By agreement with the publisher, this book is accessible by the search feature, but cannot be browsed.
NCBI Bookshelf. A service of the National Library of Medicine, National Institutes of Health.
Siegel GJ, Agranoff BW, Albers RW, et al., editors. Basic Neurochemistry: Molecular, Cellular and Medical Aspects. 6th edition. Philadelphia: Lippincott-Raven; 1999.
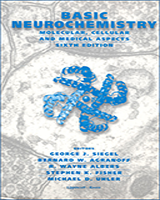
Basic Neurochemistry: Molecular, Cellular and Medical Aspects. 6th edition.
Show detailsThe study of peptidergic neurons requires a number of special tools
These tools include methods to detect the neuropeptides, the enzymes specific to their biosynthesis and their cognate receptors.
Antibody-based detection includes immunocytochemistry, which gives only qualitative data but has very good spatial resolution. Radioimmunoassay is a second method for neuropeptide detection which provides a quantitative measure of release or content. Sometimes passive immunization has been used successfully to establish the importance of a particular peptide in a physiological interaction between cells. One of the major limitations of all antibody-based methods is the potential for cross-reactivity among the many peptides that are distinct but closely related structurally. For example, some of the most sensitive “gastrin” antisera also detect CCK since the peptides share a common COOH-terminal tetrapeptide sequence.
RNA-based methods for detection of mRNA-encoding neuropeptides include Northern blots and RNase protection assays, which provide quantitative data but lack fine anatomical resolution. Alternatively, in situ hybridization preserves anatomical relationships but is not usually considered quantitative.
Direct methods for detection of neuropeptides include metabolic labeling with an appropriate radioactive amino acid and chemical isolation of peptides. Isolation often involves using high-pressure liquid chromatography and sensitive spectroscopic methods of detection along with mass spectroscopy. These approaches make possible measurements of tissue concentrations of peptides and quantitative study of the amount and timing of secretion. Direct methods are difficult to apply to peptides or their receptors in the CNS, where the cells expressing the peptide are part of a large population of other neurons or endocrine cells.
Peptide agonists and antagonists are becoming available for many peptides, and (as discussed in Chaps. 11–16) these are essential to a successful dissection of the function of peptidergic synapses, as they have been for conventional neurotransmitters.
Ligand-binding assays allow localization and quantification of receptors, using an appropriate tracer ligand. Measurement of receptors may be made in solution, in suspension or by binding to membranes in situ. These approaches have the advantage that a physiologically important trait, binding of the ligand to the receptor, is being observed, and they can reveal binding to several related receptors, which are then distinguished by antisera or in situ hybridization.
Peptides play a role in the plurichemical coding of neuronal signals
As shown in Figure 18-10, many sets of neurons are chemically coded, giving rise to the concept of plurichemical transmission [21]. In the human lumbar paravertebral ganglion, adrenergic neurons efferent to blood vessels in skin and muscle usually contain NPY along with norepinephrine, while adrenergic neurons innervating hair follicles express primarily norepinephrine. The dual transmission onto blood vessels is important since the catecholamine effects on blood vessels undergo rapid tachyphylaxis, while sustained contraction of the blood vessels is mediated by NPY. In this case, the conventional neurotransmitter norepinephrine and NPY have similar actions on different time scales, leading to rapid but sustained responses of the same sort. In the sympathetic innervation of sweat glands, another example of plasticity is seen. The sympathetic fibers first express catecholamines as they arrive at the sweat gland, and in a later response to a factor released by the sweat gland, the fibers change to express a cholinergic phenotype. In this later response, a set of peptides is expressed that is different from that expressed when the fibers initially reach the sweat glands. In this way, within a sympathetic ganglion, several functional subgroups of neurons can be identified based upon their content of neuropeptide and conventional neurotransmitter. It is likely that a similar but more complex mixture of phenotypes occurs in the CNS. Peptides are generally released together with classical neurotransmitters, and each transmitter has its own unique effects on target tissues. Neuropeptides have a particularly important role in the integration of incoming and outgoing signals of sympathetic ganglia.
![Figure 18-10. Plurichemical transmission in a sympathetic ganglion (adapted from [21]).](/books/NBK28128/bin/ch18f10.gif)
Figure 18-10
Plurichemical transmission in a sympathetic ganglion (adapted from [21]). Cholinergic stimulation of distinct ganglionic neurons leads to distinct neurotransmitter release and physiological actions. ACh, acetylcholine; Enk, enkephalin; NE, norepinephrine; (more...)
Neuropeptides make a unique contribution to signaling
The release of neuropeptides generally requires a more intense stimulus, resulting in more entry of Ca2+ into the presynaptic terminal, than required for release of conventional neurotransmitters, presumably due to the distance the Ca2+ must diffuse to reach the LDCVs (Fig. 18-3). As a result, the contribution of peptides to signaling can vary with the pattern of stimulation. For example, in Aplysia, several identified motor neurons devote about 10% of their total protein synthesis to their respective peptide neurotransmitters. Peptide release in each case has been shown to require extracellular Ca2+, and release increases with the frequency of action potentials, in the range of frequencies seen in behaving animals. In addition to the peptides, several of the motor neurons secrete ACh. In these motor neurons, the peptide release increases more strongly with action potential frequency than does release of ACh, so the ratio of peptide to ACh is dependent on frequency. In addition, there are unique postsynaptic responses, such as elevation of cAMP concentrations in muscles, which can be mimicked only by application of the peptides and not by the conventional neurotransmitters.
In other examples, the amount of peptide available for release can be depleted by repeated firing of a terminal since new peptide must arrive by axonal transport, while new conventional neurotransmitters are synthesized or recaptured locally and pumped into small synaptic vesicles.
Regulation of neuropeptide expression is exerted at several levels
Control of neuropeptide function is mediated by factors controlling rates of prepropeptide gene transcription, translation, degradation and secretion (Fig. 18-11). On the scale of seconds to minutes, peptide secretion is not always coupled lock-step with classical transmitter release (example above). Peptides are inactivated by diffusion and by proteolysis, so it would be expected that inhibition of specific extracellular proteases could lead to increased effectiveness of their neuropeptide substrates, as has been shown in the cases of CCK and the enkephalins.

Figure 18-11
Regulation of neuropeptide expression is exerted at several levels. ER, endoplasmic reticulum; LDCV, large dense core vesicle; TGN, trans-Golgi network.
On a time scale of minutes to hours, the transcription of prepropeptide mRNA can be controlled, and peptide mRNAs are immediate early genes in many cases, showing extremely rapid responses to stimuli. The rate of translation of existing prepropeptide mRNAs can also change dramatically within a few minutes of stimulation. Since processing enzymes must also be synthesized and inserted into the same LDCVs as the peptide precursors, the rate of production of these enzymes might be expected to show changes under physiological conditions. They do show such changes but usually more slowly than the peptide precursors. As seen above (Figs. 18-4–18-7), the peptide products produced are crucially dependent on the mixture of processing enzymes in the LDCVs.
On a scale of hours to days, dramatic changes can be seen in cells producing peptides that are subjected to chronic stimulation or inhibition. POMC production by intermediate pituitary melanotropes is inhibited by treatment of rats with dopaminergic agonists or exposure of frogs to a light background; POMC production is stimulated by treatment of rats with dopaminergic antagonists or exposure of frogs to a dark background. The expression of processing enzymes, the number of LDCVs and the rate of cell division are also responsive to the treatments. Similarly, the processing of different regions of the pro-TRH precursor responds to thyroid hormone status, and it is possible that the routing of ELH and the BCPs is regulated (Fig. 18-7). In response to changes in steroid hormone concentrations that occur during the estrous cycle in female rats, the concentration of CCK mRNA varies more than twofold in a subset of CCK-producing CNS neurons.
- Neuropeptide Functions and Regulation - Basic NeurochemistryNeuropeptide Functions and Regulation - Basic Neurochemistry
- Borderline Breast Phyllodes TumorBorderline Breast Phyllodes TumorMedGen
- Plasmodium falciparum 3D7 genome assembly, chromosome: 11Plasmodium falciparum 3D7 genome assembly, chromosome: 11gi|1371547585|ref|NC_037282.1||gnl| CF_000002765.5|11Nucleotide
- BioAssay, by Gene target for Gene (Select 54496) (15)PubChem BioAssay
- TRPC4 transient receptor potential cation channel subfamily C member 4 [Homo sap...TRPC4 transient receptor potential cation channel subfamily C member 4 [Homo sapiens]Gene ID:7223Gene
Your browsing activity is empty.
Activity recording is turned off.
See more...