NCBI Bookshelf. A service of the National Library of Medicine, National Institutes of Health.
Feingold KR, Anawalt B, Blackman MR, et al., editors. Endotext [Internet]. South Dartmouth (MA): MDText.com, Inc.; 2000-.
ABSTRACT
Abnormalities in lipid metabolism are very commonly observed in patients who are obese. Approximately 60-70% of patients with obesity are dyslipidemic. The lipid abnormalities in patients who are obese include elevated serum TG, VLDL, apolipoprotein B, and non-HDL-C levels. The increase in serum TG is due to increased hepatic production of VLDL particles and a decrease in the clearance of TG rich lipoproteins. HDL-C levels are typically low and are associated with the increase in serum TG. LDL-C levels are frequently in the normal range or only slightly elevated but there is an increase in small dense LDL. Patients who are obese are at an increased risk of developing cardiovascular disease and therefore treatment of their dyslipidemia is often indicated. Life style induced weight loss will decrease serum TG and LDL-C levels and increase HDL-C levels. In most patients the changes in lipid levels with life style induced weight loss are not very robust and are proportional to the change in weight. Dietary constituents of a weight loss diet have a small but significant impact on the changes in lipid levels. Low carbohydrate diets decrease TG levels to a greater extent than high carbohydrate diets. High fat diets blunt the decrease in LDL-C that occurs with weight loss. The increase in HDL-C with weight loss is greatest with a high fat diet but the significance of this increase on cardiovascular disease risk is uncertain. Weight loss medications will also improve dyslipidemia. Bariatric surgery results in robust weight loss and has a marked effect on serum lipid levels. Remission of hyperlipidemia with gastric bypass surgery is frequently observed. The reduction in cardiovascular disease with statin therapy is no different in patients with a BMI >30 or BMI <25 (i.e., statins are effective in patients who are obese). Many, if not most, patients who are obese should be on statin therapy and some will require the addition of other LDL-C decreasing drugs to achieve satisfactory reductions in LDL-C. The mixed dyslipidemia that is frequently observed in patients who are obese will often require combination therapy. However, recent studies have failed to demonstrate that adding fibrates or niacin to statin therapy provides additional benefits beyond statins alone. However, the addition of the omega-3-fatty acid, icosapent ethyl, to statin therapy has been shown to decrease cardiovascular events. For complete coverage of all related areas of Endocrinology, please visit our on-line FREE web-text, WWW.ENDOTEXT.ORG.
INTRODUCTION
The prevalence of obesity has increased dramatically over the last several decades (1,2). In the United States it is estimated that approximately 35% of men and 40% of women are obese defined as a BMI >30 kg/m2 (2,3). Additionally, approximately 1/3 of the population is overweight defined as a BMI between 25 and 30 kg/m2 (2,4). Moreover, the obesity epidemic is not localized to the United States as there has been a marked increase in the prevalence of obesity worldwide (5). The number of individuals with morbid obesity (BMI > 40) has also greatly increased (6). It should be noted that very athletic individuals may have a high BMI without excess body fat (the increase in weight is due to muscle mass) and as a consequence not have metabolic abnormalities. Conversely, in certain ethnic groups obesity occurs even though the BMI is in the normal range (7). Of great concern is that the prevalence of obesity has also markedly increased in children (8). Obesity is associated with insulin resistance, alterations in lipid metabolism, and the metabolic syndrome, particularly when the excess adipose tissue is located in an intra-abdominal location or in the upper chest (9-11). Obesity is a risk factor for the development of cardiovascular disease, but it appears that much of this effect is accounted for by obesity inducing dyslipidemia, diabetes, hypertension, inflammation, and a procoagulant state (9-13). The majority of deaths related to high BMI are due to cardiovascular disease (5).
LIPID ABNORMALITIES IN PATIENTS WITH OBESITY
The lipid abnormalities seen in patients who are obese include elevated TG, VLDL, Apo B, and non-HDL-C levels, which are all commonly observed (9,10,14,15). HDL-C and Apo A-I levels are typically low (9,10,14,15). LDL-C levels are frequently in the normal to slightly elevated range, but an increase in small dense LDL is often seen resulting in an increased number of LDL particles (9,10,14,15). These small dense LDL particles are considered to be more pro-atherogenic than large LDL particles for a number of reasons (16). Small dense LDL particles have a decreased affinity for the LDL receptor resulting in a prolonged period of time in the circulation. Additionally, these small particles enter the arterial wall more easily than large particles and then they bind more avidly to intra-arterial proteoglycans, which traps them in the arterial wall. Finally, small dense LDL particles are more susceptible to oxidation, which could result in an enhanced uptake by macrophages. Postprandial TG levels are also increased in subjects with obesity and these chylomicron remnants are pro-atherogenic (17,18). The greater the increase in BMI the greater the abnormalities in lipid levels. Approximately 60-70% of patients who are obese are dyslipidemic while 50-60% of patients who are overweight are dyslipidemic (9). Notably, obesity in children and young adults also leads to an increased prevalence of elevated TG and decreased HDL-C levels (19). The increased risk for cardiovascular disease in patients with obesity is partially accounted for by this dyslipidemia.
Table 1.
Lipid and Lipoprotein Levels in Patients who are Obese
Increased TG |
Increased VLDL |
Increased apo B |
Increased non-HDL-C |
Increased small dense LDL |
Increased LDL particle number |
Decreased HDL-C |
Decreased apo A1 |
LDL-C and Lp(a) are not altered |
It should be emphasized that the effects of obesity on lipid metabolism are dependent on the location of the adipose tissue (20-24). Increased visceral adipose tissue and trunk (especially upper trunk) subcutaneous adipose tissue are associated with higher TG and lower HDL-C levels. In contrast, increased subcutaneous adipose tissue in the leg is associated with lower TG levels. The protective effect of leg fat may explain why women and African-Americans have lower TG levels. In addition, increased visceral adipose tissue and upper trunk subcutaneous adipose tissue are associated with insulin resistance, which may contribute to the lipid changes described above.
PATHOPHYSIOLOGY OF THE DYSLIPIDEMIA OF OBESITY

Figure 1.
Changes in Lipid/Lipoprotein Metabolism Leading to the Dyslipidemia of Obesity.
Production of TG Rich Lipoproteins
There are a number of different abnormalities that contribute to the dyslipidemia seen in patients with obesity (figure 1) (9,15,18,25,26). These abnormalities are driven by the combination of the greater delivery of free fatty acids to the liver from increased total and visceral adiposity, insulin resistance, and a pro-inflammatory state, induced by macrophages infiltrating fat tissue (9,15,18,25,26). A key abnormality is the overproduction of VLDL particles by the liver, which is an important contributor to the elevation in serum TG levels (9,15,18,25,26). The rate of secretion of VLDL particles is highly dependent on TG availability, which is determined by the levels of fatty acids available for the synthesis of TG in the liver. An abundance of TG prevents the intrahepatic degradation of Apo B-100 allowing for increased VLDL formation and secretion.
There are three major sources of fatty acids in the liver all of which may be altered in patients with obesity (9,15,18,25,26). First, the flux of fatty acids from adipose tissue to the liver is increased (9,25,26). An increased mass of adipose tissue, particularly visceral stores, results in increased fatty acid delivery to the liver. Additionally, insulin suppresses the lipolysis of TG to free fatty acids in adipose tissue. In patients with obesity a decrease in insulin activity due to insulin resistance results in the blunting of the inhibition of TG lipolysis and an increase in TG breakdown in adipose tissue leading to increased fatty acid deliver to the liver (9,26). A second source of fatty acids in the liver is de novo fatty acid synthesis. Numerous studies have shown that fatty acid synthesis is increased in the liver in patients with obesity (15,25,27). This increase may be mediated by the hyperinsulinemia seen in patients with insulin resistance. Specifically, insulin stimulates the activity of SREBP-1c, a transcription factor that increases the expression of the enzymes required for the synthesis of fatty acids. While the liver is insulin resistant to the effects of insulin on carbohydrate metabolism, the liver remains sensitive to the effects of insulin stimulating lipid synthesis (28). The third source of fatty acids is the uptake of TG rich lipoproteins by the liver. Studies have shown an increase in intestinal fatty acid synthesis accompanied by the enhanced secretion of chylomicrons in obesity (15,25,29). This increase in chylomicrons leads to the increased delivery of fatty acids to the liver. The increase in hepatic fatty acids by these three pathways results in an increase in the synthesis of TG in the liver and the protection of Apo B-100 from degradation resulting in the increased formation and secretion of VLDL (15,26). Additionally, the ability of insulin to suppress Apo B secretion is diminished in patients with obesity and marked insulin resistance (25,26). Finally, increased caloric intake may contribute to circulating TG, either by dietary fat leading to increased chylomicron TG levels and/or providing fatty acids to the liver or dietary carbohydrate enhancing de novo hepatic lipogenesis.
Metabolism of TG Rich Lipoproteins
In addition to the overproduction of TG rich lipoproteins by the liver and intestine there are also abnormalities in the subsequent metabolism of these TG rich lipoproteins, which contributes to the increase in TG levels (9,15,18,25). Patients who are obese have an increase in Apo C-III levels (25,30). Apo C-III expression is inhibited by insulin and hence the insulin resistance that occurs in patients with obesity could account for the increase in Apo C-III (25). Apo C-III is an inhibitor of lipoprotein lipase activity and could thereby reduce the clearance of TG rich lipoproteins (31). In addition, Apo C-III also inhibits the cellular uptake of TG rich lipoproteins (31). Recent studies have shown that loss of function mutations in Apo C-III lead to decreases in serum TG levels and a reduced risk of cardiovascular disease (32-34). Interestingly, inhibition of Apo C-III expression results in a decrease in serum TG levels even in patients deficient in lipoprotein lipase indicating that the ability of Apo C-III to modulate serum TG levels is not dependent solely on regulating lipoprotein lipase activity (35). Finally, if insulin resistance is severe the insulin induced stimulation of lipoprotein lipase may be reduced, which would also decrease the clearance of TG rich lipoproteins (18). Thus, a decrease in clearance of TG rich lipoproteins also contributes to the elevation in serum TG levels in patients with obesity.
Production of Small Dense LDL and HDL
The elevation in TG rich lipoproteins in turn has effects on other lipoproteins (figure 1). Specifically, cholesterol ester transfer protein (CETP) mediates the equimolar exchange of TG from TG rich VLDL and chylomicrons for cholesterol from LDL and HDL (9,10,14,18). The increase in TG rich lipoproteins per se leads to an increase in CETP mediated exchange, increasing the TG content and decreasing the cholesterol content of both LDL and HDL. Additionally, obesity also increases the activity and mass of CETP (14). This CETP-mediated exchange underlies the commonly observed reciprocal relationship of low HDL-C levels when TG levels are high and the increase in HDL-C when TG levels decrease.
The TG on LDL and HDL is then hydrolyzed by hepatic lipase and lipoprotein lipase leading to the production of small dense LDL and small HDL particles (9,10,18). Notably hepatic lipase activity is increased in patients who are obese with increased visceral adiposity, which will facilitate the removal of TG from LDL and HDL resulting in small lipoprotein particles (9,10,18). The affinity of Apo A-I for small HDL particles is reduced leading to the disassociation of Apo A-I and the clearance and breakdown of Apo A-I by the kidneys (9). These changes result in reduced levels of Apo A-I and HDL-C in patients who are obese.
Role of Inflammation and Adipokines
Obesity is a pro-inflammatory state due to macrophages that infiltrate adipose tissue. The cytokines produced by macrophages and the adipokines that are produced by fat cells also alter lipid metabolism (26,36-38).
Adipokines, such as adiponectin and resistin, regulate lipid metabolism. The circulating levels of adiponectin are decreased in subjects who are obese (39). Decreased adiponectin levels are associated with elevations in serum TG levels and decreases in HDL-C levels (39). This association is thought to be causal as studies in mice have shown that overexpressing adiponectin (transgenic mice) decreases TG and increases HDL-C levels while conversely, adiponectin knock-out mice have increased TG and decreased HDL-C levels (39). The adiponectin induced decrease in TG levels is mediated by an increased catabolism of TG rich lipoproteins due to an increase in lipoprotein lipase activity and a decrease Apo C-III, an inhibitor of lipoprotein lipase (39). The increase in HDL-C levels induced by adiponectin is mediated by an increase in hepatic Apo A-I and ABCA1, which results in the increased production of HDL particles (39).
Resistin is increased in subjects who are obese and the levels of resistin directly correlate with plasma TG levels (40). Moreover, resistin has been shown to stimulate hepatic VLDL production and secretion due to an increase in the synthesis of Apo B, TG, and cholesterol (26,40). Finally, resistin is associated with a decrease in HDL-C and Apo A-I levels (26).
The pro-inflammatory cytokines, TNF and IL-1, stimulate lipolysis in adipocytes increasing circulating free fatty acid levels, which will provide substrate for hepatic TG synthesis (37). In the liver, pro-inflammatory cytokines stimulate de novo fatty acid and TG synthesis (37). These alterations will lead to the increased production and secretion of VLDL. At higher levels the pro-inflammatory cytokines decrease the expression of lipoprotein lipase and increase the expression of angiopoietin like protein 4, an inhibitor of lipoprotein lipase (37,41). Together these changes decrease lipoprotein lipase activity, thereby delaying the clearance of TG rich lipoproteins. Thus, increases in the levels of pro-inflammatory cytokines will stimulate the production of TG rich lipoproteins and delay the clearance of TG rich lipoproteins, which together will contribute to the increase in serum TG that occurs in patients with obesity.
Pro-inflammatory cytokines also affect HDL metabolism (42,43). First, they decrease the production of Apo A-I, the main protein constituent of HDL. Second, in macrophages pro-inflammatory cytokines decrease the expression of ABCA1 and ABCG1, which will lead to a decrease in the efflux of phospholipids and cholesterol from the cell to HDL. Third, pro-inflammatory cytokines decrease the production and activity of LCAT, which will limit the conversion of cholesterol-to-cholesterol esters in HDL. This step is required for the formation of a normal spherical HDL particle and facilitates the ability of HDL to transport cholesterol. Together these changes induced by pro-inflammatory cytokines could result in a decrease in HDL-C and Apo AI levels.
CURRENT TREATMENT GUIDELINES FOR SERUM LIPIDS
The purpose of treating lipid disorders is to prevent the development of other diseases, particularly cardiovascular disease. Thus, the decision to treat should be based on the risk of the hyperlipidemia leading to those medical problems. A number of guidelines have been published that discuss in detail cardiovascular risk assessment and provide recommendations on treatment strategies (44-48). It should be noted that while these guidelines are similar there are significant differences between their recommendations. The current American College of Cardiology/American Heart Association (ACC/AHA) guidelines do not emphasize specific lipid/lipoprotein goals of therapy but rather to just treat with statins to lower LDL-C by a certain percentage (44). An exception is that they do recommend in patients with very high-risk ASCVD, to use an LDL-C threshold of 70 mg/dL to consider addition of non-statins to statin therapy. In contrast, other groups, such as the National Lipid Association, International Atherosclerosis Society, European Society of Cardiology/European Atherosclerosis Society, and American Association of Clinical Endocrinologists (AACE), do recommend lowering the LDL-C and non-HDL-C levels to below certain levels depending upon the cardiovascular risk in a particular patient but the recommendations from these organizations are not identical (43,45-47) (49).These issues are discussed in detail in the chapters on Guidelines for the Management of High Blood Cholesterol (50). In addition to cardiovascular complications, marked elevations in TG can lead to pancreatitis (51). The National Lipid Association recommends treating TG levels greater than 500mg/dL while the Endocrine Society recommends treating TG if they are greater than 1000mg/dL to lower the risk of pancreatitis (48,52).
It should be noted that most lipid experts would recommend trying to achieve an LDL-C levels less than 70mg/dL and non-HDL-C levels less than 100mg/dL at a minimum in patients with cardiovascular disease or patients at very high risk for the development of cardiovascular disease. AACE and European Society of Cardiology/European Atherosclerosis Society have recommended LDL-C levels less than 55mg/dL in patients at very high risk (47,49). In other patients, an LDL-C level less than 100mg/dL and non-HDL-C level less than 130mg/dL is a reasonable goal. The recommendations for evaluating and treating dyslipidemia in patients with obesity are the same as non-obese patients. For additional details on deciding who to treat and the goals of therapy see other Endotext chapters (50,51,53)
MODALITIES TO TREAT LIPID ABNORMALITIES IN PATIENTS WITH OBESITY
Effect of Diet
There are two issues with regards to diet. First is the effect of weight loss on serum lipids. Second is the effect of dietary constituents (macronutrients) on serum lipids. For additional details on the effect of diet on lipid and lipoproteins please see the chapter “The Effect of Diet on Cardiovascular Disease and Lipid and Lipoprotein Levels” (54) and for additional information on weight loss diets see the chapter on “Dietary Treatment of Obesity” (55).
LOW-CALORIE DIET-INDUCED WEIGHT LOSS
In 1992, Dattilo and Kris-Etherton published a meta-analysis that evaluated the effect of weight loss on serum lipids (56). For every 1Kg decrease in body weight there was a 0.77mg/dL decrease in LDL-C and 1.33mg/dL decrease in TG. The effect of weight loss on HDL-C was more complex. For every 1kg decrease in body weight there is a 0.27mg/dL decrease in HDL-C during active weight loss. However, when weight is stabilized there is a 0.35mg/dL increase in HDL-C for every 1kg decrease in body weight that has occurred. In a more recent systemic review and meta-analysis Zomer et al. reported that a 5-10% weight loss resulted in a 16mg/dL decrease in TG, 10mg/dL decrease in LDL-C, and a nonsignificant effect on HDL (+0.5 mg/dL) (57). Finally, in a very recent meta-analysis of 30 randomized controlled trials with 2,434 participants by Hasan et al it was reported that lifestyle (diet and/or exercise) induced weight loss at 12 months resulted in a 4mg/dL decrease in TG, a 1.28 mg/dL decease in LDL-C, and 0.46 mg/dL increase in HDL-C per 1kg weight loss (58). Using the results of the most recent meta-analysis if a patient lost 10Kg of body weight and maintained the weight loss, one would expect that LDL-C would have decreased by 12.8mg/dL, TG would have decreased by 40mg/dL, and HDL-C would have increased by 4.6mg/dL. Of course, many, if not most patients, will not be able to loss 10kg and maintain that weight loss for an extended period of time.
Additionally, one should recognize that the response of lipid levels to weight loss will vary greatly in individual patients but in general one can expect a decrease in serum TG and LDL-C levels and an increase in HDL-C levels. The degree of decrease in serum TG levels is related to baseline TG levels with higher levels typically demonstrating a greater reduction with weight loss. In the Look-Ahead Trial decreases in LDL-C (15-18mg/dL) and TG (21-25mg/dL) levels were similar across BMI categories in lifestyle participants who lost weight but in the severely obese the increase in HDL-C levels was blunted (59). Interestingly, in metabolically healthy patients who are obese and do not have lipid abnormalities or other metabolic abnormalities a calorie deficient diet still results in a decrease in TG with no change in HDL-C levels (60). Finally, a systematic review of studies has shown that weight loss in children also decreases TG and increases HDL-C levels (61).
Whether particular diets are better at inducing weight loss is hotly debated with many “experts” recommending certain diets as being advantageous. Sacks and colleagues in a large trial of 811 obese subjects compared four different diets (fat 20%/protein 15%/carbohydrate 65%; fat 20%/protein 25%/carbohydrate 55%; fat 40%/protein 121-25mg/dL)5%/carbohydrate 45%; and fat 40% protein 25% carbohydrate 35%) in which total calories were also reduced. They found that after two years weight loss was similar (62). Other studies that compared different diets over an extended of time period (at least one year) have reached similar conclusions (63-65). During the first 6 months of many diet studies, patients lose a significant amount of weight but unfortunately over an extended period of time most patients regain weight such that after two years the amount of weight loss is relatively modest. For example, in the study of Sacks et al patients lost approximately 6kg of weight during the first six months but by 24 months the total weight loss was only between 3-4kg (62). It is therefore essential that one focuses on “long-term” studies when comparing different diet approaches. At this time, it is not clear that any particular diet is “best” for inducing weight loss and individual weight loss is highly variable. The key is the ability of the patient to follow the diet for an extended period of time.
DIETARY MACRONUTRIENT CONSTITUENTS
The effect of different weight loss diets that differ by macronutrient content on the lipid profile has been evaluated in a large number of studies. A meta-analysis by Hu and colleagues examined the effect of a low-carbohydrate vs. a low-fat diet in 23 studies with 2,788 participants (64). They found, as expected, that both types of weight loss diets decreased LDL-C and TG levels and increased HDL-C levels. However, the low carbohydrate diet decreased TG and increased HDL-C to a greater extent than the low-fat diet. Conversely, the low-fat diet was more effective in lowering LDL-C levels. The results of this meta-analysis are shown in table 2. It should be noted that the magnitude of these changes, except for the decrease in TG are small. Similarly, Mansoor et al in a meta-analysis of 11 RCT with 1369 participants reported that a low carbohydrate diet resulted in a decreased TG level (23mg/dL) and increased HDL-C level (5.5mg/dL) compared to a high fat diet but LDL-C levels were also increased (6.2mg/dL) with the low carbohydrate diet (66). Additionally, Naude and colleagues in a meta-analysis of 12 studies with 1603 subjects, found that a low carbohydrate diet compared to a “balanced” diet resulted in lower TG levels and higher HDL-C and LDL-C levels but once again the differences were relatively small (67). Additionally, a meta-analysis by Schwingshackl and Hoffmann compared high fat vs. low fat diets and observed that the decrease in LDL-C was more pronounced with a low-fat diet whereas the increase in HDL-C and the decrease in TG were greater with the high-fat diet (68). Finally, a meta-analysis comparing ketogenic diets that are very-low in carbohydrates with low-fat diets resulted, as expected, in greater decreases in TG and increases in HDL-C levels with the ketogenic than the low-fat diet but the ketogenic diet leads to an increase in LDL-C levels (69).
Table 2.
Comparison of High and Low Fat and Carbohydrate Diets (64)
Low carbohydrate/high fat | Low fat/high carbohydrate | |
---|---|---|
Weight Loss (kg) | -6.1 | -5.0 |
LDL-C (mg/dL) | -2.1 | -6.0 |
HDL-C (mg/dL) | 4.5 | 1.6 |
TG (mg/dL) | -30.4 | -17.1 |
While the typical increases in LDL-C levels observed with a ketogenic diet are modest, recently a series of reports have described marked elevations in LDL-C levels in some patients on a ketogenic diet (70-72). For example, Goldberg et al reported 5 patients with marked increases in LDL-C levels on a ketogenic diet (73). Three patients had LDL-C levels greater than 500mg/dl. Similarly, Schaffer et al described 3 patients in which a very low carbohydrate diet induced LDL-C levels greater then 400mg/dL (74). Finally, Schmidt et al reported 17 patients with LDL-C levels greater than 200mg/dL on a ketogenic diet (75). In these patients there was an average increase in their LDL-C level of 187 mg/dL (75). The elevations in LDL-C levels decrease towards normal with cessation of the ketogenic diet (73-75). It should be noted that most of the patients with marked elevations in LDL-C in response to a ketogenic diet had normal LDL-C levels prior to the dietary change (71). This hyper response seems to occur more commonly in patients who are lean (71) but has also been seen in obese patients (73).
Many of the individuals who develop marked increases in LDL-C on a very low carbohydrate ketogenic diet have low triglyceride levels, elevated HDL-C levels, and are thin (70,71). This phenotype has been called the lean mass hyper-responder (LMHR) phenotype (70,71). LMHR individuals have been defined as having triglycerides <70mg/dL, HDL-C > 80mg/dL, and LDL-C > 200mg/dL (70,71). The mechanism for the marked increase in LDL-C levels is unknown.
A meta-analysis by Wycherley et al evaluated the effect of high protein vs. low protein diet on lipid levels in 24 studies with over a 1000 subjects (76). Weight loss was similar between the two diet strategies with less than a 1kg difference in weight loss between the high protein vs. low protein diets. Similarly, there were no differences in LDL-C or HDL-C levels but the high protein diet resulted in a greater decrease in TG levels (20mg/dL). A meta-analysis by Schwingshackl and Hoffmann compared the effect of low fat diets that were either low or high in protein (77). They observed no significant differences in LDL-C, HDL-C, or TG levels indicating that high protein diets have neither beneficial nor detrimental effects on lipid levels.
There are many diet programs that a widely advertised. In a network meta-analysis of 121 eligible trials with 21, 942 overweight or obese patients Ge and colleagues compared the effect of 14 different diets on LDL-C and HDL-C levels (78). The diets could be grouped into low CHO diets (Atkins, South Beach, Zone), moderate macronutrients diets (Biggest Loser, DASH, Jenny Craig, Mediterranean, Portfolio, Slimming World, Volumetrics, Weight Watchers), and low-fat diets (Ornish, Rosemary Conley). The effect of these different diets on LDL-C and HDL-C levels are shown in table 3. It should be noted that despite considerable weight loss the effect of these diets on LDL-C and HDL-C levels was very modest except for the LDL-C lowering seen with the Portfolio diet. The portfolio dietary pattern is a plant-based dietary pattern that includes four cholesterol-lowering foods; a) tree nuts or peanuts, b) plant protein from soy products, beans, peas, chickpeas, or lentils, c) viscous soluble fiber from oats, barley, psyllium, eggplant, okra, apples, oranges, or berries, and d) plant sterols initially provided in a plant sterol-enriched margarine. Unfortunately, a comparison of the effect of these 14 different diets on TG levels was not reported.
Table 3.
Effect of Different Diets in Comparison with Usual Diet
Diet vs. Usual Diet | Decrease in Weight (Kg) | Change in LDL-C (mg/dL) | Change in HDL-C (mg/dL) |
---|---|---|---|
Atkins | 5.46 | +2.75 | -3.41 |
Zone | 4.07 | +2.89 | +0.33 |
Dash | 3.63 | -3.93 | +1.90 |
Mediterranean | 2.87 | -4.59 | +0.61 |
Paleolithic | 5.31 | -7.27 | +2.52 |
Low Fat | 4.87 | -1.92 | +2.13 |
Jenny Craig | 7.77 | -0.21 | +2.85 |
Volumetrics | 5.95 | -7.13 | +0.13 |
Weight Watchers | 3.90 | -7.13 | +0.88 |
Rosemary Conley | 3.76 | -7.15 | +2.04 |
Ornish | 3.64 | -4.71 | +4.87 |
Portfolio | 3.64 | -21.29 | +3.26 |
Biggest Loser | 2.88 | -3.90 | +0.01 |
Slimming World | 2.15 | N/A | N/A |
South Beach | 9.86 | +0.64 | -3.60 |
Dietary Advice | 0.31 | +2.01 | +1.71 |
In a study carried out in a single center the Atkins, Zone, Weight Watchers, and Ornish diets were compared and the effect on TG levels was also reported (79). Table 4 shows the results of this study at 2 months, a period at which dietary compliance was still high. The magnitude of weight loss was similar but the decrease in LDL-C that occurs with weight loss was blunted with a diet that was high in fat (Atkins diet). In contrast HDL-C levels increased with a high fat diet, particularly saturated fatty acids (Atkins diet) and decreased with a very low-fat diet (Ornish diet). The weight loss induced decrease in TG levels was blunted by a high carbohydrate intake (Ornish diet). These observations confirm and extend the results described above.
Table 4.
Effect of Different Diets on Lipid Levels
Weight (kg) | LDL-C (mg/dL) | HDL-C (mg/dL) | TG (mg/dL) | |
---|---|---|---|---|
Atkins | -3.6 | 1.3 | 3.2 | -32 |
Zone | -3.8 | -9.7 | 1.8 | -54 |
Weight Watchers | -3.5 | -12.1 | -0.2 | -9.2 |
Ornish | -3.6 | -16.5 | -3.6 | -0.4 |
In summary, low carbohydrate/high fat diets result in greater decreases in TG and increases in HDL-C but LDL-C decreases are blunted. In contrast, low fat/high carbohydrate diets are more effective in lowering LDL-C levels but the decrease in TG and the increase in HDL-C are blunted. Stated simply, diets that contain carbohydrates tend to increase TG levels while diets high in fat increase HDL-C levels and if they contain saturated fats and/or trans fats increase LDL-C levels.
GLYCEMIC INDEX
The glycemic index of foods is a marker for the rapid absorption and appearance of glucose in the blood during meal consumption. Higher glycemic index foods result in greater glucose and insulin excursions than low-glycemic index foods. A meta-analysis by Goff et al has examined the effect of foods with a high glycemic index vs. foods with a low glycemic index in 28 studies with over a 1000 subjects (80). There was no significant effect of glycemic index on either HDL-C or TG levels. However, the low glycemic index foods resulted in a small decrease in LDL-C (approximately 6mg/dL). The decrease in LDL-C was only seen in the studies where the low glycemic diet also had an increase in dietary fiber, indicating that the observed differences were likely due to dietary fiber, a factor well known to decrease LDL-C levels. The effect of glycemic index has to be distinguished from that of carbohydrate levels. Indeed, in a recent study in which fiber was kept constant, a low glycemic index diet increased LDL-C when carbohydrate intake was high, but decreased LDL-C when carbohydrate was low (81).
To summarize dietary constituents of weight loss diets have a small but significant impact on the changes in lipid levels. Low carbohydrate diets decrease TG levels to a greater extent than high carbohydrate diets. High saturated fat diets blunt the decrease in LDL-C that occurs with weight loss. HDL-C levels increase with weight loss and this increase is greatest with a high fat diet. A weight loss diet that contains a markedly reduced fat content my result in a decrease in HDL-C levels. Finally, a diet high in soluble fiber will lower LDL-C levels. As should be apparent from these data the effect of diet induced weight loss on the lipid profile is modest and thus in most patient’s pharmacologic therapy will be required to induce significant changes in the lipid profile.
It should be emphasized that the inability to decrease weight with diet therapy is not a reason to abandon dietary therapy. Patients should be encouraged to decrease their intake of saturated fats (<7% of calories) and trans fats and increase their intake of soluble fiber, which will favorably effect LDL-C levels. Additionally, reducing intake of simple sugars and alcohol will lower TG levels. Thus, even in the absence of significant weight loss dietary therapy can be beneficial and should be encouraged.
Effect of Exercise
Exercise alone is usually not sufficient to induce significant weight loss (82,83). However, exercise when combined with diet therapy can facilitate weight loss and is considered very important for weight loss maintenance (82). It may also diminish the loss of muscle mass during weight loss (82). The effect of exercise on serum LDL-C varies with some studies showing a 4-7% decrease and some even showing increases (84,85). The decrease in LDL-C levels typically occurs in association with weight loss. However, the levels of small dense LDL decrease with exercise, while the levels of large LDL increase, an effect that occurs even in the absence of significant weight loss (85). To significantly increase HDL-C levels requires a considerable amount of exercise (700-2000kcal of exercise per week) (84,86). Serum TG levels are most responsive to exercise with various studies showing a 4-37% decrease in serum TG levels with exercise (mean decrease 24%) (84). Of note the changes in HDL-C and TG induced by exercise occur independent of weight loss (84). Resistance training alone has minimal effects on TG and HDL-C levels (87). It is recommended that patients exercise 150 minutes or more per week (for example 30 minutes 5x per week). The more intensive the exercise program the greater the effect on weight and lipid levels.
Effect of Weight Loss Drugs
There are several weight loss drugs currently approved for the long-term treatment of obesity. For a detailed discussion of weight loss drugs see the chapter on “Pharmacologic Treatment of Overweight and Obese Adults” (88). Weight loss drugs tend to decrease TG and LDL-C levels and increase HDL-C levels due to their ability to decrease weight but the results vary in individual patients.
ORLISTAT (XENICAL)
Orlistat is a lipase inhibitor that decreases fat absorption. Total cholesterol and LDL-C levels decrease with orlistat treatment to a greater degree than expected with diet alone (89-91). For example, in the XENDOS study LDL-C decreased by 12.8% in the orlistat group vs. 5.1% in the placebo group (89). Additionally, studies have shown that the levels of small dense LDL are reduced and the average LDL particle size increased with orlistat treatment (92). It has been shown that orlistat, in addition to reducing dietary TG absorption, also decreases cholesterol absorption (93). A likely mechanism for the decrease in cholesterol absorption is orlistat inhibition of NPC1L1, a transporter in the intestine that mediates cholesterol absorption (94). Despite the effect on TG absorption, orlistat does not markedly affect either fasting TG or HDL-C levels beyond what one would expect with weight loss (90,95). However, orlistat does reduce postprandial TG levels and has been used to treat patients with familial chylomicronemia syndrome (96).
PHENTERAMINE + TOPIRAMATE (QSYMIA)
Phenteramine is a sympathomimetic amine that induces satiety and topiramate is a neurostabilizer that also decreases appetite. In randomized controlled trials, phenteramine + topiramate combination therapy decreased TG levels and increased HDL-C without a consistent effect on LDL-C levels (97-99). It is likely that these changes primarily represent the effect of the weight loss induced by this drug.
NALTREXONE + BUPROPION (CONTRAVE)
Naltrexone is an opioid antagonist and bupropion is an antidepressant. In large randomized control trials naltrexone + bupropion decreased TG levels by approx. 8-12%, decreased LDL-C levels by 0-6%, and increased HDL-C by 3-8% (100-103). The magnitude of these changes in lipid levels mimics what one would expect from weight loss.
LIRAGLUTIDE (SAXENDA)
Liraglutide is a GLP-1 agonist that has been approved for the treatment of obesity. A large randomized trial demonstrated modest reductions in TG (9%) and LDL-C levels (2.4%) and increases in HDL-C (1.9%) with liraglutide treatment (104). Another randomized trial failed to demonstrate changes in lipid parameters (105). However, a trial in patients with diabetes also resulted in modest improvements in TG and HDL-C levels (106). Thus, liraglutide induces modest changes in the lipid profile that mimics what one observes with weight loss.
SEMAGLUTIDE (WEGOVY)
Several studies have determined the effect of saxagliptin on lipid levels during weight loss trials. In the STEP 1and 2 trials minimal decreases in LDL-C and increases in HDL-C were observed (107,108). However, a more marked decrease in TG were observed (107,108). In the STEP 3 trial LDL-C was decreased by 7mg/dL and TG by 17mg/dL while HDL-C was increased by 1.5mg/dL (109). In other studies it has been shown that GLP-1 receptor agonists reduce postprandial TGs by reducing circulating chylomicrons due to decreasing intestinal lipoprotein production (110).
SUMMARY OF WEIGHT LOSS DRUGS
Except for orlistat, which appears to lower LDL-C beyond what would be expected with weight loss alone, the effect of these weight loss drugs on fasting lipid levels seems to reflect their ability to induce weight loss. It should be noted that the change in lipid levels induced by weight loss drugs is modest, variable, and roughly correlates with the degree of weight loss.
Effect of Bariatric Surgery on Lipids
Bariatric surgery is more effective at inducing weight loss than either diet or medications (111,112). Associated with this greater decrease in weight is a more robust decrease in serum TG levels and increase in HDL-C levels (113-115). In some studies, a marked decrease in LDL-C is also observed. For example, Nguyen et al reported that in patients with severe obesity, Roux-en-Y gastric bypass (RYGB) resulted in a 63% decrease in serum TG, a 31% decrease in LDL-C, and a 39% increase in HDL-C (116). Studies have also shown a decrease in postprandial lipemia and Lp(a) levels (117-119). Moreover, the ability of HDL to mediate cholesterol efflux from cells is improved following bariatric surgery (120-122). Many patients are able to discontinue their lipid lowering drugs post bariatric surgery.
There are differences in the ability of different bariatric surgeries to impact lipids. Two randomized trials demonstrated a greater effect of RYGB compared to sleeve gastrectomy on dyslipidemia (123,124). In contrast, another randomized trial did not observe a difference in the effect of RYGB compared to sleeve gastrectomy on triglycerides, HDL-C, or LDL-C (125). However, in a meta-analysis comparing randomized trials of RNYGB vs. sleeve gastrectomy, Li et al reported that serum TG decreased to a greater degree in the RYGB patients (approximately 20mg/dL) (126). A similar greater decrease in LDL-C levels was also seen in the RYGB groups (approximately 28mg/dL). Puzziferri et al published a systemic review of the long-term follow-up of patients after bariatric surgery (127). They reported that the remission of hyperlipidemia (defined as total cholesterol < 200mg/dL, HDL-C > 40mg/dL, LDL-C < 160mg/dL, and TG < 200mg/dL) was 60.4% after RYGB but only 22.7% after gastric band. Thus, RYGB is the most effective procedure for reducing dyslipidemia in patients with obesity, followed by sleeve gastrectomy, followed by gastric banding (115). Whether the greater beneficial effects of RYGB on lipids is due to greater weight loss, endocrine changes, nutrient malabsorption, or enhanced bile-acid absorption and increases in circulating bile-acid levels induced by this procedure remains to be fully elucidated.
It should be noted that observational studies have found large reductions in cardiovascular events with bariatric surgery in both patients with and without pre-existing cardiovascular disease (128). It is likely that improvements in dyslipidemia contributes to this decrease in cardiovascular events.
Summary of Weight Loss Effect on Lipid Levels
A meta-analysis of 73 studies enrolling 32,496 patients examined the effect of weight loss due to diet, drugs, and bariatric surgery on lipid levels at 12 months (table 5) (58). One should recognize that there is quite a bit of variability and the results shown in table 5 only provide a rough estimate of the effect of weight loss in an individual patient with the different treatments.
Table 5.
Change in Lipid Levels with Weight Loss Interventions (mg/dL per kg weight loss)
Triglycerides | LDL Cholesterol | HDL Cholesterol | |
---|---|---|---|
Diet and/or Exercise | -4.00; 95% CI -5.24, -2.77 | -1.28; 95% CI -2.19, -0.37 | 0.46; 95% CI 0.19, 0.71 |
Weight Loss Drugs | -1.25; 95% CI -2.94, 0.43 | -1.67; 95% CI -2.28, -1.06 | 0.37; 95% CI 0.23, 0.52 |
Bariatric Surgery | -2.47, 95% CI -3.14, -1.80 | -0.33; 95% CI -0.77, 0.10 | 0.42; 95% CI 0.37, 0.47 |
Note- The decrease in LDL-C with weight loss drugs included a relatively large number of patients treated with orlistat, which may have enhanced the LDL-C reduction.
Effect of Lipid Lowering Drugs
In general, the effect of lipid lowering drugs in patients with obesity is similar to the effects observed in normal weight patients. Statins are the first line drug except in patients with very high TG levels (>500mg/dL) where fibrates, fish oil (omega-3-fatty acids), or niacin may be used initially to specifically target very high TG (>500-1000mg/dL). For additional information on lipid lowering drugs please see the chapters on cholesterol lowering drugs and TG lowering drugs (129,130). Below we address issues of using lipid lowering drugs that have importance specifically for patients who are obese.
STATINS
Statins are easy to use and generally well tolerated by patients who are obese. Statins can adversely affect glucose homeostasis. In non-diabetics the risk of developing diabetes is increased by approximately 10% with higher doses of statins causing a greater risk than more moderate doses (131,132). The mechanism for this adverse effect is unknown but a recent study suggests that decreases in HMG-coenzyme A activity leads to weight gain, which could increase the risk of developing diabetes (133). Older patients who are obese with higher baseline glucose levels are at greatest risk for developing diabetes on statin therapy.
Muscle symptoms occur in patients with obesity similar to what is observed in normal weight patients. One study has shown that the cardiorespiratory benefits of exercise were blunted in patients who are overweight or obese on statin therapy (134). However, a recent review concluded that statins do not consistently reduce muscle strength, endurance, or overall exercise performance (135).
Statin outcome trials have not specifically focused on the benefits of statins in patients with obesity. However, subgroup analysis of the statin trials has demonstrated that the beneficial effects also occur in patients who are obese. In the Cholesterol Clinical Trialists meta-analysis, the reduction in cardiovascular events was no different in patients with a BMI greater than 30 or less than 25 (136,137). Thus, one can anticipate that statin treatment will achieve the same beneficial outcomes in patients who are obese as seen in the general population.
BILE ACID SEQUESTRANTS
Bile acid sequestrants may increase serum TG levels, which can be a problem in some patients with obesity who are already hypertriglyceridemic (129). Colesevelam (Welchol) is a bile acid sequestrants that comes in pill or powder form that causes fewer side effects and has fewer interactions with other drugs than other preparations. Of particular note is that a number of studies have shown that colesevelam decreases A1c levels (approximately 0.5% decrease) and therefore this drug may have an added advantage in patients with obesity who are at high risk of developing diabetes (138).
NIACIN
Niacin reduces insulin sensitivity (i.e., causes insulin resistance), which can worsen glycemic control (139). In the HPS2-Thrive trial, niacin therapy induced new onset diabetes in subjects that were non-diabetic (140). In patients with obesity, who are at an increased risk of developing diabetes, niacin therapy increases the risk of these patients progressing to diabetes. Niacin can also increase serum uric acid levels and induce gout, an abnormality that is already common in patients with obesity (139).
PCSK9 INHIBITORS
In 2015 two monoclonal antibodies that inhibit PCSK9 (proprotein convertase subtilisin kexin type 9) were approved for the lowering of LDL cholesterol levels; Alirocumab (Praluent) and evolocumab (Repatha) (129). Inclisiran, small interfering RNA that stimulates the catalytic breakdown of PCSK9 mRNA, was approved in the US in 2021. The reduction in LDL cholesterol levels with PCSK9 inhibitor treatment is similar in obese and non-obese subjects and results in a 50-60% decrease in LDL cholesterol levels when added to statin therapy (129,141-143).
BEMPEDOIC ACID
Bempedoic acid was approved in the US in February 2020 and is an adenosine triphosphate-citrate lyase (ACL) inhibitor that decreases hepatic cholesterol synthesis (129). Patients with obesity often have elevated uric acid levels and an increased risk of gouty attacks and a major side effect of bempedoic acid is elevating uric acid levels (129). In clinical trials, 26% of bempedoic acid-treated patients with normal baseline uric acid values experienced hyperuricemia one or more times versus 9.5% in the placebo group (package insert). The increase in uric acid is due to bempedoic acid inhibiting renal tubular OAT2 (129). Elevations in blood uric acid levels may lead to the development of gout and gout was reported in 1.5% of patients treated with bempedoic acid vs. 0.4% of patients treated with placebo. The risk for gout attacks were higher in patients with a prior history of gout (11.2% for bempedoic acid treatment vs. 1.7% in the placebo group) (package insert). In patients with no prior history of gout only 1% of patients treated with bempedoic acid and 0.3% of the placebo group had a gouty attack (package insert).
EZETIMIBE, FIBRATES, AND OMEGA-3-FATTY ACIDS
These drugs are well tolerated in patients with obesity and their use in patients with obesity does not have any unique concerns.
TREATMENT APPROACH
The first priority in treating lipid disorders is to lower the LDL-C levels to goal, unless TG are markedly elevated (> 500-1000mg/dL), which increases the risk of pancreatitis. LDL-C is the first priority because the data linking lowering LDL-C with reducing cardiovascular disease are extremely strong and we now have the ability to markedly decrease LDL-C levels. Dietary therapy is the initial step but in the majority of patients’ dietary modifications will not be sufficient to achieve the LDL-C goals. If patients are willing and able to make major changes in their diet it is possible to achieve remarkable reductions in LDL-C levels but this seldom occurs in clinical practice.
Primary Prevention Patients
The first step is determining the risk for developing atherosclerotic cardiovascular disease. There are a number of different calculators for determining risk. In the US the most popular is the ACC/AHA risk calculator (http://www.cvriskcalculator.com/) whereas in Europe the SCORE (Systematic Coronary Risk Estimation) is popular (http://www.heartscore.org/en_GB/access). The ACC/AHA recommendations are shown in Figure 2 and the European Society of Cardiology/European Atherosclerosis Society (ESC/EAS) recommendations are shown in Figure 3 (45,47). Note that the risk shown in the ACC/AHA calculator is for major cardiovascular events while the risk in the SCORE calculator is for mortality.
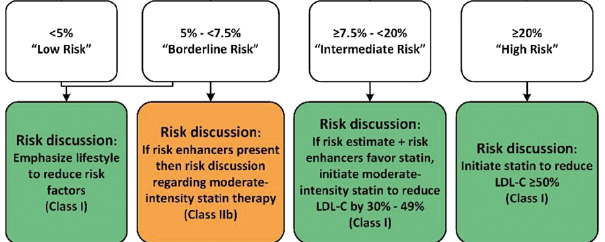
Figure 2.
ACC/AHA Recommendations for Patients without ASCVD, Diabetes, or LDL-C greater than 190mg/dL. Risk enhancers are listed in table 6. (Note the risk is for MI and stroke, both fatal and nonfatal).

Figure 3.
Table 6. ASCVD Risk Enhancers (ACC/AHA)
Family history of premature ASCVD Persistently elevated LDL > 160mg/dL Chronic kidney disease Metabolic syndrome History of preeclampsia History of premature menopause Inflammatory disease (especially rheumatoid arthritis, psoriasis, HIV) Ethnicity (e.g., South Asian ancestry) Persistently elevated TG > 175mg/dL Hs-CRP > 2mg/L Lp(a) > 50mg/dL or >125nmol/L Apo B > 130mg/dL Ankle-brachial index (ABI) < 0.9 |
Table 7.
Cardiovascular Risk Categories (ESC/EAS)
Very High Risk | ASCVD DM with target organ damage or at least three major risk factors or early onset of T1DM of long duration (>20 years) Severe CKD (eGFR <30 mL/min/1.73 m2) A calculated SCORE >10% for 10-year risk of fatal CVD FH with ASCVD or with another major risk factor |
High Risk | Markedly elevated single risk factors, in particular TC >310 mg/dL, LDL-C >190 mg/dL, or BP >180/110 mmHg Patients with FH without other major risk factors Patients with DM without target organ damage with DM duration >10 years or another additional risk factor Moderate CKD (eGFR 30-59 mL/min/1.73 m2). A calculated SCORE >5% and <10% for 10-year risk of fatal CVD |
Moderate Risk | Young patients (T1DM <35 years; T2DM <50 years) with DM duration <10 years, without other risk factors Calculated SCORE >1% and <5% for 10-year risk of fatal CVD. |
Low Risk | Calculated SCORE <1% for 10-year risk of fatal CVD |
Table 8.
Treatment Targets and Goals (ECS/EAS)
LDL-C | Non-HDL-C** | Apo B** | |
---|---|---|---|
Very High Risk | < 55mg/L; 1.4mmol/L* | < 85mg/dL; 2.2mmol/L | < 65mg/dL |
High Risk | < 70mg/dL; 1.8mmol/L* | < 100mg/dL; 2.6mmol/L | < 80mg/dL |
Moderate Risk | < 100mg/L; 2.6mmol/L | < 130mg/dL; 3.4mmol/L | < 100mg/dL |
Low Risk | < 116mg/dL; 3.0mmol/L |
- *
>50% LDL-C reduction from baseline; ** Secondary goals.
A few caveats are worth noting. First, in patients less than 60 years of age it is very helpful to calculate the life-time risk of ASCVD events. Often one will find that the 10-year risk is modest but the life-time risk is high and this information should be included in the risk discussion to help in the decision process. Second, patients should be made aware of the natural history of ASCVD and that it begins early in life and slowly progresses overtime with high LDL-C levels accelerating the rate of development of atherosclerosis and low LDL-C leading to a slower progression of atherosclerosis (144). Third, patients should be made aware of genetic studies demonstrating that variants in genes that lead to lifetime decreases in LDL-C levels (for example the HMG-CoA reductase gene, NPC1L1 gene, PCSK9 gene, ATP citrate lyase gene, and LDL receptor gene) result in a decreased risk of cardiovascular events. In a recent study it was reported that a 10mg/dL lifetime decrease in LDL-C with any of these genetic variants was associated with a 16-18% decrease in cardiovascular events whereas a 10mg/dL reduction in LDL-C with lipid lowering therapy later in life results in only approximately a 5% decrease in cardiovascular events ((145,146). The combination of the natural history and the results observed with genetic variants strongly suggests that early therapy to lower LDL-C levels will have greater effects on reducing the risk of ASCVD events than starting therapy later in life. This information needs to be discussed with the patient. Fourth, when patients and/or health care providers are uncertain of the best course of action obtaining a cardiac calcium scan can be very helpful in the decision-making process, particularly in older individuals (45). A score of 0, particularly in an older patient would indicate that statin therapy is not needed whereas a score > 100 would indicate a need for statin therapy. A score of 1-99 favors the use of a statin.
In most primary prevention patients, statin therapy is sufficient to lower LDL-C levels to goal (< 100mg/dL). One can usually start with moderate statin therapy (for example atorvastatin 10-20mg or rosuvastatin 5-10mg) and increase the statin dose, if necessary, to achieve LDL-C goals. In some instances, primary prevention patients are at high risk (see figures 2 and 3) and should be started on intensive statin therapy with lower LDL-C goals. Statins are available as generic drugs and therefore are relatively inexpensive. If a patient does not achieve their LDL-C goal on intensive statin therapy, cannot tolerate statin therapy, or is able to take only a low dose of a statin one can use ezetimibe (generic drug), bempedoic acid, bile acid sequestrant, or PCSK9 inhibitors to further lower LDL-C levels (for detailed discussion of cholesterol lowering drugs see (129)). It should be noted that the addition of ezetimibe or a PCSK9 inhibitor to statin therapy has been shown to reduce cardiovascular events (147-150). Bempedoic acid has been shown to reduce cardiovascular event in statin intolerant patients (151). In most situations, ezetimibe is the drug of choice given its low cost, ability to reduce ASCVD events, and long-term safety record.
Patients with Diabetes
Most patients with diabetes without risk factors should be started on moderate statin therapy (for example atorvastatin 10-20mg or rosuvastatin 5-10mg) and the dose increased as needed to reach therapy goals. Patients with diabetes with ASCVD or risk factors should be started on intensive statin therapy. In my opinion reasonable goals are shown in table 9 (similar to AACE and ECS/EAS guidelines) (47,49,152). If moderate statin therapy does not achieve the LDL-C goal the dose can be increased. If intensive therapy does not achieve LDL-C goals additional drugs can be added. If reasonably close to the LDL-C goal the initial drug added should be ezetimibe. If far from goal one could add a PCSK9 inhibitor. Once the LDL-C is at goal if the non-HDL-C remains high one can consider the approaches described in the section describing the approach to patients with LDL-C at goal and TG elevated.
Table 9.
ASCVD Risk Categories and Treatment Goals
Risk Category | Risk Factors/10-year risk | LDL-C mg/dL | Non-HDL-C mg/dL |
---|---|---|---|
Extreme Risk | Diabetes and clinical cardiovascular disease | <55 | <80 |
Very High Risk | Diabetes with one or more risk factors | <70 | <100 |
High Risk | Diabetes and no other risk factors | <100 | <130 |
Secondary Prevention Patients
Patients with ASCVD (secondary prevention patients) should be started on intensive statin therapy (atorvastatin 40-80mg per day or rosuvastatin 20-40mg per day). Given the extensive data showing that the lower the LDL-C the greater the reduction in ASCVD events most secondary prevention patients would benefit from the addition of ezetimibe to maximize LDL-C lowering without markedly increasing costs (146). The goal LDL-C in this patient population is an LDL<70mg/dL but many experts and some guidelines (ACCE, ECS/EAS) would prefer an LDL-C<55mg/dL if possible. If on intensive statin therapy and ezetimibe treatment the LDL-C is far above goal one could consider adding a PCSK9 inhibitor (this is particularly necessary if the LDL-C is greater than 100mg/dL or the patient is at very high risk due to other factors (diabetes, cerebral vascular disease, peripheral vascular disease, recent MI, history of multiple Mis, etc.)) (146).
Patients with LDL Cholesterol at Goal but High TG (>150mg/dL to <500mg/dL)
Patients with an LDL-C at goal but high TG levels (>150mg/dL to <500mg/dL) will often have increased non-HDL-C levels. Numerous studies have shown that the risk of ASCVD events is increased in this patient population (153). The initial step should be to improve lifestyle, treat secondary disorders that may be contributing to the increase in TG, and if possible, discontinue medications that increase TG levels (53). Studies have not demonstrated a reduction in cardiovascular events when niacin is added to statin therapy and given the side effects of niacin enthusiasm for using niacin in combination with statins to reduce ASCVD is limited (140,154). Similarly, the ACCORD-LIPID trial using fenofibrate and the PROMINENT trial using pemafibrate also failed to demonstrate that adding a fibrate to statin therapy reduces cardiovascular disease (155,156). Thus, there is no evidence that adding a fibrate to statin therapy in patients with high TG levels will reduce cardiovascular events. It should also be noted that in patients with diabetes fenofibrate reduces the development of diabetic microvascular disease (130).
Recently, the REDUCE-IT trial demonstrated that adding the omega-3-fatty acid icosapent ethyl (EPA; Vascepa) to statin therapy in patients with elevated TG levels reduced the risk of ASCVD events by 25% while decreasing TG levels by 18% (157). In this trial the reduction in TG levels was relatively modest and would not have been expected to result in the magnitude of the decrease in cardiovascular disease observed in the REDUCE-IT trial. Other actions of EPA, such as decreasing platelet function, anti-inflammation, decreasing lipid oxidation, stabilizing membranes, etc. could account for or contribute to the reduction in cardiovascular events (158). However, a very similar trial using a carboxylic acid formulation of EPA and DHA (STRENGTH Trial) while lowering the TG levels similar to that observed in the REDUCE-IT trial did not reduce cardiovascular events. This has led to controversy; does purified EPA have cardiovascular benefits that are not observed with the combination of DHA/EPA or was the use of mineral oil in the REDUCE-IT trial “toxic” increasing LDL-C levels and increasing inflammation? This controversy is discussed in another Endotext chapters (130) and in other publications (159,160). Ideally, this controversy will be resolved by a new cardiovascular outcome trial using icosapent ethyl without mineral oil serving as the placebo. In the meantime, in selected high-risk patients with LDL-C levels at goal and TG levels between 150-500mg/dL one can use icosapent ethyl therapy to reduce cardiovascular events.
Patients with Very High TG Levels (>500-1000mg/dL)
The main aim is to keep TG levels below 500 mg/dL to prevent TG-induced pancreatitis (161,162). Very high TG levels are frequently due to the coexistence of a genetic predisposition to hypertriglyceridemia with 1 or more secondary causes of hypertriglyceridemia (161,162). Initial treatment is a very low-fat diet to reduce TG levels into a safe range (<1000mg/dL). Treating secondary disorders that raise TG levels and when possible stopping drugs that increase TG levels is essential (161,162). Avoidance of simple sugars and ethanol is also indicated. If the TG levels remain above 500mg/dL the addition of fenofibrate or omega-3-fatty acids is indicated. Many patients with very high TG levels are at high risk for ASCVD and therefore after TG levels are controlled the patient should be evaluated for cardiovascular disease risk and if indicated LDL-C lowering therapy initiated.
Decreased HDL Cholesterol Levels
There are no studies demonstrating that increasing HDL-C levels reduces cardiovascular disease (163). It should be recognized that the crucial issue with HDL may not be the HDL-C levels per se but rather the function of the HDL particles (163). Assays have been developed to determine the ability of HDL to facilitate cholesterol efflux from macrophages and these studies have shown that the levels of HDL-C do not necessarily indicate the ability to mediate cholesterol efflux (164). Similarly, the ability of HDL to protect LDL from oxidation may also play an important role in the ability of HDL to reduce ASCVD (165). Thus, the functional capability of HDL may be more important than HDL-C levels (164,165).
SUMMARY
In summary, modern therapy of patients with obesity demands that we aggressively treat lipids to reduce the high risk of cardiovascular disease in this susceptible population and in those with very high TG to reduce the risk of pancreatitis.
REFERENCES
- 1.
- Flegal KM. Epidemiologic aspects of overweight and obesity in the United States. Physiol Behav. 2005;86:599–602. [PubMed: 16242735]
- 2.
- Purnell JQ. Definitions, Classification, and Epidemiology of Obesity. In: Feingold KR, Anawalt B, Boyce A, Chrousos G, Dungan K, Grossman A, Hershman JM, Kaltsas G, Koch C, Kopp P, Korbonits M, McLachlan R, Morley JE, New M, Perreault L, Purnell J, Rebar R, Singer F, Trence DL, Vinik A, Wilson DP, eds. Endotext. South Dartmouth (MA)2018.
- 3.
- Flegal KM, Kruszon-Moran D, Carroll MD, Fryar CD, Ogden CL. Trends in Obesity Among Adults in the United States, 2005 to 2014. JAMA. 2016;315:2284–2291. [PubMed: 27272580]
- 4.
- Hedley AA, Ogden CL, Johnson CL, Carroll MD, Curtin LR, Flegal KM. Prevalence of overweight and obesity among US children, adolescents, and adults, 1999-2002. JAMA. 2004;291:2847–2850. [PubMed: 15199035]
- 5.
- Collaborators GBDO, Afshin A, Forouzanfar MH, Reitsma MB, Sur P, Estep K, Lee A, Marczak L, Mokdad AH, Moradi-Lakeh M, Naghavi M, Salama JS, Vos T, Abate KH, Abbafati C, Ahmed MB, Al-Aly Z, Alkerwi A, Al-Raddadi R, Amare AT, Amberbir A, Amegah AK, Amini E, Amrock SM, Anjana RM, Arnlov J, Asayesh H, Banerjee A, Barac A, Baye E, Bennett DA, Beyene AS, Biadgilign S, Biryukov S, Bjertness E, Boneya DJ, Campos-Nonato I, Carrero JJ, Cecilio P, Cercy K, Ciobanu LG, Cornaby L, Damtew SA, Dandona L, Dandona R, Dharmaratne SD, Duncan BB, Eshrati B, Esteghamati A, Feigin VL, Fernandes JC, Furst T, Gebrehiwot TT, Gold A, Gona PN, Goto A, Habtewold TD, Hadush KT, Hafezi-Nejad N, Hay SI, Horino M, Islami F, Kamal R, Kasaeian A, Katikireddi SV, Kengne AP, Kesavachandran CN, Khader YS, Khang YH, Khubchandani J, Kim D, Kim YJ, Kinfu Y, Kosen S, Ku T, Defo BK, Kumar GA, Larson HJ, Leinsalu M, Liang X, Lim SS, Liu P, Lopez AD, Lozano R, Majeed A, Malekzadeh R, Malta DC, Mazidi M, McAlinden C, McGarvey ST, Mengistu DT, Mensah GA, Mensink GBM, Mezgebe HB, Mirrakhimov EM, Mueller UO, Noubiap JJ, Obermeyer CM, Ogbo FA, Owolabi MO, Patton GC, Pourmalek F, Qorbani M, Rafay A, Rai RK, Ranabhat CL, Reinig N, Safiri S, Salomon JA, Sanabria JR, Santos IS, Sartorius B, Sawhney M, Schmidhuber J, Schutte AE, Schmidt MI, Sepanlou SG, Shamsizadeh M, Sheikhbahaei S, Shin MJ, Shiri R, Shiue I, Roba HS, Silva DAS, Silverberg JI, Singh JA, Stranges S, Swaminathan S, Tabares-Seisdedos R, Tadese F, Tedla BA, Tegegne BS, Terkawi AS, Thakur JS, Tonelli M, Topor-Madry R, Tyrovolas S, Ukwaja KN, Uthman OA, Vaezghasemi M, Vasankari T, Vlassov VV, Vollset SE, Weiderpass E, Werdecker A, Wesana J, Westerman R, Yano Y, Yonemoto N, Yonga G, Zaidi Z, Zenebe ZM, Zipkin B, Murray CJL. Health Effects of Overweight and Obesity in 195 Countries over 25 Years. N Engl J Med. 2017;377:13–27. [PMC free article: PMC5477817] [PubMed: 28604169]
- 6.
- Finkelstein EA, Khavjou OA, Thompson H, Trogdon JG, Pan L, Sherry B, Dietz W. Obesity and severe obesity forecasts through 2030. Am J Prev Med. 2012;42:563–570. [PubMed: 22608371]
- 7.
- Kapoor N. Thin Fat Obesity: The Tropical Phenotype of Obesity. In: Feingold KR, Anawalt B, Blackman MR, Boyce A, Chrousos G, Corpas E, de Herder WW, Dhatariya K, Dungan K, Hofland J, Kalra S, Kaltsas G, Kapoor N, Koch C, Kopp P, Korbonits M, Kovacs CS, Kuohung W, Laferrere B, Levy M, McGee EA, McLachlan R, New M, Purnell J, Sahay R, Singer F, Sperling MA, Stratakis CA, Trence DL, Wilson DP, eds. Endotext. South Dartmouth (MA)2021.
- 8.
- Kumar S, Kelly AS. Review of Childhood Obesity: From Epidemiology, Etiology, and Comorbidities to Clinical Assessment and Treatment. Mayo Clin Proc. 2017;92:251–265. [PubMed: 28065514]
- 9.
- Bays HE, Toth PP, Kris-Etherton PM, Abate N, Aronne LJ, Brown WV, Gonzalez-Campoy JM, Jones SR, Kumar R, La Forge R, Samuel VT. Obesity, adiposity, and dyslipidemia: a consensus statement from the National Lipid Association. J Clin Lipidol. 2013;7:304–383. [PubMed: 23890517]
- 10.
- Grundy SM. Obesity, metabolic syndrome, and cardiovascular disease. J Clin Endocrinol Metab. 2004;89:2595–2600. [PubMed: 15181029]
- 11.
- Kyrou I, Randeva HS, Tsigos C, Kaltsas G, Weickert MO. Clinical Problems Caused by Obesity. In: Feingold KR, Anawalt B, Boyce A, Chrousos G, Dungan K, Grossman A, Hershman JM, Kaltsas G, Koch C, Kopp P, Korbonits M, McLachlan R, Morley JE, New M, Perreault L, Purnell J, Rebar R, Singer F, Trence DL, Vinik A, Wilson DP, eds. Endotext. South Dartmouth (MA)2018.
- 12.
- Mandviwala T, Khalid U, Deswal A. Obesity and Cardiovascular Disease: a Risk Factor or a Risk Marker? Curr Atheroscler Rep. 2016;18:21. [PubMed: 26973130]
- 13.
- Zalesin KC, Franklin BA, Miller WM, Peterson ED, McCullough PA. Impact of obesity on cardiovascular disease. Med Clin North Am. 2011;95:919–937. [PubMed: 21855700]
- 14.
- Franssen R, Monajemi H, Stroes ES, Kastelein JJ. Obesity and dyslipidemia. Med Clin North Am. 2011;95:893–902. [PubMed: 21855698]
- 15.
- Xiao C, Dash S, Morgantini C, Hegele RA, Lewis GF. Pharmacological Targeting of the Atherogenic Dyslipidemia Complex: The Next Frontier in CVD Prevention Beyond Lowering LDL Cholesterol. Diabetes. 2016;65:1767–1778. [PubMed: 27329952]
- 16.
- Berneis KK, Krauss RM. Metabolic origins and clinical significance of LDL heterogeneity. J Lipid Res. 2002;43:1363–1379. [PubMed: 12235168]
- 17.
- Masuda D, Yamashita S. Postprandial Hyperlipidemia and Remnant Lipoproteins. J Atheroscler Thromb. 2017;24:95–109. [PMC free article: PMC5305681] [PubMed: 27829582]
- 18.
- Klop B, Elte JW, Cabezas MC. Dyslipidemia in obesity: mechanisms and potential targets. Nutrients. 2013;5:1218–1240. [PMC free article: PMC3705344] [PubMed: 23584084]
- 19.
- Skinner AC, Perrin EM, Moss LA, Skelton JA. Cardiometabolic Risks and Severity of Obesity in Children and Young Adults. N Engl J Med. 2015;373:1307–1317. [PubMed: 26422721]
- 20.
- Cameron AJ, Magliano DJ, Soderberg S. A systematic review of the impact of including both waist and hip circumference in risk models for cardiovascular diseases, diabetes and mortality. Obes Rev. 2013;14:86–94. [PubMed: 23072327]
- 21.
- Grundy SM. Adipose tissue and metabolic syndrome: too much, too little or neither. Eur J Clin Invest. 2015;45:1209–1217. [PMC free article: PMC5049481] [PubMed: 26291691]
- 22.
- Jensen MD. Role of body fat distribution and the metabolic complications of obesity. J Clin Endocrinol Metab. 2008;93:S57–63. [PMC free article: PMC2585758] [PubMed: 18987271]
- 23.
- Snijder MB, Zimmet PZ, Visser M, Dekker JM, Seidell JC, Shaw JE. Independent and opposite associations of waist and hip circumferences with diabetes, hypertension and dyslipidemia: the AusDiab Study. Int J Obes Relat Metab Disord. 2004;28:402–409. [PubMed: 14724659]
- 24.
- Wohl D, Scherzer R, Heymsfield S, Simberkoff M, Sidney S, Bacchetti P, Grunfeld C, Investigators FS. The associations of regional adipose tissue with lipid and lipoprotein levels in HIV-infected men. J Acquir Immune Defic Syndr. 2008;48:44–52. [PMC free article: PMC3156607] [PubMed: 18360291]
- 25.
- Bjornson E, Adiels M, Taskinen MR, Boren J. Kinetics of plasma triglycerides in abdominal obesity. Curr Opin Lipidol. 2017;28:11–18. [PubMed: 27898581]
- 26.
- Yu YH, Ginsberg HN. Adipocyte signaling and lipid homeostasis: sequelae of insulin-resistant adipose tissue. Circ Res. 2005;96:1042–1052. [PubMed: 15920027]
- 27.
- Jacome-Sosa MM, Parks EJ. Fatty acid sources and their fluxes as they contribute to plasma triglyceride concentrations and fatty liver in humans. Curr Opin Lipidol. 2014;25:213–220. [PubMed: 24785962]
- 28.
- Li S, Brown MS, Goldstein JL. Bifurcation of insulin signaling pathway in rat liver: mTORC1 required for stimulation of lipogenesis, but not inhibition of gluconeogenesis. Proc Natl Acad Sci U S A. 2010;107:3441–3446. [PMC free article: PMC2840492] [PubMed: 20133650]
- 29.
- Dash S, Xiao C, Morgantini C, Lewis GF. New Insights into the Regulation of Chylomicron Production. Annu Rev Nutr. 2015;35:265–294. [PubMed: 25974693]
- 30.
- Chan DC, Watts GF, Redgrave TG, Mori TA, Barrett PH. Apolipoprotein B-100 kinetics in visceral obesity: associations with plasma apolipoprotein C-III concentration. Metabolism. 2002;51:1041–1046. [PubMed: 12145779]
- 31.
- Feingold KR, Grunfeld C. Introduction to Lipids and Lipoproteins. In: Feingold KR, Anawalt B, Boyce A, Chrousos G, Dungan K, Grossman A, Hershman JM, Kaltsas G, Koch C, Kopp P, Korbonits M, McLachlan R, Morley JE, New M, Perreault L, Purnell J, Rebar R, Singer F, Trence DL, Vinik A, Wilson DP, eds. Endotext. South Dartmouth (MA)2018.
- 32.
- Jorgensen AB, Frikke-Schmidt R, Nordestgaard BG, Tybjaerg-Hansen A. Loss-of-function mutations in APOC3 and risk of ischemic vascular disease. N Engl J Med. 2014;371:32–41. [PubMed: 24941082]
- 33.
- Tg, Hdl Working Group of the Exome Sequencing Project NHL, Blood I, Crosby J, Peloso GM, Auer PL, Crosslin DR, Stitziel NO, Lange LA, Lu Y, Tang ZZ, Zhang H, Hindy G, Masca N, Stirrups K, Kanoni S, Do R, Jun G, Hu Y, Kang HM, Xue C, Goel A, Farrall M, Duga S, Merlini PA, Asselta R, Girelli D, Olivieri O, Martinelli N, Yin W, Reilly D, Speliotes E, Fox CS, Hveem K, Holmen OL, Nikpay M, Farlow DN, Assimes TL, Franceschini N, Robinson J, North KE, Martin LW, DePristo M, Gupta N, Escher SA, Jansson JH, Van Zuydam N, Palmer CN, Wareham N, Koch W, Meitinger T, Peters A, Lieb W, Erbel R, Konig IR, Kruppa J, Degenhardt F, Gottesman O, Bottinger EP, O'Donnell CJ, Psaty BM, Ballantyne CM, Abecasis G, Ordovas JM, Melander O, Watkins H, Orho-Melander M, Ardissino D, Loos RJ, McPherson R, Willer CJ, Erdmann J, Hall AS, Samani NJ, Deloukas P, Schunkert H, Wilson JG, Kooperberg C, Rich SS, Tracy RP, Lin DY, Altshuler D, Gabriel S, Nickerson DA, Jarvik GP, Cupples LA, Reiner AP, Boerwinkle E, Kathiresan S. Loss-of-function mutations in APOC3, triglycerides, and coronary disease. N Engl J Med. 2014;371:22–31. [PMC free article: PMC4180269] [PubMed: 24941081]
- 34.
- Wulff AB, Nordestgaard BG, Tybjaerg-Hansen A. APOC3 Loss-of-Function Mutations, Remnant Cholesterol, Low-Density Lipoprotein Cholesterol, and Cardiovascular Risk: Mediation- and Meta-Analyses of 137 895 Individuals. Arterioscler Thromb Vasc Biol. 2018;38:660–668. [PubMed: 29348120]
- 35.
- Gaudet D, Brisson D, Tremblay K, Alexander VJ, Singleton W, Hughes SG, Geary RS, Baker BF, Graham MJ, Crooke RM, Witztum JL. Targeting APOC3 in the familial chylomicronemia syndrome. N Engl J Med. 2014;371:2200–2206. [PubMed: 25470695]
- 36.
- Feingold KR, Grunfeld C. The Effect of Inflammation and Infection on Lipids and Lipoproteins. In: Feingold KR, Anawalt B, Boyce A, Chrousos G, Dungan K, Grossman A, Hershman JM, Kaltsas G, Koch C, Kopp P, Korbonits M, McLachlan R, Morley JE, New M, Perreault L, Purnell J, Rebar R, Singer F, Trence DL, Vinik A, Wilson DP, eds. Endotext. South Dartmouth (MA)2019.
- 37.
- Khovidhunkit W, Kim MS, Memon RA, Shigenaga JK, Moser AH, Feingold KR, Grunfeld C. Effects of infection and inflammation on lipid and lipoprotein metabolism: mechanisms and consequences to the host. J Lipid Res. 2004;45:1169–1196. [PubMed: 15102878]
- 38.
- Lara-Castro C, Fu Y, Chung BH, Garvey WT. Adiponectin and the metabolic syndrome: mechanisms mediating risk for metabolic and cardiovascular disease. Curr Opin Lipidol. 2007;18:263–270. [PubMed: 17495599]
- 39.
- Christou GA, Kiortsis DN. Adiponectin and lipoprotein metabolism. Obes Rev. 2013;14:939–949. [PubMed: 23957239]
- 40.
- Rashid S, Kastelein JJ. PCSK9 and resistin at the crossroads of the atherogenic dyslipidemia. Expert Rev Cardiovasc Ther. 2013;11:1567–1577. [PubMed: 24134510]
- 41.
- Lu B, Moser A, Shigenaga JK, Grunfeld C, Feingold KR. The acute phase response stimulates the expression of angiopoietin like protein 4. Biochem Biophys Res Commun. 2010;391:1737–1741. [PubMed: 20043872]
- 42.
- Feingold KR, Grunfeld C. The acute phase response inhibits reverse cholesterol transport. J Lipid Res. 2010;51:682–684. [PMC free article: PMC2842157] [PubMed: 20071695]
- 43.
- Feingold KR, Grunfeld C. Effect of inflammation on HDL structure and function. Curr Opin Lipidol. 2016;27:521–530. [PubMed: 27495134]
- 44.
- Expert Panel on D. An International Atherosclerosis Society position paper: global recommendations for the management of dyslipidemia: executive summary. Atherosclerosis. 2014;232:410–413. [PubMed: 24468156]
- 45.
- Grundy SM, Stone NJ, Bailey AL, Beam C, Birtcher KK, Blumenthal RS, Braun LT, de Ferranti S, Faiella-Tommasino J, Forman DE, Goldberg R, Heidenreich PA, Hlatky MA, Jones DW, Lloyd-Jones D, Lopez-Pajares N, Ndumele CE, Orringer CE, Peralta CA, Saseen JJ, Smith SC Jr, Sperling L, Virani SS, Yeboah J. 2018 AHA/ACC/AACVPR/AAPA/ABC/ACPM/ADA/AGS/APhA/ASPC/NLA/PCNA Guideline on the Management of Blood Cholesterol: A Report of the American College of Cardiology/American Heart Association Task Force on Clinical Practice Guidelines. Circulation. 2019;139:e1082–e1143. [PMC free article: PMC7403606] [PubMed: 30586774]
- 46.
- Jellinger PS, Handelsman Y, Rosenblit PD, Bloomgarden ZT, Fonseca VA, Garber AJ, Grunberger G, Guerin CK, Bell DSH, Mechanick JI, Pessah-Pollack R, Wyne K, Smith D, Brinton EA, Fazio S, Davidson M. American Association of Clinical Endocrinologists and American College of Endocrinology Guidelines for Management of Dyslipidemia and Prevention of Cardiovascular Disease. Endocr Pract. 2017;23:1–87. [PubMed: 28437620]
- 47.
- Mach F, Baigent C, Catapano AL, Koskinas KC, Casula M, Badimon L, Chapman MJ, De Backer GG, Delgado V, Ference BA, Graham IM, Halliday A, Landmesser U, Mihaylova B, Pedersen TR, Riccardi G, Richter DJ, Sabatine MS, Taskinen MR, Tokgozoglu L, Wiklund O, Group ESCSD. 2019 ESC/EAS Guidelines for the management of dyslipidaemias: lipid modification to reduce cardiovascular risk. Eur Heart J. 2020;41:111–188. [PubMed: 31504418]
- 48.
- Jacobson TA, Ito MK, Maki KC, Orringer CE, Bays HE, Jones PH, McKenney JM, Grundy SM, Gill EA, Wild RA, Wilson DP, Brown WV. National Lipid Association recommendations for patient-centered management of dyslipidemia: part 1 - executive summary. J Clin Lipidol. 2014;8:473–488. [PubMed: 25234560]
- 49.
- Handelsman Y JP, Guerin CK, Bloomgarden ZT, Brinton EA, Budoff MJ, Davidson MH, Einhorn D, Fazio S, Fonseca VA, Garber AJ, Grunberger G, Krauss RM, Mechanick JI, Rosenblit PD, Smith DA, Wyne KL. CONSENSUS STATEMENT BY THE AMERICAN ASSOCIATION OF CLINICAL ENDOCRINOLOGISTS AND AMERICAN COLLEGE OF ENDOCRINOLOGY ON THE MANAGEMENT OF DYSLIPIDEMIA AND PREVENTION OF CARDIOVASCULAR DISEASE ALGORITHM – 2020 EXECUTIVE SUMMARY. Endocrine Practice. 2020;26:1–29. [PubMed: 33471721]
- 50.
- Grundy SM, Feingold KR. Guidelines for the Management of High Blood Cholesterol. In: Feingold KR, Anawalt B, Boyce A, Chrousos G, Dungan K, Grossman A, Hershman JM, Kaltsas G, Koch C, Kopp P, Korbonits M, McLachlan R, Morley JE, New M, Perreault L, Purnell J, Rebar R, Singer F, Trence DL, Vinik A, Wilson DP, eds. Endotext. South Dartmouth (MA)2020.
- 51.
- Bhatt D, Tannock L. Risk of Fasting and Non-Fasting Hypertriglyceridemia in Coronary Vascular Disease and Pancreatitis. In: Feingold KR, Anawalt B, Boyce A, Chrousos G, Dungan K, Grossman A, Hershman JM, Kaltsas G, Koch C, Kopp P, Korbonits M, McLachlan R, Morley JE, New M, Perreault L, Purnell J, Rebar R, Singer F, Trence DL, Vinik A, Wilson DP, eds. Endotext. South Dartmouth (MA)2018.
- 52.
- Berglund L, Brunzell JD, Goldberg AC, Goldberg IJ, Sacks F, Murad MH, Stalenhoef AF. Endocrine s. Evaluation and treatment of hypertriglyceridemia: an Endocrine Society clinical practice guideline. J Clin Endocrinol Metab. 2012;97:2969–2989. [PMC free article: PMC3431581] [PubMed: 22962670]
- 53.
- Feingold KR. Approach to the Patient with Dyslipidemia. In: Feingold KR, Anawalt B, Boyce A, Chrousos G, Dungan K, Grossman A, Hershman JM, Kaltsas G, Koch C, Kopp P, Korbonits M, McLachlan R, Morley JE, New M, Perreault L, Purnell J, Rebar R, Singer F, Trence DL, Vinik A, Wilson DP, eds. Endotext. South Dartmouth (MA)2020.
- 54.
- Feingold KR. The Effect of Diet on Cardiovascular Disease and Lipid and Lipoprotein Levels. In: Feingold KR, Anawalt B, Blackman MR, Boyce A, Chrousos G, Corpas E, de Herder WW, Dhatariya K, Dungan K, Hofland J, Kalra S, Kaltsas G, Kapoor N, Koch C, Kopp P, Korbonits M, Kovacs CS, Kuohung W, Laferrere B, Levy M, McGee EA, McLachlan R, New M, Purnell J, Sahay R, Singer F, Sperling MA, Stratakis CA, Trence DL, Wilson DP, eds. Endotext. South Dartmouth (MA)2021.
- 55.
- Dwyer JT, Melanson KJ, Sriprachy-anunt U, Cross P, Wilson M. Dietary Treatment of Obesity. In: Feingold KR, Anawalt B, Boyce A, Chrousos G, Dungan K, Grossman A, Hershman JM, Kaltsas G, Koch C, Kopp P, Korbonits M, McLachlan R, Morley JE, New M, Perreault L, Purnell J, Rebar R, Singer F, Trence DL, Vinik A, Wilson DP, eds. Endotext. South Dartmouth (MA)2015.
- 56.
- Dattilo AM, Kris-Etherton PM. Effects of weight reduction on blood lipids and lipoproteins: a meta-analysis. Am J Clin Nutr. 1992;56:320–328. [PubMed: 1386186]
- 57.
- Zomer E, Gurusamy K, Leach R, Trimmer C, Lobstein T, Morris S, James WP, Finer N. Interventions that cause weight loss and the impact on cardiovascular risk factors: a systematic review and meta-analysis. Obes Rev. 2016;17:1001–1011. [PubMed: 27324830]
- 58.
- Hasan B, Nayfeh T, Alzuabi M, Wang Z, Kuchkuntla AR, Prokop LJ, Newman CB, Murad MH, Rajjo TI. Weight Loss and Serum Lipids in Overweight and Obese Adults: A Systematic Review and Meta-Analysis. J Clin Endocrinol Metab. 2020:105. [PubMed: 32954416]
- 59.
- Unick JL, Beavers D, Bond DS, Clark JM, Jakicic JM, Kitabchi AE, Knowler WC, Wadden TA, Wagenknecht LE, Wing RR, Look ARG. The long-term effectiveness of a lifestyle intervention in severely obese individuals. Am J Med 2013; 126:236-242, 242 e231-232. [PMC free article: PMC3574274] [PubMed: 23410564]
- 60.
- Stelmach-Mardas M, Walkowiak J. Dietary Interventions and Changes in Cardio-Metabolic Parameters in Metabolically Healthy Obese Subjects: A Systematic Review with Meta-Analysis. Nutrients. 2016:8. [PMC free article: PMC4997370] [PubMed: 27483307]
- 61.
- Rajjo T, Almasri J, Al Nofal A, Farah W, Alsawas M, Ahmed AT, Mohammed K, Kanwar A, Asi N, Wang Z, Prokop LJ, Murad MH. The Association of Weight Loss and Cardiometabolic Outcomes in Obese Children: Systematic Review and Meta-regression. J Clin Endocrinol Metab. 2016;101:4764–4768. [PubMed: 27603909]
- 62.
- Sacks FM, Bray GA, Carey VJ, Smith SR, Ryan DH, Anton SD, McManus K, Champagne CM, Bishop LM, Laranjo N, Leboff MS, Rood JC, de Jonge L, Greenway FL, Loria CM, Obarzanek E, Williamson DA. Comparison of weight-loss diets with different compositions of fat, protein, and carbohydrates. N Engl J Med. 2009;360:859–873. [PMC free article: PMC2763382] [PubMed: 19246357]
- 63.
- Foster GD, Wyatt HR, Hill JO, Makris AP, Rosenbaum DL, Brill C, Stein RI, Mohammed BS, Miller B, Rader DJ, Zemel B, Wadden TA, Tenhave T, Newcomb CW, Klein S. Weight and metabolic outcomes after 2 years on a low-carbohydrate versus low-fat diet: a randomized trial. Ann Intern Med. 2010;153:147–157. [PMC free article: PMC2949959] [PubMed: 20679559]
- 64.
- Hu T, Mills KT, Yao L, Demanelis K, Eloustaz M, Yancy WS Jr, Kelly TN, He J, Bazzano LA. Effects of low-carbohydrate diets versus low-fat diets on metabolic risk factors: a meta-analysis of randomized controlled clinical trials. Am J Epidemiol. 2012;176 Suppl 7:S44–54. [PMC free article: PMC3530364] [PubMed: 23035144]
- 65.
- Johnston BC, Kanters S, Bandayrel K, Wu P, Naji F, Siemieniuk RA, Ball GD, Busse JW, Thorlund K, Guyatt G, Jansen JP, Mills EJ. Comparison of weight loss among named diet programs in overweight and obese adults: a meta-analysis. JAMA. 2014;312:923–933. [PubMed: 25182101]
- 66.
- Mansoor N, Vinknes KJ, Veierod MB, Retterstol K. Effects of low-carbohydrate diets v. low-fat diets on body weight and cardiovascular risk factors: a meta-analysis of randomised controlled trials. Br J Nutr. 2016;115:466–479. [PubMed: 26768850]
- 67.
- Naude CE, Schoonees A, Senekal M, Young T, Garner P, Volmink J. Low carbohydrate versus isoenergetic balanced diets for reducing weight and cardiovascular risk: a systematic review and meta-analysis. PLoS One. 2014;9:e100652. [PMC free article: PMC4090010] [PubMed: 25007189]
- 68.
- Schwingshackl L, Hoffmann G. Comparison of effects of long-term low-fat vs high-fat diets on blood lipid levels in overweight or obese patients: a systematic review and meta-analysis. J Acad Nutr Diet. 2013;113:1640–1661. [PubMed: 24139973]
- 69.
- Bueno NB, de Melo IS, de Oliveira SL, da Rocha Ataide T. Very-low-carbohydrate ketogenic diet v. low-fat diet for long-term weight loss: a meta-analysis of randomised controlled trials. Br J Nutr. 2013;110:1178–1187. [PubMed: 23651522]
- 70.
- Norwitz NG, Feldman D, Soto-Mota A, Kalayjian T, Ludwig DS. Elevated LDL Cholesterol with a Carbohydrate-Restricted Diet: Evidence for a "Lean Mass Hyper-Responder" Phenotype. Curr Dev Nutr 2022; 6:nzab144. [PMC free article: PMC8796252] [PubMed: 35106434]
- 71.
- Norwitz NG, Mindrum MR, Giral P, Kontush A, Soto-Mota A, Wood TR, D'Agostino DP, Manubolu VS, Budoff M, Krauss RM. Elevated LDL-cholesterol levels among lean mass hyper-responders on low-carbohydrate ketogenic diets deserve urgent clinical attention and further research. J Clin Lipidol. 2022;16:765–768. [PubMed: 36351849]
- 72.
- Houttu V, Grefhorst A, Cohn DM, Levels JHM, Roeters van Lennep J, Stroes ESG, Groen AK, Tromp TR. Severe Dyslipidemia Mimicking Familial Hypercholesterolemia Induced by High-Fat, Low-Carbohydrate Diets: A Critical Review. Nutrients. 2023:15. [PMC free article: PMC9964047] [PubMed: 36839320]
- 73.
- Goldberg IJ, Ibrahim N, Bredefeld C, Foo S, Lim V, Gutman D, Huggins LA, Hegele RA. Ketogenic diets, not for everyone. J Clin Lipidol. 2021;15:61–67. [PMC free article: PMC7887024] [PubMed: 33191194]
- 74.
- Schaffer AE, D'Alessio DA, Guyton JR. Extreme elevations of low-density lipoprotein cholesterol with very low carbohydrate, high fat diets. J Clin Lipidol. 2021;15:525–526. [PubMed: 34030981]
- 75.
- Schmidt T, Harmon DM, Kludtke E, Mickow A, Simha V, Kopecky S. Dramatic elevation of LDL cholesterol from ketogenic-dieting: A Case Series. Am J Prev Cardiol. 2023;14:100495. [PMC free article: PMC10121782] [PubMed: 37096158]
- 76.
- Wycherley TP, Moran LJ, Clifton PM, Noakes M, Brinkworth GD. Effects of energy-restricted high-protein, low-fat compared with standard-protein, low-fat diets: a meta-analysis of randomized controlled trials. Am J Clin Nutr. 2012;96:1281–1298. [PubMed: 23097268]
- 77.
- Schwingshackl L, Hoffmann G. Long-term effects of low-fat diets either low or high in protein on cardiovascular and metabolic risk factors: a systematic review and meta-analysis. Nutr J. 2013;12:48. [PMC free article: PMC3636027] [PubMed: 23587198]
- 78.
- Ge L, Sadeghirad B, Ball GDC, da Costa BR, Hitchcock CL, Svendrovski A, Kiflen R, Quadri K, Kwon HY, Karamouzian M, Adams-Webber T, Ahmed W, Damanhoury S, Zeraatkar D, Nikolakopoulou A, Tsuyuki RT, Tian J, Yang K, Guyatt GH, Johnston BC. Comparison of dietary macronutrient patterns of 14 popular named dietary programmes for weight and cardiovascular risk factor reduction in adults: systematic review and network meta-analysis of randomised trials. BMJ. 2020;369:m696. [PMC free article: PMC7190064] [PubMed: 32238384]
- 79.
- Dansinger ML, Gleason JA, Griffith JL, Selker HP, Schaefer EJ. Comparison of the Atkins, Ornish, Weight Watchers, and Zone diets for weight loss and heart disease risk reduction: a randomized trial. JAMA. 2005;293:43–53. [PubMed: 15632335]
- 80.
- Goff LM, Cowland DE, Hooper L, Frost GS. Low glycaemic index diets and blood lipids: a systematic review and meta-analysis of randomised controlled trials. Nutr Metab Cardiovasc Dis. 2013;23:1–10. [PubMed: 22841185]
- 81.
- Sacks FM, Carey VJ, Anderson CA, Miller ER 3rd, Copeland T, Charleston J, Harshfield BJ, Laranjo N, McCarron P, Swain J, White K, Yee K, Appel LJ. Effects of high vs low glycemic index of dietary carbohydrate on cardiovascular disease risk factors and insulin sensitivity: the OmniCarb randomized clinical trial. JAMA. 2014;312:2531–2541. [PMC free article: PMC4370345] [PubMed: 25514303]
- 82.
- DiPietro L, Stachenfeld NS. Exercise Treatment of Obesity. In: Feingold KR, Anawalt B, Boyce A, Chrousos G, Dungan K, Grossman A, Hershman JM, Kaltsas G, Koch C, Kopp P, Korbonits M, McLachlan R, Morley JE, New M, Perreault L, Purnell J, Rebar R, Singer F, Trence DL, Vinik A, Wilson DP, eds. Endotext. South Dartmouth (MA)2015.
- 83.
- Shaw K, Gennat H, O'Rourke P, Del Mar C. Exercise for overweight or obesity. Cochrane Database Syst Rev. 2006:CD003817. [PMC free article: PMC9017288] [PubMed: 17054187]
- 84.
- Ahmed HM, Blaha MJ, Nasir K, Rivera JJ, Blumenthal RS. Effects of physical activity on cardiovascular disease. Am J Cardiol. 2012;109:288–295. [PubMed: 22011559]
- 85.
- Sarzynski MA, Burton J, Rankinen T, Blair SN, Church TS, Despres JP, Hagberg JM, Landers-Ramos R, Leon AS, Mikus CR, Rao DC, Seip RL, Skinner JS, Slentz CA, Thompson PD, Wilund KR, Kraus WE, Bouchard C. The effects of exercise on the lipoprotein subclass profile: A meta-analysis of 10 interventions. Atherosclerosis. 2015;243:364–372. [PMC free article: PMC4663138] [PubMed: 26520888]
- 86.
- Kodama S, Tanaka S, Saito K, Shu M, Sone Y, Onitake F, Suzuki E, Shimano H, Yamamoto S, Kondo K, Ohashi Y, Yamada N, Sone H. Effect of aerobic exercise training on serum levels of high-density lipoprotein cholesterol: a meta-analysis. Arch Intern Med. 2007;167:999–1008. [PubMed: 17533202]
- 87.
- Lemes IR, Ferreira PH, Linares SN, Machado AF, Pastre CM, Netto JJ. Resistance training reduces systolic blood pressure in metabolic syndrome: a systematic review and meta-analysis of randomised controlled trials. Br J Sports Med. 2016;50:1438–1442. [PMC free article: PMC5136729] [PubMed: 26964146]
- 88.
- Tchang BG, Kumar RB, Aronne LJ. Pharmacologic Treatment of Overweight and Obesity in Adults. In: Feingold KR, Anawalt B, Boyce A, Chrousos G, de Herder WW, Dungan K, Grossman A, Hershman JM, Hofland HJ, Kaltsas G, Koch C, Kopp P, Korbonits M, McLachlan R, Morley JE, New M, Purnell J, Singer F, Stratakis CA, Trence DL, Wilson DP, eds. Endotext. South Dartmouth (MA)2020.
- 89.
- Sjostrom L, Rissanen A, Andersen T, Boldrin M, Golay A, Koppeschaar HP, Krempf M. Randomised placebo-controlled trial of orlistat for weight loss and prevention of weight regain in obese patients. European Multicentre Orlistat Study Group. Lancet. 1998;352:167–172. [PubMed: 9683204]
- 90.
- Swinburn BA, Carey D, Hills AP, Hooper M, Marks S, Proietto J, Strauss BJ, Sullivan D, Welborn TA, Caterson ID. Effect of orlistat on cardiovascular disease risk in obese adults. Diabetes Obes Metab. 2005;7:254–262. [PubMed: 15811142]
- 91.
- Mannucci E, Dicembrini I, Rotella F, Rotella CM. Orlistat and sibutramine beyond weight loss. Nutr Metab Cardiovasc Dis. 2008;18:342–348. [PubMed: 17928208]
- 92.
- Nakou ES, Filippatos TD, Georgoula M, Kiortsis DN, Tselepis AD, Mikhailidis DP, Elisaf MS. The effect of orlistat and ezetimibe, alone or in combination, on serum LDL and small dense LDL cholesterol levels in overweight and obese patients with hypercholesterolaemia. Curr Med Res Opin. 2008;24:1919–1929. [PubMed: 18513463]
- 93.
- Mittendorfer B, Ostlund RE Jr, Patterson BW, Klein S. Orlistat inhibits dietary cholesterol absorption. Obes Res. 2001;9:599–604. [PubMed: 11595776]
- 94.
- Alqahtani S, Qosa H, Primeaux B, Kaddoumi A. Orlistat limits cholesterol intestinal absorption by Niemann-pick C1-like 1 (NPC1L1) inhibition. Eur J Pharmacol. 2015;762:263–269. [PubMed: 26048312]
- 95.
- Sahebkar A, Simental-Mendia LE, Reiner Z, Kovanen PT, Simental-Mendia M, Bianconi V, Pirro M. Effect of orlistat on plasma lipids and body weight: A systematic review and meta-analysis of 33 randomized controlled trials. Pharmacol Res. 2017;122:53–65. [PubMed: 28559211]
- 96.
- Blackett P, Tryggestad J, Krishnan S, Li S, Xu W, Alaupovic P, Quiroga C, Copeland K. Lipoprotein abnormalities in compound heterozygous lipoprotein lipase deficiency after treatment with a low-fat diet and orlistat. J Clin Lipidol. 2013;7:132–139. [PubMed: 23415432]
- 97.
- Allison DB, Gadde KM, Garvey WT, Peterson CA, Schwiers ML, Najarian T, Tam PY, Troupin B, Day WW. Controlled-release phentermine/topiramate in severely obese adults: a randomized controlled trial (EQUIP). Obesity (Silver Spring). 2012;20:330–342. [PMC free article: PMC3270297] [PubMed: 22051941]
- 98.
- Gadde KM, Allison DB, Ryan DH, Peterson CA, Troupin B, Schwiers ML, Day WW. Effects of low-dose, controlled-release, phentermine plus topiramate combination on weight and associated comorbidities in overweight and obese adults (CONQUER): a randomised, placebo-controlled, phase 3 trial. Lancet. 2011;377:1341–1352. [PubMed: 21481449]
- 99.
- Garvey WT, Ryan DH, Look M, Gadde KM, Allison DB, Peterson CA, Schwiers M, Day WW, Bowden CH. Two-year sustained weight loss and metabolic benefits with controlled-release phentermine/topiramate in obese and overweight adults (SEQUEL): a randomized, placebo-controlled, phase 3 extension study. Am J Clin Nutr. 2012;95:297–308. [PMC free article: PMC3260065] [PubMed: 22158731]
- 100.
- Apovian CM, Aronne L, Rubino D, Still C, Wyatt H, Burns C, Kim D, Dunayevich E. Group C-IS. A randomized, phase 3 trial of naltrexone SR/bupropion SR on weight and obesity-related risk factors (COR-II). Obesity (Silver Spring). 2013;21:935–943. [PMC free article: PMC3739931] [PubMed: 23408728]
- 101.
- Greenway FL, Dunayevich E, Tollefson G, Erickson J, Guttadauria M, Fujioka K, Cowley MA, Group NBS. Comparison of combined bupropion and naltrexone therapy for obesity with monotherapy and placebo. J Clin Endocrinol Metab. 2009;94:4898–4906. [PubMed: 19846734]
- 102.
- Greenway FL, Fujioka K, Plodkowski RA, Mudaliar S, Guttadauria M, Erickson J, Kim DD, Dunayevich E. Group C-IS. Effect of naltrexone plus bupropion on weight loss in overweight and obese adults (COR-I): a multicentre, randomised, double-blind, placebo-controlled, phase 3 trial. Lancet. 2010;376:595–605. [PubMed: 20673995]
- 103.
- Wadden TA, Foreyt JP, Foster GD, Hill JO, Klein S, O'Neil PM, Perri MG, Pi-Sunyer FX, Rock CL, Erickson JS, Maier HN, Kim DD, Dunayevich E. Weight loss with naltrexone SR/bupropion SR combination therapy as an adjunct to behavior modification: the COR-BMOD trial. Obesity (Silver Spring). 2011;19:110–120. [PMC free article: PMC4459776] [PubMed: 20559296]
- 104.
- Pi-Sunyer X, Astrup A, Fujioka K, Greenway F, Halpern A, Krempf M, Lau DC, le Roux CW, Violante Ortiz R, Jensen CB, Wilding JP, Obesity S, Prediabetes NNSG. A Randomized, Controlled Trial of 3.0 mg of Liraglutide in Weight Management. N Engl J Med. 2015;373:11–22. [PubMed: 26132939]
- 105.
- Astrup A, Rossner S, Van Gaal L, Rissanen A, Niskanen L, Al Hakim M, Madsen J, Rasmussen MF, Lean ME, Group NNS. Effects of liraglutide in the treatment of obesity: a randomised, double-blind, placebo-controlled study. Lancet. 2009;374:1606–1616. [PubMed: 19853906]
- 106.
- Davies MJ, Bergenstal R, Bode B, Kushner RF, Lewin A, Skjoth TV, Andreasen AH, Jensen CB, DeFronzo RA, Group NNS. Efficacy of Liraglutide for Weight Loss Among Patients With Type 2 Diabetes: The SCALE Diabetes Randomized Clinical Trial. JAMA. 2015;314:687–699. [PubMed: 26284720]
- 107.
- Wilding JPH, Batterham RL, Calanna S, Davies M, Van Gaal LF, Lingvay I, McGowan BM, Rosenstock J, Tran MTD, Wadden TA, Wharton S, Yokote K, Zeuthen N, Kushner RF, Group SS. Once-Weekly Semaglutide in Adults with Overweight or Obesity. N Engl J Med. 2021;384:989–1002. [PubMed: 33567185]
- 108.
- Davies M, Faerch L, Jeppesen OK, Pakseresht A, Pedersen SD, Perreault L, Rosenstock J, Shimomura I, Viljoen A, Wadden TA, Lingvay I, Group SS. Semaglutide 2.4 mg once a week in adults with overweight or obesity, and type 2 diabetes (STEP 2): a randomised, double-blind, double-dummy, placebo-controlled, phase 3 trial. Lancet. 2021;397:971–984. [PubMed: 33667417]
- 109.
- Wadden TA, Bailey TS, Billings LK, Davies M, Frias JP, Koroleva A, Lingvay I, O'Neil PM, Rubino DM, Skovgaard D, Wallenstein SOR, Garvey WT, Investigators S. Effect of Subcutaneous Semaglutide vs Placebo as an Adjunct to Intensive Behavioral Therapy on Body Weight in Adults With Overweight or Obesity: The STEP 3 Randomized Clinical Trial. JAMA. 2021;325:1403–1413. [PMC free article: PMC7905697] [PubMed: 33625476]
- 110.
- Nauck MA, Meier JJ, Cavender MA, Abd El Aziz M, Drucker DJ. Cardiovascular Actions and Clinical Outcomes With Glucagon-Like Peptide-1 Receptor Agonists and Dipeptidyl Peptidase-4 Inhibitors. Circulation. 2017;136:849–870. [PubMed: 28847797]
- 111.
- Kim TY, Kim S, Schafer AL. Medical Management of the Postoperative Bariatric Surgery Patient. In: Feingold KR, Anawalt B, Boyce A, Chrousos G, Dungan K, Grossman A, Hershman JM, Kaltsas G, Koch C, Kopp P, Korbonits M, McLachlan R, Morley JE, New M, Perreault L, Purnell J, Rebar R, Singer F, Trence DL, Vinik A, Wilson DP, eds. Endotext. South Dartmouth (MA)2017.
- 112.
- O'Brien P. Surgical Treatment of Obesity. In: Feingold KR, Anawalt B, Boyce A, Chrousos G, Dungan K, Grossman A, Hershman JM, Kaltsas G, Koch C, Kopp P, Korbonits M, McLachlan R, Morley JE, New M, Perreault L, Purnell J, Rebar R, Singer F, Trence DL, Vinik A, Wilson DP, eds. Endotext. South Dartmouth (MA)2016.
- 113.
- Gloy VL, Briel M, Bhatt DL, Kashyap SR, Schauer PR, Mingrone G, Bucher HC, Nordmann AJ. Bariatric surgery versus non-surgical treatment for obesity: a systematic review and meta-analysis of randomised controlled trials. BMJ. 2013;347:f5934. [PMC free article: PMC3806364] [PubMed: 24149519]
- 114.
- Dash S, Xiao C, Lewis GF. Effects of bariatric surgery on hepatic and intestinal lipoprotein particle metabolism. Curr Opin Lipidol. 2016;27:14–18. [PubMed: 26628436]
- 115.
- Heffron SP, Parikh A, Volodarskiy A, Ren-Fielding C, Schwartzbard A, Nicholson J, Bangalore S. Changes in Lipid Profile of Obese Patients Following Contemporary Bariatric Surgery: A Meta-Analysis. Am J Med. 2016;129:952–959. [PMC free article: PMC4988934] [PubMed: 26899751]
- 116.
- Nguyen NT, Varela E, Sabio A, Tran CL, Stamos M, Wilson SE. Resolution of hyperlipidemia after laparoscopic Roux-en-Y gastric bypass. J Am Coll Surg. 2006;203:24–29. [PubMed: 16798484]
- 117.
- Griffo E, Cotugno M, Nosso G, Saldalamacchia G, Mangione A, Angrisani L, Rivellese AA, Capaldo B. Effects of Sleeve Gastrectomy and Gastric Bypass on Postprandial Lipid Profile in Obese Type 2 Diabetic Patients: a 2-Year Follow-up. Obes Surg. 2016;26:1247–1253. [PubMed: 26435537]
- 118.
- Lin BX, Weiss MC, Parikh M, Berger JS, Fisher EA, Heffron SP. Changes in lipoprotein(a) following bariatric surgery. Am Heart J. 2018;197:175–176. [PMC free article: PMC6583893] [PubMed: 29447779]
- 119.
- Boman L, Ericson M. Lipoprotein A levels after intestinal bypass operation for morbid obesity. Obes Surg. 1997;7:125–127. [PubMed: 9730539]
- 120.
- Aron-Wisnewsky J, Julia Z, Poitou C, Bouillot JL, Basdevant A, Chapman MJ, Clement K, Guerin M. Effect of bariatric surgery-induced weight loss on SR-BI-, ABCG1-, and ABCA1-mediated cellular cholesterol efflux in obese women. J Clin Endocrinol Metab. 2011;96:1151–1159. [PubMed: 21289254]
- 121.
- Heffron SP, Lin BX, Parikh M, Scolaro B, Adelman SJ, Collins HL, Berger JS, Fisher EA. Changes in High-Density Lipoprotein Cholesterol Efflux Capacity After Bariatric Surgery Are Procedure Dependent. Arterioscler Thromb Vasc Biol. 2018;38:245–254. [PMC free article: PMC5746465] [PubMed: 29162605]
- 122.
- Osto E, Doytcheva P, Corteville C, Bueter M, Dorig C, Stivala S, Buhmann H, Colin S, Rohrer L, Hasballa R, Tailleux A, Wolfrum C, Tona F, Manz J, Vetter D, Spliethoff K, Vanhoutte PM, Landmesser U, Pattou F, Staels B, Matter CM, Lutz TA, Luscher TF. Rapid and body weight-independent improvement of endothelial and high-density lipoprotein function after Roux-en-Y gastric bypass: role of glucagon-like peptide-1. Circulation. 2015;131:871–881. [PubMed: 25673670]
- 123.
- Peterli R, Wolnerhanssen BK, Peters T, Vetter D, Kroll D, Borbely Y, Schultes B, Beglinger C, Drewe J, Schiesser M, Nett P, Bueter M. Effect of Laparoscopic Sleeve Gastrectomy vs Laparoscopic Roux-en-Y Gastric Bypass on Weight Loss in Patients With Morbid Obesity: The SM-BOSS Randomized Clinical Trial. JAMA. 2018;319:255–265. [PMC free article: PMC5833546] [PubMed: 29340679]
- 124.
- Salminen P, Helmio M, Ovaska J, Juuti A, Leivonen M, Peromaa-Haavisto P, Hurme S, Soinio M, Nuutila P, Victorzon M. Effect of Laparoscopic Sleeve Gastrectomy vs Laparoscopic Roux-en-Y Gastric Bypass on Weight Loss at 5 Years Among Patients With Morbid Obesity: The SLEEVEPASS Randomized Clinical Trial. JAMA. 2018;319:241–254. [PMC free article: PMC5833550] [PubMed: 29340676]
- 125.
- Schauer PR, Bhatt DL, Kirwan JP, Wolski K, Aminian A, Brethauer SA, Navaneethan SD, Singh RP, Pothier CE, Nissen SE, Kashyap SR, Investigators S. Bariatric Surgery versus Intensive Medical Therapy for Diabetes - 5-Year Outcomes. N Engl J Med. 2017;376:641–651. [PMC free article: PMC5451258] [PubMed: 28199805]
- 126.
- Li JF, Lai DD, Ni B, Sun KX. Comparison of laparoscopic Roux-en-Y gastric bypass with laparoscopic sleeve gastrectomy for morbid obesity or type 2 diabetes mellitus: a meta-analysis of randomized controlled trials. Can J Surg. 2013;56:E158–164. [PMC free article: PMC3859791] [PubMed: 24284156]
- 127.
- Puzziferri N, Roshek TB 3rd, Mayo HG, Gallagher R, Belle SH, Livingston EH. Long-term follow-up after bariatric surgery: a systematic review. JAMA. 2014;312:934–942. [PMC free article: PMC4409000] [PubMed: 25182102]
- 128.
- Schauer PR, Nissen SE. After 70 Years, Metabolic Surgery Has Earned a Cardiovascular Outcome Trial. Circulation. 2021;143:1481–1483. [PubMed: 33844579]
- 129.
- Feingold KR. Cholesterol Lowering Drugs. In: Feingold KR, Anawalt B, Boyce A, Chrousos G, Dungan K, Grossman A, Hershman JM, Kaltsas G, Koch C, Kopp P, Korbonits M, McLachlan R, Morley JE, New M, Perreault L, Purnell J, Rebar R, Singer F, Trence DL, Vinik A, Wilson DP, eds. Endotext. South Dartmouth (MA)2021.
- 130.
- Feingold KR. Triglyceride Lowering Drugs. In: Feingold KR, Anawalt B, Boyce A, Chrousos G, Dungan K, Grossman A, Hershman JM, Kaltsas G, Koch C, Kopp P, Korbonits M, McLachlan R, Morley JE, New M, Perreault L, Purnell J, Rebar R, Singer F, Trence DL, Vinik A, Wilson DP, eds. Endotext. South Dartmouth (MA)2021.
- 131.
- Preiss D, Sattar N. Statins and the risk of new-onset diabetes: a review of recent evidence. Curr Opin Lipidol. 2011;22:460–466. [PubMed: 21897230]
- 132.
- Sattar N, Preiss D, Murray HM, Welsh P, Buckley BM, de Craen AJ, Seshasai SR, McMurray JJ, Freeman DJ, Jukema JW, Macfarlane PW, Packard CJ, Stott DJ, Westendorp RG, Shepherd J, Davis BR, Pressel SL, Marchioli R, Marfisi RM, Maggioni AP, Tavazzi L, Tognoni G, Kjekshus J, Pedersen TR, Cook TJ, Gotto AM, Clearfield MB, Downs JR, Nakamura H, Ohashi Y, Mizuno K, Ray KK, Ford I. Statins and risk of incident diabetes: a collaborative meta-analysis of randomised statin trials. Lancet. 2010;375:735–742. [PubMed: 20167359]
- 133.
- Swerdlow DI, Preiss D, Kuchenbaecker KB, Holmes MV, Engmann JE, Shah T, Sofat R, Stender S, Johnson PC, Scott RA, Leusink M, Verweij N, Sharp SJ, Guo Y, Giambartolomei C, Chung C, Peasey A, Amuzu A, Li K, Palmen J, Howard P, Cooper JA, Drenos F, Li YR, Lowe G, Gallacher J, Stewart MC, Tzoulaki I, Buxbaum SG. van der AD, Forouhi NG, Onland-Moret NC, van der Schouw YT, Schnabel RB, Hubacek JA, Kubinova R, Baceviciene M, Tamosiunas A, Pajak A, Topor-Madry R, Stepaniak U, Malyutina S, Baldassarre D, Sennblad B, Tremoli E, de Faire U, Veglia F, Ford I, Jukema JW, Westendorp RG, de Borst GJ, de Jong PA, Algra A, Spiering W, der Zee AH, Klungel OH, de Boer A, Doevendans PA, Eaton CB, Robinson JG, Duggan D, Diagram Consortium MCIC, Kjekshus J, Downs JR, Gotto AM, Keech AC, Marchioli R, Tognoni G, Sever PS, Poulter NR, Waters DD, Pedersen TR, Amarenco P, Nakamura H, McMurray JJ, Lewsey JD, Chasman DI, Ridker PM, Maggioni AP, Tavazzi L, Ray KK, Seshasai SR, Manson JE, Price JF, Whincup PH, Morris RW, Lawlor DA, Smith GD, Ben-Shlomo Y, Schreiner PJ, Fornage M, Siscovick DS, Cushman M, Kumari M, Wareham NJ, Verschuren WM, Redline S, Patel SR, Whittaker JC, Hamsten A, Delaney JA, Dale C, Gaunt TR, Wong A, Kuh D, Hardy R, Kathiresan S, Castillo BA, van der Harst P, Brunner EJ, Tybjaerg-Hansen A, Marmot MG, Krauss RM, Tsai M, Coresh J, Hoogeveen RC, Psaty BM, Lange LA, Hakonarson H, Dudbridge F, Humphries SE, Talmud PJ, Kivimaki M, Timpson NJ, Langenberg C, Asselbergs FW, Voevoda M, Bobak M, Pikhart H, Wilson JG, Reiner AP, Keating BJ, Hingorani AD, Sattar N. HMG-coenzyme A reductase inhibition, type 2 diabetes, and bodyweight: evidence from genetic analysis and randomised trials. Lancet. 2014
- 134.
- Mikus CR, Boyle LJ, Borengasser SJ, Oberlin DJ, Naples SP, Fletcher J, Meers GM, Ruebel M, Laughlin MH, Dellsperger KC, Fadel PJ, Thyfault JP. Simvastatin impairs exercise training adaptations. J Am Coll Cardiol. 2013;62:709–714. [PMC free article: PMC3745788] [PubMed: 23583255]
- 135.
- Noyes AM, Thompson PD. The effects of statins on exercise and physical activity. J Clin Lipidol. 2017;11:1134–1144. [PubMed: 28807461]
- 136.
- Baigent C, Keech A, Kearney PM, Blackwell L, Buck G, Pollicino C, Kirby A, Sourjina T, Peto R, Collins R, Simes R. Cholesterol Treatment Trialists C. Efficacy and safety of cholesterol-lowering treatment: prospective meta-analysis of data from 90,056 participants in 14 randomised trials of statins. Lancet. 2005;366:1267–1278. [PubMed: 16214597]
- 137.
- Cholesterol Treatment Trialists C. Baigent C, Blackwell L, Emberson J, Holland LE, Reith C, Bhala N, Peto R, Barnes EH, Keech A, Simes J, Collins R. Efficacy and safety of more intensive lowering of LDL cholesterol: a meta-analysis of data from 170,000 participants in 26 randomised trials. Lancet. 2010;376:1670–1681. [PMC free article: PMC2988224] [PubMed: 21067804]
- 138.
- Ooi CP, Loke SC. Colesevelam for type 2 diabetes mellitus. Cochrane Database Syst Rev. 2012;12:CD009361. [PMC free article: PMC6486226] [PubMed: 23235674]
- 139.
- Song WL, FitzGerald GA. Niacin, an old drug with a new twist. J Lipid Res. 2013;54:2586–2594. [PMC free article: PMC3770072] [PubMed: 23948546]
- 140.
- Group HTC, Landray MJ, Haynes R, Hopewell JC, Parish S, Aung T, Tomson J, Wallendszus K, Craig M, Jiang L, Collins R, Armitage J. Effects of extended-release niacin with laropiprant in high-risk patients. N Engl J Med. 2014;371:203–212. [PubMed: 25014686]
- 141.
- Cannon CP, Cariou B, Blom D, McKenney JM, Lorenzato C, Pordy R, Chaudhari U, Colhoun HM, Investigators OCI. Efficacy and safety of alirocumab in high cardiovascular risk patients with inadequately controlled hypercholesterolaemia on maximally tolerated doses of statins: the ODYSSEY COMBO II randomized controlled trial. Eur Heart J. 2015;36:1186–1194. [PMC free article: PMC4430683] [PubMed: 25687353]
- 142.
- Tinahones FJ, Laufs U, Cariou B, Louie MJ, Yang J, Thompson D, Leiter LA. Alirocumab efficacy and safety by body mass index: A pooled analysis from 10 Phase 3 ODYSSEY trials. Diabetes Metab. 2019:101120. [PubMed: 31533069]
- 143.
- Ray KK, Wright RS, Kallend D, Koenig W, Leiter LA, Raal FJ, Bisch JA, Richardson T, Jaros M, Wijngaard PLJ, Kastelein JJP. Orion, Investigators O-. Two Phase 3 Trials of Inclisiran in Patients with Elevated LDL Cholesterol. N Engl J Med. 2020;382:1507–1519. [PubMed: 32187462]
- 144.
- Wilson DP. Is Atherosclerosis a Pediatric Disease? In: Feingold KR, Anawalt B, Boyce A, Chrousos G, Dungan K, Grossman A, Hershman JM, Kaltsas G, Koch C, Kopp P, Korbonits M, McLachlan R, Morley JE, New M, Perreault L, Purnell J, Rebar R, Singer F, Trence DL, Vinik A, Wilson DP, eds. Endotext. South Dartmouth (MA)2020.
- 145.
- Ference BA, Ray KK, Catapano AL, Ference TB, Burgess S, Neff DR, Oliver-Williams C, Wood AM, Butterworth AS, Di Angelantonio E, Danesh J, Kastelein JJP, Nicholls SJ. Mendelian Randomization Study of ACLY and Cardiovascular Disease. N Engl J Med. 2019;380:1033–1042. [PMC free article: PMC7612927] [PubMed: 30865797]
- 146.
- Feingold KR. Maximizing the benefits of cholesterol-lowering drugs. Curr Opin Lipidol. 2019;30:388–394. [PubMed: 31335331]
- 147.
- Cannon CP, Blazing MA, Giugliano RP, McCagg A, White JA, Theroux P, Darius H, Lewis BS, Ophuis TO, Jukema JW, De Ferrari GM, Ruzyllo W, De Lucca P, Im K, Bohula EA, Reist C, Wiviott SD, Tershakovec AM, Musliner TA, Braunwald E, Califf RM. Investigators I-I. Ezetimibe Added to Statin Therapy after Acute Coronary Syndromes. N Engl J Med. 2015;372:2387–2397. [PubMed: 26039521]
- 148.
- Sabatine MS, Giugliano RP, Keech AC, Honarpour N, Wiviott SD, Murphy SA, Kuder JF, Wang H, Liu T, Wasserman SM, Sever PS, Pedersen TR, Committee FS. Investigators. Evolocumab and Clinical Outcomes in Patients with Cardiovascular Disease. N Engl J Med. 2017;376:1713–1722. [PubMed: 28304224]
- 149.
- Ridker PM, Revkin J, Amarenco P, Brunell R, Curto M, Civeira F, Flather M, Glynn RJ, Gregoire J, Jukema JW, Karpov Y, Kastelein JJP, Koenig W, Lorenzatti A, Manga P, Masiukiewicz U, Miller M, Mosterd A, Murin J, Nicolau JC, Nissen S, Ponikowski P, Santos RD, Schwartz PF, Soran H, White H, Wright RS, Vrablik M, Yunis C, Shear CL, Tardif JC, Investigators SCO. Cardiovascular Efficacy and Safety of Bococizumab in High-Risk Patients. N Engl J Med. 2017;376:1527–1539. [PubMed: 28304242]
- 150.
- Schwartz GG, Steg PG, Szarek M, Bhatt DL, Bittner VA, Diaz R, Edelberg JM, Goodman SG, Hanotin C, Harrington RA, Jukema JW, Lecorps G, Mahaffey KW, Moryusef A, Pordy R, Quintero K, Roe MT, Sasiela WJ, Tamby JF, Tricoci P, White HD, Zeiher AM, Committees OO. Investigators. Alirocumab and Cardiovascular Outcomes after Acute Coronary Syndrome. N Engl J Med. 2018;379:2097–2107. [PubMed: 30403574]
- 151.
- Nissen SE, Lincoff AM, Brennan D, Ray KK, Mason D, Kastelein JJP, Thompson PD, Libby P, Cho L, Plutzky J, Bays HE, Moriarty PM, Menon V, Grobbee DE, Louie MJ, Chen CF, Li N, Bloedon L, Robinson P, Horner M, Sasiela WJ, McCluskey J, Davey D, Fajardo-Campos P, Petrovic P, Fedacko J, Zmuda W, Lukyanov Y, Nicholls SJ, Investigators CO. Bempedoic Acid and Cardiovascular Outcomes in Statin-Intolerant Patients. N Engl J Med. 2023;388:1353–1364. [PubMed: 36876740]
- 152.
- Jellinger PS, Handelsman Y, Rosenblit PD, Bloomgarden ZT, Fonseca VA, Garber AJ, Grunberger G, Guerin CK, Bell DSH, Mechanick JI, Pessah-Pollack R, Wyne K, Smith D, Brinton EA, Fazio S, Davidson M, Zangeneh F, Bush MA. AMERICAN ASSOCIATION OF CLINICAL ENDOCRINOLOGISTS AND AMERICAN COLLEGE OF ENDOCRINOLOGY GUIDELINES FOR MANAGEMENT OF DYSLIPIDEMIA AND PREVENTION OF CARDIOVASCULAR DISEASE - EXECUTIVE SUMMARYComplete Appendix to Guidelines available at http://journals
.aace.com. Endocr Pract 2017; 23:479-497. [PubMed: 28156151] - 153.
- Sandesara PB, Virani SS, Fazio S, Shapiro MD. The Forgotten Lipids: Triglycerides, Remnant Cholesterol, and Atherosclerotic Cardiovascular Disease Risk. Endocr Rev. 2019;40:537–557. [PMC free article: PMC6416708] [PubMed: 30312399]
- 154.
- Investigators A-H. Boden WE, Probstfield JL, Anderson T, Chaitman BR, Desvignes-Nickens P, Koprowicz K, McBride R, Teo K, Weintraub W. Niacin in patients with low HDL cholesterol levels receiving intensive statin therapy. N Engl J Med. 2011;365:2255–2267. [PubMed: 22085343]
- 155.
- Group AS, Ginsberg HN, Elam MB, Lovato LC, Crouse JR 3rd, Leiter LA, Linz P, Friedewald WT, Buse JB, Gerstein HC, Probstfield J, Grimm RH, Ismail-Beigi F, Bigger JT, Goff DC Jr, Cushman WC, Simons-Morton DG, Byington RP. Effects of combination lipid therapy in type 2 diabetes mellitus. N Engl J Med. 2010;362:1563–1574. [PMC free article: PMC2879499] [PubMed: 20228404]
- 156.
- Das Pradhan A, Glynn RJ, Fruchart JC, MacFadyen JG, Zaharris ES, Everett BM, Campbell SE, Oshima R, Amarenco P, Blom DJ, Brinton EA, Eckel RH, Elam MB, Felicio JS, Ginsberg HN, Goudev A, Ishibashi S, Joseph J, Kodama T, Koenig W, Leiter LA, Lorenzatti AJ, Mankovsky B, Marx N, Nordestgaard BG, Pall D, Ray KK, Santos RD, Soran H, Susekov A, Tendera M, Yokote K, Paynter NP, Buring JE, Libby P, Ridker PM, Investigators P. Triglyceride Lowering with Pemafibrate to Reduce Cardiovascular Risk. N Engl J Med. 2022;387:1923–1934. [PubMed: 36342113]
- 157.
- Bhatt DL, Steg PG, Miller M, Brinton EA, Jacobson TA, Ketchum SB, Doyle RT Jr, Juliano RA, Jiao L, Granowitz C, Tardif JC, Ballantyne CM. Investigators R-I. Cardiovascular Risk Reduction with Icosapent Ethyl for Hypertriglyceridemia. N Engl J Med. 2019;380:11–22. [PubMed: 30415628]
- 158.
- Mason RP, Libby P, Bhatt DL. Emerging Mechanisms of Cardiovascular Protection for the Omega-3 Fatty Acid Eicosapentaenoic Acid. Arterioscler Thromb Vasc Biol 2020:ATVBAHA119313286. [PMC free article: PMC7176343] [PubMed: 32212849]
- 159.
- Goff ZD, Nissen SE. N-3 polyunsaturated fatty acids for cardiovascular risk. Curr Opin Cardiol. 2022;37:356–363. [PubMed: 35275889]
- 160.
- Mason RP, Sherratt SCR, Eckel RH. Omega-3-fatty acids: Do they prevent cardiovascular disease? Best Pract Res Clin Endocrinol Metab. 2022:101681. [PubMed: 35739003]
- 161.
- Chait A, Subramanian S. Hypertriglyceridemia: Pathophysiology, Role of Genetics, Consequences, and Treatment. In: Feingold KR, Anawalt B, Boyce A, Chrousos G, Dungan K, Grossman A, Hershman JM, Kaltsas G, Koch C, Kopp P, Korbonits M, McLachlan R, Morley JE, New M, Perreault L, Purnell J, Rebar R, Singer F, Trence DL, Vinik A, Wilson DP, eds. Endotext. South Dartmouth (MA)2019.
- 162.
- Chait A, Eckel RH. The Chylomicronemia Syndrome Is Most Often Multifactorial: A Narrative Review of Causes and Treatment. Ann Intern Med. 2019;170:626–634. [PubMed: 31035285]
- 163.
- Niisuke K, Horvath KV, Asztalos BF. Where next with HDL assays? Curr Opin Lipidol. 2018;29:293–298. [PubMed: 29846266]
- 164.
- Hovingh GK, Rader DJ, Hegele RA. HDL re-examined. Curr Opin Lipidol. 2015;26:127–132. [PubMed: 25692348]
- 165.
- Navab M, Reddy ST, Van Lenten BJ, Anantharamaiah GM, Fogelman AM. The role of dysfunctional HDL in atherosclerosis. J Lipid Res. 2009;50 Suppl:S145–149. [PMC free article: PMC2674720] [PubMed: 18955731]
- Review Triglyceride Lowering Drugs.[Endotext. 2000]Review Triglyceride Lowering Drugs.Feingold KR. Endotext. 2000
- Review Targeting low HDL-cholesterol to decrease residual cardiovascular risk in the managed care setting.[J Manag Care Pharm. 2008]Review Targeting low HDL-cholesterol to decrease residual cardiovascular risk in the managed care setting.Cziraky MJ, Watson KE, Talbert RL. J Manag Care Pharm. 2008 Oct; 14(8 Suppl):S3-28; quiz S30-1.
- Review Dyslipidemia in Patients with Diabetes.[Endotext. 2000]Review Dyslipidemia in Patients with Diabetes.Feingold KR. Endotext. 2000
- Effects of switching from omega-3-acid ethyl esters to icosapent ethyl in a statin-treated patient with elevated triglycerides.[Postgrad Med. 2015]Effects of switching from omega-3-acid ethyl esters to icosapent ethyl in a statin-treated patient with elevated triglycerides.Kedia AW, Lynch E. Postgrad Med. 2015; 127(8):869-73. Epub 2015 Oct 9.
- Review Role of Glucose and Lipids in the Atherosclerotic Cardiovascular Disease in Patients with Diabetes.[Endotext. 2000]Review Role of Glucose and Lipids in the Atherosclerotic Cardiovascular Disease in Patients with Diabetes.Feingold KR. Endotext. 2000
- Obesity and Dyslipidemia - EndotextObesity and Dyslipidemia - Endotext
Your browsing activity is empty.
Activity recording is turned off.
See more...