner M, Alonso P. African children with malaria in an area of intense Plasmodium falciparum transmission: features on admission to the hospital and risk factors for death. Am J Trop Med Hyg. 1999;61:431–438. [PubMed: 10497986]
- 42.
- Kahigwa E, Schellenberg D, Sanz S, Aponte JJ, Wigayi J, Mshinda H, Alonso P, Menendez C. Risk factors for presentation to hospital with severe anaemia in Tanzanian children: a case-control study. Trop Med Int Health. 2002;7:823–830. [PubMed: 12358616]
- 43.
- Bojang KA, Van Hensbroek MB, Palmer A, Banya WA, Jaffar S, Greenwood BM. Predictors of mortality in Gambian children with severe malaria anaemia. Ann Trop Paediatr. 1997;17:355–359. [PubMed: 9578796]
- 44.
- Newton CR, Warn PA, Winstanley PA, Peshu N, Snow RW, Pasvol G, Marsh K. Severe anaemia in children living in a malaria endemic area of Kenya. Trop Med Int Health. 1997;2:165–178. [PubMed: 9472302]
- 45.
- Biemba G, Dolmans D, Thuma PE, Weiss G, Gordeuk VR. Severe anaemia in Zambian children with Plasmodium falciparum malaria. Trop Med Int Health. 2000;5:9–16. [PubMed: 10672200]
- 46.
- Menendez C, Fleming AF, Alonso PL. Malaria-related anaemia. Parasitol Today. 2000;16:469–476. [PubMed: 11063857]
- 47.
- Nagel RL. Malarial anemia. Hemoglobin. 2002;26:329–343. [PubMed: 12484628]
- 48.
- Ekvall H. Malaria and anemia. Curr Opin Hematol. 2003;10:108–114. [PubMed: 12579035]
- 49.
- Ekvall H, Arese P, Turrini F, Ayi K, Mannu F, Premji Z, Bjorkman A. Acute haemolysis in childhood falciparum malaria. Trans R Soc Trop Med Hyg. 2001;95:611–617. [PubMed: 11816432]
- 50.
- Bjorkman A. Malaria associated anaemia, drug resistance and antimalarial combination therapy. Int J Parasitol. 2002;32:1637–1643. [PubMed: 12435448]
- 51.
- English M. Life-threatening severe malarial anaemia. Trans R Soc Trop Med Hyg. 2000;94:585–588. [PubMed: 11198634]
- 52.
- Murphy SC, Breman JG. Gaps in the childhood malaria burden in Africa: cerebral malaria, neurological sequelae, anemia, respiratory distress, hypoglycemia, and complications of pregnancy. Am J Trop Med Hyg. 2001;64(Suppl 12):57–67. [PubMed: 11425178]
- 53.
- Lackritz EM, Campbell CC, Ruebush TK II,, Hightower AW, Wakube W, Steketee RW, Were JB. Effect of blood transfusion on survival among children in a Kenyan hospital. Lancet. 1992;340:524–528. [PubMed: 1354285]
- 54.
- Marsh K, Forster D, Waruiru C, Mwangi I, Winstanley M, Marsh V, Newton C, Winstanley P, Warn P, Peshu N. Indicators of life-threatening malaria in African children. N Engl J Med. 1995;332:1399–1404. [PubMed: 7723795]
- 55.
- Bojang KA, Palmer A, Boele van Hensbroek M, Banya WA, Greenwood BM. Management of severe malarial anaemia in Gambian children. Trans R Soc Trop Med Hyg. 1997;91:557–561. [PubMed: 9463667]
- 56.
- English M, Ahmed M, Ngando C, Berkley J, Ross A. Blood transfusion for severe anaemia in children in a Kenyan hospital. Lancet. 2002;359:494–495. [PubMed: 11853798]
- 57.
- Fuchs D, Zangerle R, Artner-Dworzak E, Weiss G, Fritsch P, Tilz GP, Dierich MP, Wachter H. Association between immune activation, changes of iron metabolism and anaemia in patients with HIV infection. Eur J Haematol. 1993;50:90–94. [PubMed: 8440363]
- 58.
- Cairo G, Pietrangelo A. Nitric-oxide-mediated activation of iron-regulatory protein controls hepatic iron metabolism during acute inflammation. Eur J Biochem. 1995;232:358–363. [PubMed: 7556182]
- 59.
- Kreuzer KA, Rockstroh JK, Jelkmann W, Theisen A, Spengler U, Sauerbruch T. Inadequate erythropoietin response to anaemia in HIV patients: relationship to serum levels of tumour necrosis factor-alpha, interleukin-6 and their soluble receptors. Br J Haematol. 1997;96:235–239. [PubMed: 9029005]
- 60.
- Jurado RL. Iron, infections, and anemia of inflammation. Clin Infect Dis. 1997;25:888–895. [PubMed: 9356804]
- 61.
- Ganz T. Hepcidin, a key regulator of iron metabolism and mediator of anemia of inflammation. Blood. 2003;102:783–788. [PubMed: 12663437]
- 62.
- Simeon D, Callender J, Wong M, Grantham-McGregor S, Ramdath DD. School performance, nutritional status and tri-churiasis in Jamaican schoolchildren. Acta Paediatr. 1994;83:1188–1193. [PubMed: 7531045]
- 63.
- Stoltzfus RJ, Dreyfuss ML, Chwaya HM, Albonico M. Hookworm control as a strategy to prevent iron deficiency. Nutr Rev. 1997;55:223–232. [PubMed: 9279058]
- 64.
- Savioli L, Bundy D, Tomkins A. Intestinal parasitic infections: a soluble public health problem. Trans R Soc Trop Med Hyg. 1992;86:353–354. [PubMed: 1440799]
- 65.
- World Health Organization, 1994. Report of the WHO Informal Consultation on Hookworm Infection and Anaemia in Girls and Women. Geneva: World Health Organization.
- 66.
- World Health Organization, 1996. Report of the WHO Informal Consultation on the Use of Chemotherapy for the Control of Morbidity Due to Soil-Transmitted Nematodes in Humans. Geneva: World Health Organization.
- 67.
- Montresor A, Awasthi S, Crompton DW. Use of benzimidazoles in children younger than 24 months for the treatment of soil-transmitted helminthiasis. Acta Trop. 2003;86:223–232. [PMC free article: PMC5633076] [PubMed: 12745139]
- 68.
- Brooker S, Peshu N, Warn PA, Mosobo M, Guyatt HL, Marsh K, Snow RW. The epidemiology of hookworm infection and its contribution to anaemia among pre-school children on the Kenyan coast. Trans R Soc Trop Med Hyg. 1999;93:240–246. [PubMed: 10492749]
- 69.
- Stoltzfus RJ, Chway HM, Montresor A, Tielsch JM, Jape JK, Albonico M, Savioli L. Low dose daily iron supplementation improves iron status and appetite but not anemia, whereas quarterly anthelminthic treatment improves growth, appetite and anemia in Zanzibari preschool children. J Nutr. 2004;134:348–356. [PubMed: 14747671]
- 70.
- Albonico M, Stoltzfus RJ, Savioli L, Tielsch JM, Chwaya HM, Ercole E, Cancrini G. Epidemiological evidence for a differential effect of hookworm species, Ancylostoma duodenale or Necator americanus, on iron status of children. Int J Epidemiol. 1998;27:530–537. [PubMed: 9698148]
- 71.
- Black RE, Morris SS, Bryce J. Where and why are 10 million children dying every year? Lancet. 2003;361:2226–2234. [PubMed: 12842379]
- 72.
- Tomkins A, 1988. The risk of morbidity in a stunted child. Waterlow JC, ed. Linear Growth Reduction in Less Developed Countries. New York: Raven Press, 185–199.
- 73.
- Scrimshaw NS, SanGiovanni JP. Synergism of nutrition, infection, and immunity: an overview. Am J Clin Nutr. 1997;66:464S–477S. [PubMed: 9250134]
- 74.
- Shankar AH. Nutritional modulation of malaria morbidity and mortality. J Infect Dis. 2000;182(Suppl 1):S37–S53. [PubMed: 10944483]
- 75.
- Nussenblatt V, Semba RD. Micronutrient malnutrition and the pathogenesis of malarial anemia. Acta Trop. 2002;82:321–337. [PubMed: 12039672]
- 76.
- Verhoef H, West CE, Veenemans J, Beguin Y, Kok FJ. Stunting may determine the severity of malaria-associated anemia in African children. Pediatrics. 2002;110:e48. [PubMed: 12359821]
- 77.
- Semba RD, Bloem MW. The anemia of vitamin A deficiency: epidemiology and pathogenesis. Eur J Clin Nutr. 2002;56:271–281. [PubMed: 11965502]
- 78.
- Nussenblatt V, Mukasa G, Metzger A, Ndeezi G, Eisinger W, Semba RD. Relationship between carotenoids and anaemia during acute uncomplicated Plasmodium falciparum malaria in children. J Health Popul Nutr. 2002;20:205–214. [PubMed: 12430756]
- 79.
- Shankar AH, Prasad AS. Zinc and immune function: the biological basis of altered resistance to infection. Am J Clin Nutr. 1998;68(Suppl 2):447S–463S. [PubMed: 9701160]
- 80.
- Gibson RS, Huddle JM. Suboptimal zinc status in pregnant Malawian women: its association with low intakes of poorly available zinc, frequent reproductive cycling, and malaria. Am J Clin Nutr. 1998;67:702–709. [PubMed: 9537617]
- 81.
- Strickland GT, Kostinas JE. Folic acid deficiency complicating malaria. Am J Trop Med Hyg. 1970;19:910–915. [PubMed: 4923968]
- 82.
- Fishman SM, Christian P, West KP. The role of vitamins in the prevention and control of anaemia. Public Health Nutr. 2000;3:125–150. [PubMed: 10948381]
- 83.
- Thurnham DI, Oppenheimer SJ, Bull R. Riboflavin status and malaria in infants in Papua New Guinea. Trans R Soc Trop Med Hyg. 1983;77:423–424. [PubMed: 6353691]
- 84.
- Thurnham DI. Antimalarial effects of riboflavin deficiency. Lancet. 1985;2:1310–1311. [PubMed: 2866378]
- 85.
- Greve B, Kremsner PG, Lell B, Luckner D, Schmid D. Malarial anaemia in African children associated with high oxygen-radical production. Lancet. 2000;355:40–41. [PubMed: 10615892]
- 86.
- Kay MM, Bosman GJ, Shapiro SS, Bendich A, Bassel PS. Oxidation as a possible mechanism of cellular aging: vitamin E deficiency causes premature aging and IgG binding to erythrocytes. Proc Natl Acad Sci USA. 1986;83:2463–2467. [PMC free article: PMC323318] [PubMed: 3458208]
- 87.
- Turi S, Nemeth I, Vargha I, Matkovics B. Oxidative damage of red blood cells in haemolytic uraemic syndrome. Pediatr Nephrol. 1994;8:26–29. [PubMed: 8142220]
- 88.
- Griffiths MJ, Ndungu F, Baird KL, Muller DP, Marsh K, Newton CR. Oxidative stress and erythrocyte damage in Kenyan children with severe Plasmodium falciparum malaria. Br J Haematol. 2001;113:486–491. [PubMed: 11380421]
- 89.
- Lengeler C, 2002. Insecticide-treated bednets and curtains for preventing malaria (Cochrane Review). The Cochrane Library. Oxford: Update Software. [PubMed: 10796535]
- 90.
- Phillips-Howard PA, Nahlen BL, Kolczak MS, Hightower AW, ter Kuile FO, Alaii JA, Gimnig JE, Arudo J, Vulule JM, Odhacha A, Kachur SP, Schoute E, Rosen DH, Sexton JD, Oloo AJ, Hawley WA. Efficacy of permethrin-treated bed nets in the prevention of mortality in young children in an area of high perennial malaria transmission in western Kenya. Am J Trop Med Hyg. 2003;68(Suppl 4):23–29. [PubMed: 12749482]
- 91.
- ter Kuile FO, Terlouw DJ, Kariuki SK, Phillips-Howard PA, Mirel LB, Hawley WA, Friedman JF, Shi YP, Kolczak MS, Lal AA, Vulule JM, Nahlen BL. Impact of permethrin-treated bed nets on malaria, anemia, and growth in infants in an area of intense perennial malaria transmission in western Kenya. Am J Trop Med Hyg. 2003;68(Suppl 4):68–77. [PubMed: 12749488]
- 92.
- Hawley WA, Phillips-Howard PA, ter Kuile FO, Terlouw DJ, Vulule JM, Ombok M, Nahlen BL, Gimnig JE, Kariuki SK, Kolczak MS, Hightower AW. Community-wide effects of permethrin-treated bed nets on child mortality and malaria morbidity in western Kenya. Am J Trop Med Hyg. 2003;68(Suppl 4):121–127. [PubMed: 12749495]
- 93.
- ter Kuile FO, Terlouw DJ, Phillips-Howard PA, Hawley WA, Friedman JF, Kariuki SK, Shi YP, Kolczak MS, Lal AA, Vulule JM, Nahlen BL. Reduction of malaria during pregnancy by permethrin-treated bed nets in an area of intense perennial malaria transmission in western Kenya. Am J Trop Med Hyg. 2003;68(Suppl 4):50–60. [PubMed: 12749486]
- 94.
- Wiseman V, Hawley WA, ter Kuile FO, Phillips-Howard PA, Vulule JM, Nahlen BL, Mills AJ. The cost-effectiveness of permethrin-treated bed nets in an area of intense malaria transmission in western Kenya. Am J Trop Med Hyg. 2003;68(Suppl 4):161–167. [PubMed: 12749500]
- 95.
- Guyatt HL, Noor AM, Ochola SA, Snow RW. Use of intermittent presumptive treatment and insecticide treated nets by pregnant women in four Kenyan districts. Trop Med Int Health. 2004;9:255–261. [PubMed: 15040563]
- 96.
- Guillet P, Alnwick D, Cham MK, Neira M, Zaim M, Heymann D, Mukelabai K. Long-lasting treated mosquito nets: a breakthrough in malaria prevention. Bull World Health Organ. 2001;79:998. [PMC free article: PMC2566672] [PubMed: 11693984]
- 97.
- Morley D, Woodland M, Cuthbertson WF. Controlled trial of pyrimethamine in pregnant women in an African village. BMJ. 1964;5384:667–668. [PMC free article: PMC1813732] [PubMed: 14096460]
- 98.
- Garner P, Gulmezoglu AM. Prevention versus treatment for malaria in pregnant women. Cochrane Database Syst Rev. 2000;2:CD000169. [PubMed: 10796500]
- 99.
- Greenwood BM, Greenwood AM, Snow RW, Byass P, Bennett S. Hatib-N’Jie AB. The effects of malaria chemoprophylaxis given by traditional birth attendants on the course and outcome of pregnancy. Trans R Soc Trop Med Hyg. 1989;83:589–594. [PubMed: 2617619]
- 100.
- WHO, 2002. WHO Expert Committee on Malaria. Geneva: World Health Organization.
- 101.
- Shulman CE, Dorman EK, Cutts F, Kawuondo K, Bulmer JN, Peshu N, Marsh K. Intermittent sulphadoxine-pyrimethamine to prevent severe anaemia secondary to malaria in pregnancy: a randomised placebo-controlled trial. Lancet. 1999;353:632–636. [PubMed: 10030329]
- 102.
- Rogerson SJ, Chaluluka E, Kanjala M, Mkundika P, Mhango C, Molyneux ME. Intermittent sulfadoxine-pyrimethamine in pregnancy: effectiveness against malaria morbidity in Blantyre, Malawi, in 1997–99. Trans R Soc Trop Med Hyg. 2000;94:549–553. [PubMed: 11132387]
- 103.
- Parise ME, Ayisi JG, Nahlen BL, Schultz LJ, Roberts JM, Misore A, Muga R, Oloo AJ, Steketee RW. Efficacy of sulfadoxine-pyrimethamine for prevention of placental malaria in an area of Kenya with a high prevalence of malaria and human immunodeficiency virus infection. Am J Trop Med Hyg. 1998;59:813–822. [PubMed: 9840604]
- 104.
- Newman RD, Parise ME, Slutsker L, Nahlen B, Steketee RW. Safety, efficacy and determinants of effectiveness of antimalarial drugs during pregnancy: implications for prevention programmes in Plasmodium falciparum-endemic sub-Saharan Africa. Trop Med Int Health. 2003;8:488–506. [PubMed: 12791054]
- 105.
- Greenwood B. The use of anti-malarial drugs to prevent malaria in the population of malaria-endemic areas. Am J Trop Med Hyg. 2004;71:1–7. [PubMed: 14971690]
- 106.
- Wolfe EB, Parise ME, Haddix AC, Nahlen BL, Ayisi JG, Misore A, Steketee RW. Cost-effectiveness of sulfadoxine-pyrimethamine for the prevention of malaria-associated low birth weight. Am J Trop Med Hyg. 2001;64:178–186. [PubMed: 11442215]
- 107.
- Goodman CA, Mills AJ. The evidence base on the cost-effectiveness of malaria control measures in Africa. Health Policy Plan. 1999;14:301–312. [PubMed: 10787646]
- 108.
- McGregor IA, Gilles HM, Walters JH, Davies AH, Pearson FA. Effects of heavy and repeated malarial infections on Gambian infants and children; effects of erythrocytic parasitization. BMJ. 1956;32:686–692. [PMC free article: PMC2035220] [PubMed: 13356045]
- 109.
- Bjorkman A, Brohult J, Pehrson PO, Willcox M, Rombo L, Hedman P, Kollie E, Alestig K, Hanson A, Bengtsson E. Monthly antimalarial chemotherapy to children in a holoendemic area of Liberia. Ann Trop Med Parasitol. 1986;80:155–167. [PubMed: 3530156]
- 110.
- Menendez C, Kahigwa E, Hirt R, Vounatsou P, Aponte JJ, Font F, Acosta CJ, Schellenberg DM, Galindo CM, Kimario J, Urassa H, Brabin B, Smith TA, Kitua AY, Tanner M, Alonso PL. Randomised placebo-controlled trial of iron supplementation and malaria chemoprophylaxis for prevention of severe anaemia and malaria in Tanzanian infants. Lancet. 1997;350:844–850. [PubMed: 9310602]
- 111.
- Schellenberg D, Menendez C, Kahigwa E, Aponte J, Vidal J, Tanner M, Mshinda H, Alonso P. Intermittent treatment for malaria and anaemia control at time of routine vaccinations in Tanzanian infants: a randomised, placebo-controlled trial. Lancet. 2001;357:1471–1477. [PubMed: 11377597]
- 112.
- Massaga JJ, Kitua AY, Lemnge MM, Akida JA, Malle LN, Ronn AM, Theander TG, Bygbjerg IC. Effect of intermittent treatment with amodiaquine on anaemia and malarial fevers in infants in Tanzania: a randomised placebo-controlled trial. Lancet. 2003;361:1853–1860. [PubMed: 12788572]
- 113.
- Ekvall H, Premji Z, Bennett S, Bjorkman A. Hemoglobin concentration in children in a malaria holoendemic area is determined by cumulated Plasmodium falciparum parasite densities. Am J Trop Med Hyg. 2001;64:58–66. [PubMed: 11425164]
- 114.
- Bloland PB, Lackritz EM, Kazembe PN, Were JB, Steketee R, Campbell CC. Beyond chloroquine: implications of drug resistance for evaluating malaria therapy efficacy and treatment policy in Africa. J Infect Dis. 1993;167:932–937. [PubMed: 8450258]
- 115.
- Ekvall H, Premji Z, Bjorkman A. Chloroquine treatment for uncomplicated childhood malaria in an area with drug resistance: early treatment failure aggravates anaemia. Trans R Soc Trop Med Hyg. 1998;92:556–560. [PubMed: 9861379]
- 116.
- Trape JF, Pison G, Preziosi MP, Enel C, Desgrees du Lou A, Delaunay V, Samb B, Lagarde E, Molez JF, Simondon F. Impact of chloroquine resistance on malaria mortality. Comp R Acad Sci III. 1998;321:689–697. [PubMed: 9769862]
- 117.
- Snow RW, Trape JF, Marsh K. The past, present and future of childhood malaria mortality in Africa. Trends Parasitol. 2001;17:593–597. [PubMed: 11756044]
- 118.
- WHO, 2001. Antimalarial Drug Combination Therapy, Report of a WHO Technical Consultation. Geneva: World Health Organization, 2001.
- 119.
- Adjuik M, Babiker A, Garner P, Olliaro P, Taylor W, White N. Artesunate combinations for treatment of malaria: meta-analysis. Lancet. 2004;363:9–17. [PubMed: 14723987]
- 120.
- Awasthi S, Pande VK. Six-monthly de-worming in infants to study effects on growth. Indian J Pediatr. 2001;68:823–827. [PubMed: 11669028]
- 121.
- Plata-Salaman CR. Cytokine-induced anorexia. Behavioral, cellular, and molecular mechanisms. Ann N Y Acad Sci. 1998;856:160–170. [PubMed: 9917876]
- 122.
- Jelkmann W. Proinflammatory cytokines lowering erythropoietin production. J Interferon Cytokine Res. 1998;18:555–559. [PubMed: 9726435]
- 123.
- World Health Organization, 2003. Report of the WHO Informal Consultation on the Use of Praziquantel during Pregnancy/Lactation and Albendazole/Mebendazole in Children under 24 Months. Geneva: World Health Organization.
- 124.
- Means RT Jr, Cytokines and anaemia in human immunodeficiency virus infection. Cytokines Cell Mol Ther. 1997;3:179–186. [PubMed: 9426976]
- 125.
- Newell ML, Gray G, Bryson YJ. Prevention of mother-to-child transmission of HIV-1 infection. AIDS. 1997;11(Suppl A):S165–S172. [PubMed: 9451981]
- 126.
- Guay LA, Musoke P, Fleming T, Bagenda D, Allen M, Nakabiito C, Sherman J, Bakaki P, Ducar C, Deseyve M, Emel L, Mirochnick M, Fowler MG, Mofenson L, Miotti P, Dransfield K, Bray D, Mmiro F, Jackson JB. Intrapartum and neonatal single-dose nevirapine compared with zidovudine for prevention of mother-to-child transmission of HIV-1 in Kampala, Uganda: HIVNET 012 randomised trial. Lancet. 1999;354:795–802. [PubMed: 10485720]
- 127.
- Aisen P, Enns C, Wessling-Resnick M. Chemistry and biology of eukaryotic iron metabolism. Int J Biochem Cell Biol. 2001;33:940–959. [PubMed: 11470229]
- 128.
- Andrews NC. Disorders of iron metabolism. N Engl J Med. 1999;341:1986–1995. [PubMed: 10607817]
- 129.
- Nemeth E, Valore EV, Territo M, Schiller G, Lichtenstein A, Ganz T. Hepcidin, a putative mediator of anemia of inflammation, is a type II acute-phase protein. Blood. 2003;101:2461–2463. [PubMed: 12433676]
- 130.
- International Nutritional Anemia Consultative Group, 1999. Safety of Iron Supplementation Programs in Malaria-Endemic Regions. Washington, DC: International Life Sciences Institute Press.
- 131.
- Gera T, Sachdev H. Effect of iron supplementation on incidence of infectious illness in children: systematic review. BMJ. 2002;325:1142. [PMC free article: PMC133452] [PubMed: 12433763]
- 132.
- Alonzo Gonzalez M, Menendez C, Font F, Kahigwa E, Kimario J, Mshinda H, Tanner M, Bosch-Capblanch X, Alonso PL. Cost-effectiveness of iron supplementation and malaria chemoprophylaxis in the prevention of anaemia and malaria among Tanzanian infants. Bull World Health Organ. 2000;78:97–107. [PMC free article: PMC2560593] [PubMed: 10686744]
- 133.
- Shankar AH, Genton B, Semba RD, Baisor M, Paino J, Tamja S, Adiguma T, Wu L, Rare L, Tielsch JM, Alpers MP, West KP Jr, Effect of vitamin A supplementation on morbidity due to Plasmodium falciparum in young children in Papua New Guinea: a randomised trial. Lancet. 1999;354:203–209. [PubMed: 10421302]
- 134.
- Binka FN, Ross DA, Morris SS, Kirkwood BR, Arthur P, Dollimore N, Gyapong JO, Smith PG. Vitamin A supplementation and childhood malaria in northern Ghana. Am J Clin Nutr. 1995;61:853–859. [PubMed: 7702031]
- 135.
- Villamor E, Mbise R, Spiegelman D, Hertzmark E, Fataki M, Peterson KE, Ndossi G, Fawzi WW. Vitamin A supplements ameliorate the adverse effect of HIV-1, malaria, and diarrheal infections on child growth. Pediatrics. 2002;109:E6. [PubMed: 11773574]
- 136.
- Fawzi WW, Chalmers TC, Herrera MG, Mosteller F. Vitamin A supplementation and child mortality. A meta-analysis. JAMA. 1993;269:898–903. [PubMed: 8426449]
- 137.
- WHO, 1997. Vitamin A Supplements, A Guide to Their Use in the Treatment and Prevention of Vitamin A Deficiency and Xerophthalmia. Geneva: World Health Organization.
- 138.
- Shankar AH, Genton B, Baisor M, Paino J, Tamja S, Adiguma T, Wu L, Rare L, Bannon D, Tielsch JM, West KP Jr,, Alpers MP. The influence of zinc supplementation on morbidity due to Plasmodium falciparum: a randomized trial in preschool children in Papua New Guinea. Am J Trop Med Hyg. 2000;62:663–669. [PubMed: 11304051]
- 139.
- Bates CJ, Evans PH, Dardenne M, A, Lunn PG, Northrop-Clewes CA, Hoare S, Cole TJ, Horan SJ, Longman SC. A trial of zinc supplementation in young rural Gambian children. Br J Nutr. 1993;69:243–255. [PubMed: 8457531]
- 140.
- Muller O, Becher H, van Zweeden AB, Ye Y, Diallo DA, Konate AT, Gbangou A, Kouyate B, Garenne M. Effect of zinc supplementation on malaria and other causes of morbidity in west African children: randomised double blind placebo controlled trial. BMJ. 2001;322:1567. [PMC free article: PMC33513] [PubMed: 11431296]
- 141.
- Darnton-Hill I, Nalubola R. Fortification strategies to meet micronutrient needs: successes and failures. Proc Nutr Soc. 2002;61:231–241. [PubMed: 12133205]
- 142.
- Nestel P, Briend A, de Benoist B, Decker E, Ferguson E, Fontaine O, Micardi A, Nalubola R. Complementary food supplements to achieve micronutrient adequacy for infants and young children. J Pediatr Gastroenterol Nutr. 2003;36:316–328. [PubMed: 12604969]
- 143.
- Bouis HE. Micronutrient fortification of plants through plant breeding: can it improve nutrition in man at low cost? Proc Nutr Soc. 2003;62:403–411. [PubMed: 14506888]
- 144.
- Allen LH. Interventions for micronutrient deficiency control in developing countries: past, present and future. J Nutr. 2003;133(11 Suppl 2):3875S–3878S. [PubMed: 14672284]
- 145.
- Davis P, Howden-Chapman P. Translating research findings into health policy. Soc Sci Med. 1996;43:865–872. [PubMed: 8870150]
- 146.
- Editorial WHO 2003–08: a programme of quiet thunder takes shape. Lancet. 2003;362:179. [PubMed: 12885472]
- 147.
- English M, Waruiru C, Amukoye E, Murphy S, Crawley J, Mwangi I, Peshu N, Marsh K. Deep breathing in children with severe malaria: indicator of metabolic acidosis and poor outcome. Am J Trop Med Hyg. 1996;55:521–524. [PubMed: 8940984]
- 148.
- Meremikwu M, Smith H J. Blood transfusion for treating malarial anaemia. Cochrane Database Syst Rev. 2000;2:CD001475. [PMC free article: PMC6532690] [PubMed: 10796646]
- 149.
- Chang TM. Present status of modified hemoglobin as blood substitutes and oral therapy for end stage renal failure using artificial cells containing genetically engineered cells. Ann N Y Acad Sci. 2001;944:362–372. [PubMed: 11797686]
- 150.
- Muller O, Jahn A, von Braun J. Micronutrient supplementation for malaria control—hype or hope? Trop Med Int Health. 2002;7:1–3. [PubMed: 11851948]
Footnotes
Received February 23, 2004.Accepted for publication April 27, 2004.
Author’s address: Jane Crawley, Roll Back Malaria Department, World Health Organization, Geneva, Switzerland, Telephone: 41-22-791-3214, Fax: 41-22-791-4824, E-mail: crawleyj@who.int.
- Reducing the Burden of Anemia in Infants and Young Children in Malaria-endemic C...Reducing the Burden of Anemia in Infants and Young Children in Malaria-endemic Countries of Africa: From Evidence to Action - The Intolerable Burden of Malaria II: What's New, What's Needed
Your browsing activity is empty.
Activity recording is turned off.
See more...