NCBI Bookshelf. A service of the National Library of Medicine, National Institutes of Health.
Feingold KR, Anawalt B, Blackman MR, et al., editors. Endotext [Internet]. South Dartmouth (MA): MDText.com, Inc.; 2000-.
ABSTRACT
Since the symptoms of hypogonadism are nonspecific, and the signs of testosterone deficiency can be subtle and slow to develop, the assessment of testicular function relies heavily on laboratory testing. The laboratory diagnosis of hypogonadism is based on a consistent and unequivocally low serum total testosterone level measured in blood samples obtained in the early morning, but normal ranges vary with different methods and among laboratories. Moreover, many men who present with adult onset testosterone deficiency have a low level of sex hormone-binding globulin (SHBG) associated with obesity, insulin resistance, and type 2 diabetes. In these men, tests for the free (or non-SHBG/bioavailable) testosterone fraction testosterone) are helpful for an accurate diagnosis. If testosterone deficiency is confirmed, the next step is to differentiate between primary and secondary hypogonadism by measuring LH and FSH. With many disorders, however, both the testes and the hypothalamic-pituitary unit are affected. Other tests such as estradiol, inhibin-B, and Mullerian inhibitory hormone, and provocative endocrine tests using hCG, GnRH or its analogs, or antiandrogens or antiestrogens, and semen analysis in the subfertile male are discussed. For complete coverage of all related areas of Endocrinology, please visit our on-line FREE web-text, WWW.ENDOTEXT.ORG.
INTRODUCTION
The evaluation of men for suspected hypogonadism begins with a detailed medical history and a careful physical examination. Laboratory tests are an essential component of almost all evaluations, and proper interpretation of the results obtained requires an understanding of methodology as well as an awareness of the impact of endocrine rhythms, age, race, body composition, drug exposure, co-morbidities, other illnesses, and nutrition on tests of endocrine testicular function. Endocrinologists rely on clinical laboratories to provide accurate and precise, and in some cases highly sensitive assays for accurate diagnoses. We expect the reference ranges to be based on large normal control populations, and for some assays, normative data must be stratified by age. Unfortunately, these expectations are not always fulfilled. Endocrinologists should examine the protocols for each assay, and discuss them with the laboratory director. In recognition of these potential shortcomings, the U.S. Endocrine Society has spearheaded efforts towards standardizing the methods by which testosterone assays are validated (1), and several societies have provided guidelines and recommendations to physicians who order and interpret the results of androgen assays (2-4). The College of American Pathologists and the U.S. Centers for Disease Control have voluntary Hormone Standardization Programs to help clinical, research, and public health laboratories maintain and enhance the quality and comparability of their results.
TESTOSTERONE
Testosterone, the major androgen in men, is necessary for fetal male sexual differentiation, pubertal development, and the maintenance of adult secondary sex characteristics and spermatogenesis. Testosterone regulates gene expression in most extra-genital tissues, including muscle and bone, and modulates the immune system. The testes are the source of more than 95% of the circulating testosterone in men although the adrenal cortex produces large amounts of the testosterone precursor steroids, dehydroepiandrosterone (DHEA) and androstenedione.
Following birth, there is a period of activation of the gonadotropin-releasing hormone (GnRH) pulse generator that stimulates testosterone secretion to peak levels of 200-300 ng/dl (7-10.5 nmol/L) at ages 1-2 months and lasts until age 4-6 months, and is often called mini-puberty (5). Thereafter, GnRH secretion declines, causing a fall in testosterone to very low levels until puberty begins. LH and testosterone testing at mini-puberty provides an opportunity to confirm the diagnosis of hypogonadotropic hypogonadism (6).
In early puberty, LH and testosterone secretion increase dramatically during sleep which gradually transitions to sustained secretion throughout the day and night (7). The nocturnal rise in testosterone levels in early puberty can be used clinically to evaluate boys with delayed puberty because it may precede pubertal testis growth, and indicates that puberty has begun. Figure 1 illustrates age-specific median values and ranges for testosterone in morning blood samples from 138 boys (8).

Figure 1.
Serum testosterone levels (median and range) by LC-MS in leftover samples from blood checks before minor surgery or for the exclusion of endocrine diseases. Data from Kulle et al (8). To convert total testosterone levels to nmol/L, multiply by 0.0347
Hypothalamic neurons that express kisspeptin, neurokinin-B, and dynorphin, are thought to activate and up-regulate GnRH and initiate pubertal development (9). Adult testosterone levels are usually achieved by age 16 years, and generally range from 300-1000 ng/dL (10-35 nmol/L).
The secretion of testosterone is periodic with oscillations occurring over hours, days, and months. Frequent sampling of peripheral blood in adult men reveals small frequent moment-to-moment fluctuations (10) whereas spermatic venous blood sampling reveals robust episodes of testosterone secretion occurring about once per hour (11). Presumably because of this rapid pulse frequency and a plasma half-life of 60-90 min, only small testosterone fluctuations are generally observed in peripheral blood. Therefore. a single blood sample is usually an adequate assessment of testosterone production on a given day. There is a substantial diurnal variation in testosterone levels in adult men, however, with highest levels in the early morning, followed by a progressive decline throughout the day, reaching lowest levels in the evening (Figure 2). Thus, the time of day of blood sampling is an important consideration, and a blood sample drawn in the morning between 0800 and 1000h is recommended because reference ranges are generally based on morning values. Nadir evening levels in young men are generally 15-20% lower than morning values although the differences can be as great as 50% (12). The diurnal testosterone rhythm is blunted as men grow older (13) as well as in young men with primary testicular failure. On the other hand, the diurnal variation in testosterone is exaggerated in hypogonadal men with hyperprolactinemia (14), much like normal adolescents. There may be a seasonable variation in testosterone as well (15).
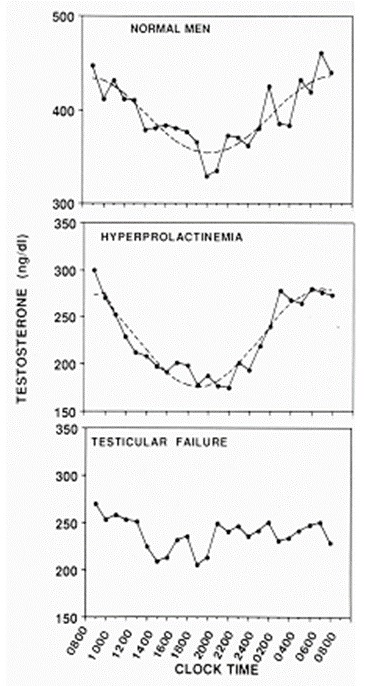
Figure 2.
24-hour testosterone concentration profiles in eugonadal men (n=5), untreated hypogonadal men with PRL-producing pituitary adenomas, and men with testicular failure (n=5). Adapted from Winters, SJ (14). To convert total testosterone levels to nmol/L, multiply by 0.0347.
There is also a slight decline in testosterone levels following a meal (16), so that some authors suggest obtaining a fasting level. In general, the results of one abnormal test should be confirmed. In one study in which multiple blood samples were taken from middle-aged men over one year, there was a relatively good correlation (r=0.85) between the plasma testosterone level in the first sample and the mean value of seven subsequent samples; however, as many as 15% of men were misclassified, with either low or normal values that were not reproducible (17).
Testosterone deficiency can be an obvious clinical diagnosis, and laboratory tests are often merely confirmatory; however, the diagnosis of hypogonadism is sometimes less straightforward. Experts and international guidelines state that the diagnosis of hypogonadism requires the presence of symptoms of hypogonadism as well as a low serum testosterone level. Symptoms of hypogonadism lack specificity, however, and are subjective. Thus, the diagnosis by laboratory testing may be more objective and less controversial. On the other hand, as discussed below, laboratory test results may be inconsistent and suffer from methodological flaws. While men with low testosterone levels tend to have symptoms of hypogonadism (18), many studies have shown that symptoms are often unrelated to testosterone levels, and many men with low testosterone levels do not complain of symptoms (19, 20).
The level of total testosterone in serum is the best single test to screen for hypogonadism because methodology has been optimized and normative data are widely available. While early assays extracted plasma steroids into organic solvents and separated them by column chromatography, those research methods generated radioactive waste and are too costly for clinical purposes. Currently most hospital laboratories use automated platforms with either a competitive or proportional two-site format. These platforms use small sample volumes to avoid matrix effects, and a monoclonal antibody with a chemi-luminescent label for detection. The accuracy of the result is highly dependent on the affinity and specificity of the antibody used, and the survey result summarized in Figure 3 demonstrates that platform immunoassays continue to provide variable results with a coefficient of variation (SD/mean) among methods of 12% at a potency of 256 ng/dL which is close to the lower limit of the usual reference range for normal men. Nevertheless, when the manufacturer provides a reference range which is based on a large number of samples, and results are age-adjusted, automated assays are probably sufficient for most clinical purposes in adult men. Assay modifications have reduced the impact of hemolysis, icterus, and lipemia on measured results.
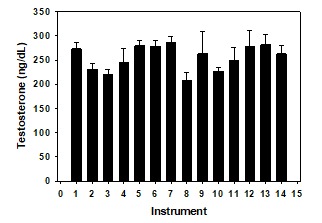
Figure 3.
Total testosterone levels (±SD) in a proficiency sample (Y-04) distributed by the College of American Pathologists, and assayed using 14 different instruments in 1556 participating laboratories in 2018. The mean (±SD) value was 256 ± 51 ng/dL. The most frequent method (#6) was used by 353 laboratories. Method #4 is mass spectrometry, and was 7% lower than the mean of all methods. To convert total testosterone levels to nmol/L multiply by 0.0347
There is a positive bias at low values in most immunoassays so that automated assays are not recommended for clinical purposes in women and children. Reference laboratories are increasingly employing liquid chromatography (LC) - tandem mass spectrometry (MS) methods (21, 22). This approach combines the resolution of chromatography with the specificity of MS, and is now viewed as a gold standard. Larger aliquots of serum are extracted with organic solvents, steroid hormones are separated by chromatography, in some assays following derivatization, and values are determined by peak area integration of testosterone-containing fractions of the column eluate. Accuracy is gained by adding an internal standard as a stable isotope of the same compound being measured in order to correct for procedural losses. LC-MS accurately detects testosterone concentrations as low as 1.0 ng/dL (23, 24). In the 2018 CAP survey (Figure 3), the coefficient of variation for the high range sample (419 ng/dL) was 10%, and was a very respectable 11% for both the mid- range (245 ng/dL) and low-range (72 ng/dL) samples among those laboratories (n=35) that used LC-MS methodology. The equipment needed is costly, however, and each LC-MS assay requires substantial analytical development and optimization, as well as highly trained personnel.
While one study of men ages 40-80 who self-reported good or excellent health found no age-related change in total testosterone levels (25), most cross-sectional and longitudinal studies have found that testosterone levels peak in the third decade, and decline as men grow older (e.g. (26, 27). Therefore, most reference laboratories present results for testosterone as a function of age. Small differences based on race and ethnicity have been found (28). One reference range for total testosterone levels in normal volunteers assayed by high-turbulence flow liquid chromatography (HTLC)-MS (22) is shown in Table 1. The decline in total testosterone in this study was roughly 0.4% per year beyond age 20, and mean T levels were 31% lower in men older than age 80 compared to men in their 20’s.
Table 1.
Impact of Age on Total Testosterone by HPLC–MS/MS in Normal Men
Age (years) | 20-29 | 30-39 | 40-49 | 50-59 | 60-69 | 70-79 | >80 |
---|---|---|---|---|---|---|---|
Mean (ng/dl) | 590 | 546 | 573 | 534 | 559 | 417 | 404 |
SD | 232 | 206 | 209 | 194 | 226 | 177 | 234 |
95th percentile | 1052 | 910 | 901 | 909 | 928 | 755 | 716 |
5th percentile | 283 | 319 | 310 | 296 | 290 | 168 | 92 |
N | 61 | 76 | 55 | 51 | 19 | 44 | 19 |
Results from Quest Diagnostics, San Juan Capistrano, CA, courtesy of M.P. Caulfield. To convert total testosterone levels to nmol/L multiply by 0.0347
Table 2 shows a second reference range with combined values from the Framingham Heart Study analyzed by LC-MS, and the European Male Aging Study and Osteoporotic Fractures in Men Study analyzed by GS-MS (29). Mean levels for men in their 80s were 20% lower than for men in their 20s representing a similar decrease of 0.33% per year. Overall values are, however, 20-40% higher than from the reference laboratory in Table 1. Thus age-dependent reference ranges are method-specific, and no generalizable “cut-point” for the diagnosis of testosterone deficiency seems possible.
Table 2.
Impact of Age on Total Testosterone Levels Measured by LC-MS or GC-MS) from Bhasin et al (29)
Age (years) | 20-29 | 30-39 | 40-49 | 50-59 | 60-69 | 70-79 | 80+ |
---|---|---|---|---|---|---|---|
Mean (ng/dl) | 713 | 656 | 617 | 611 | 569 | 567 | 570 |
95thpercentile | 1104 | 1084 | 1001 | 1059 | 965 | 1097 | 1079 |
5th percentile | 375 | 343 | 304 | 286 | 276 | 254 | 238 |
N | 220 | 660 | 872 | 788 | 493 | 289 | 26 |
To convert total testosterone levels to nmol/L multiply by 0.0347
SEX HORMONE BINDING GLOBULIN (SHBG)
Sex hormone-binding globulin (SHBG) is a glycoprotein of molecular weight 90-100,000 KDa that is produced by the liver. SHBG binds testosterone and other steroids, prolongs their metabolic clearance, and regulates their access to target tissues (24). SHBG had been measured indirectly by radio-ligand binding assays, but two site immuno-radiometric and enzyme-linked assay kits are now widely available, and automated versions have been developed. There is little effect of meals or time-of-day on SHBG.

Figure 4.
SHBG levels from birth to old age in males and females. Redrawn from Elmlinger et al (30).
A cross-sectional analysis of the impact of age on SHBG levels in males and females is shown in Figure 4. There is an increase following birth to peak values at ages 4-5 years followed by a decline that is greater in males than females (30). SHBG levels in adult male plasma range from 20-100 nmol/L, and rise slightly in old age (28); analysis by race revealed higher levels among younger AA males (31).
In normal men, SHBG binds 40-60% of the circulating testosterone with high affinity, and the level of SHBG is a major determinant of the total testosterone level (Figure 5). Single nucleotide polymorphisms in the SHBG gene influence the affinity of SHBG for testosterone and affect the level in plasma (32).

Figure 5.
Levels of SHBG and total testosterone in 28 normal men whose BMI ranged from 23-40 kg/m2. From Winters SJ et al (33).
Various clinical conditions are associated with reduced or increased SHBG levels, and thereby tend to lower or increase total testosterone concentrations (Table 3). High SHBG levels in men with HepC or hyperthyroidism may lead to very high total testosterone levels, and can be a diagnostic dilemma. Serum SHBG levels are low in obesity (34), in T2DM or the Metabolic Syndrome (MetS) (35). In fact, low SHBG is frequently used as a biomarker in epidemiology studies to predict the development of the MetS as well as type 2 diabetes mellitus (36). SHBG levels rise with weight loss (37) and when insulin resistance improves even without weight loss, e.g. with exercise. The molecular mechanism linking low levels of SHBG with insulin resistance and obesity is partly through hyperinsulinemia (38, 39) and through liver fat and cytokines which together regulate the transcription factor HNF4 alpha, a proximate activator of the SHBG promoter (40, 41) and at least 50% of genes expressed in liver.
Table 3.
Factors that Influence the Level of SHBG in Plasma
Increase | Decrease |
---|---|
hyperthyroidism | hypothyroidism |
hepatitis C | insulin resistance/NAFLD |
GH deficiency | growth hormone excess |
alcoholic cirrhosis | glucocorticoids |
acute intermittent porphyria | androgens |
first generation anticonvulsants | progestins |
estrogens, certain SERMS | nephrotic syndrome |
mitotane | genetic polymorphisms |
thinness | obesity |
genetic polymorphisms |
FREE AND NON-SHBG (BIOAVAILABLE) TESTOSTERONE
The free hormone hypothesis proposes that the biological activity of a hormone is engendered by its unbound (free) but not its high affinity protein-bound concentration in plasma. The hypothesis remains controversial, and was recently reviewed for testosterone (42).
While some authors propose that there is little extra benefit in measuring free testosterone, my view is that the total testosterone level is sometimes inadequate to determine whether testosterone deficiency or excess is present. This occurs with a borderline value, or when the clinical findings and the total testosterone concentration do not agree. I encounter this conundrum most often in obese men, those with type 2 diabetes, NAFLD, or the Metabolic Syndrome among whom total testosterone levels are generally low, and who present because they have symptoms consistent with hypogonadism. In the Boston Area Community Health (BACH) Survey, 57% of men younger than age 50 with low total testosterone levels reported “normal” sexual function compared to 33% of those with low free testosterone levels (19). Likewise, the European Male Aging Study found that men age 40-79 with low free but normal total testosterone levels more often had symptoms and signs of sexual dysfunction while men with low total but normal free testosterone concentrations were more obese but had physical or sexual symptom scores that were similar to men with normal values (20). Thus, free testosterone seemed to be more closely aligned with the symptoms and signs of adult hypogonadism than was the total testosterone level.
In older men, on the other hand, the total testosterone level may be within the normal range while free testosterones decline more dramatically. The total testosterone level may also be elevated, as in men with hyperthyroidism, hepatitis-C, and patients treated with first generation anticonvulsants. Each of these situations occurs primarily because the primary condition is associated with a decrease or increase in the circulating SHBG level.
Therefore, an assessment of free or non-SHBG bound testosterone may assist in the accurate diagnosis of androgen deficiency. This approach stems from the hypothesis that the bioactivity of circulating testosterone is due to the small percentage (1-4%) of total testosterone that circulates unbound (free testosterone) as well as the 40-50% of testosterone that circulates loosely bound to albumin and is often designated as “bioavailable-testosterone” (non-SHBG testosterone). The dissociation of albumin-bound testosterone is very rapid, and a short dissociation time is thought to allow the albumin-bound fraction to be available for uptake by cells (43). However, there is considerable debate as to which method for estimating free testosterone most accurately reflects testosterone production (42, 44).
Equilibrium dialysis is the benchmark reference method for measuring free testosterone. With the two-step approach, the free testosterone concentration is calculated from the product of the total testosterone level and the percentage that is determined to be free. H3-testosterone is added to the serum sample, and is allowed to partition between two compartments, one containing the tracer and serum sample, and the second containing buffer or an albumin solution. The compartments are separated by a semi-permeable membrane with a low-molecular weight cut-off. The protein-bound testosterone is retained while unbound testosterone, including H3-testosterone, crosses the membrane. It may take several hours to reach equilibrium, and using centrifugal ultrafiltration accelerates dialysis by forcing the unbound steroid thorough the membrane. Overall, the result is dependent on the accuracy of the total testosterone assay, and there is potential error due to temperature effects, sample dilution, and tracer impurities, among other problems. This assay is limited to reference laboratories.
Liquid chromatograph tandem mass spectrometry (LC-MS) can reliably measure hormone concentrations as low 1 pg/mL, and has been adapted for the measurement of free testosterone. With this approach, equilibrium dialysis of undiluted serum is performed against buffer overnight at 37C, or more rapidly using ultracentrifugation cartridges, and (free) testosterone is measured directly in the low molecular weight dialysate by LC-MS (45). Many reference laboratories have embraced this approach but it remains too complex and costly for most hospital laboratories.
A published reference range using the two-step equilibrium dialysis method (Table 4) reveals that free testosterone levels decline as men grow older with a difference of 36% between the youngest and oldest reference groups. Thus, the percentage change in free testosterone by this method with age (0.6%/year) exceeds the rate of decline in total testosterone (0.3-0.4%/year).
Table 4.
Reference for Free Testosterone by Equilibrium Dialysis in Normal Men
Age (years) | 20-29 | 30-39 | 40-49 | 50-59 | 60-69 | 70-79 | >80 |
---|---|---|---|---|---|---|---|
Mean (pg/ml) | 102 | 86 | 74 | 84 | 84 | 80 | 65 |
SD | 29 | 33 | 22 | 30 | 22 | 27 | 26 |
95.00% | 148 | 144 | 114 | 136 | 111 | 131 | 101 |
5.00% | 57 | 45 | 37 | 35 | 55 | 47 | 34 |
N | 49 | 55 | 48 | 36 | 14 | 36 | 17 |
Results from Quest Diagnostics, San Juan Capistrano, CA, courtesy of M.P. Caulfield. 3H-testosterone was added to a sample diluted 1:5 in assay buffer and incubated in a dialysis chamber for 20 h at 37 °C to allow the tracer to reach equilibrium with the endogenous testosterone and binding proteins. To convert total testosterone levels to nmol/L multiply by 0.0347.
Calculated Free Testosterone
A technically simpler approach used by many hospital laboratories is to calculate the free testosterone level from the levels of total testosterone and SHBG, using experimentally determined testosterone binding constants. The KD for testosterone for SHBG is most often defined as 1 x 109 L/M, and for albumin 3 x 104 L/M. These are estimates, however, and different constants will produce different results (46). Differences in the level of albumin in the sample have little impact on the calculated free testosterone, and are usually ignored. Free and non-SHBG bound testosterone levels can be computed using an internet program (www.issam.ch/freetesto.htm). There is an excellent correlation between the level of free testosterone obtained by equilibrium dialysis and the calculated free testosterone level (47), and between the calculated free testosterone and non-SHBG testosterone levels. Calculated free testosterone concentrations vary with the binding constants and algorithms employed, however, and SNPs of the SHBG gene may influence the affinity of SHBG for testosterone (32), and thereby the accuracy of the calculated free testosterone level. It has also been suggested that ligand binding to the SHBG dimer is allosteric such that occupancy of one site by a ligand alters the affinity of the second site. Furthermore, because the result is calculated from the levels of testosterone and SHBG, measurement error for either assay impacts directly on the calculated free and non-SHBG testosterone levels. A reference range (21) for free testosterone based on calculation revealed a decline of 61% from young to old adult men (Table 5), representing a rate of decline of 1%/yr. In a second study (48) the difference between young and old was only 26%, but again far exceeded the fall in total testosterone with aging which was 1.2%.
Table 5.
Influence of Age on Free Testosterone Levels in Community Men
Age (years) | 20-29 | 30-39 | 40-49 | 50-59 | 60-69 | 70-79 | >80 |
---|---|---|---|---|---|---|---|
Mean (pg/ml) | 148 | 132 | 116 | 99 | 82 | 72 | 58 |
5th Percentile | 79 | 70 | 61 | 50 | 45 | 33 | 29 |
95th Percentile | 229 | 212 | 199 | 164 | 135 | 132 | 93 |
n | 220 | 660 | 872 | 788 | 493 | 289 | 26 |
Total testosterone was measured in community dwelling men in Framingham, MA using LC-MS. SHBG was measured using a two-site immunofluorometric assay from DELFIA-Wallac, Inc. Free testosterone was calculated by the law-of-mass-action equation using of K SHBG-T of 0.998 × 109 L/mol and a K Alb-T of 3.57 × 104 L/mol. Data from Bhasin et al (29). To convert total testosterone levels to nmol/L multiply by 0.0347.
Bioavailable Testosterone
Non-SHBG-testosterone is called "bio-available” (BAT) because adding SHBG to an androgen-containing sample reduces its androgen receptor binding activity (49). This approach assumes that the testosterone bound with low affinity to albumin is active. The non-SHBG-testosterone (bioavailable) level can be determined by adding a tracer amount of 3H-testosterone to the serum sample, and selectively precipitating the SHBG-bound 3H-testosterone by adding 50% ammonium sulfate or concanavalin-A Sepharose. The 3H-testosterone that remains in the supernatant is presumed to be either free or albumin-bound, and is counted. The percentage of 3H-counts added that is in the supernatant is multiplied by the total testosterone level in order to determine the non-SHBG (bioavailable) testosterone. The assay is not readily automated, requires purified 3H-testosterone, and the complete separation of SHBG from albumin is presumed, but not verified.
Free Testosterone Index
The free testosterone index (FAI, free androgen index) represents the ratio: total testosterone/SHBG (both in units of nmol/L). This value is easy to calculate, and may be valid in serum samples from women but is not valid in men (50) because most of the SHBG in men is bound to testosterone. Like the calculated free testosterone, and BAT, the FAI is dependent on accurate values for testosterone and SHBG.
Direct Free Testosterone
The direct free testosterone assay was developed as a single-step, non-extraction method in which an125I-labeled testosterone analog competes with unbound testosterone in plasma for binding to a testosterone-specific antiserum that has been immobilized on a polypropylene assay tube. The basis for the test is that the analog has a low affinity for SHBG and for albumin. Values for normal men with this method, as a percentage of the total testosterone (0.2-0.64%), are substantially lower than the 1.0-4.0% determined by other methods. While this difference alone does not cause a problem if adequate reference ranges are available, it immediately prompted speculation concerning the accuracy of the method. Subsequent studies revealed that analog free testosterone assay results are positively correlated with the level of SHBG, much like total testosterone (33), and that free testosterone is un-measurable by analog assays in a dialysate of normal adult male serum (51). Thus, the free testosterone level determined with analog assays appears to provide essentially the same information as the total testosterone level, is often misleading (tends to over diagnose hypogonadism), and is not recommended. The 2018 College of American Pathologists survey revealed that only 7% of participating laboratories continue to use this method, and it is has nearly disappeared from research articles related to testosterone.
Testosterone in Saliva
The level of testosterone in saliva is positively correlated with the plasma free testosterone concentration. Salivary samples are easily collected, usually by a non-stimulated drool. Both extraction and non-extraction immunoassay methods are available although LC-MS is being increasingly employed (44). Usual values in adult men are 150-500 pmol/L (40-145 pg/ml). Salivary testosterone assays are a useful research tool for field studies and other settings in which blood sampling is impossible or impractical. While androgens are stable for a few days in untreated saliva, method artifacts may occur, and careful assay validation and quality control are essential. Salivary testosterone assays have not been recommended for clinical purposes.
Cell-Based Reporter Bioassays
Cell-based reporter bioassays have been developed to analyze androgen bioactivity in biological samples. A stable cell line is created by transfection with plasmids encoding the human androgen receptor and a reporter system containing an androgen-responsive gene such as the mouse mammary tumor virus (MMTV)-luciferase reporter. When cells are stimulated with androgens, luciferase activity is increased dose-dependently (52), These assays remain investigational.
DIHYDROTESTOSTERONE
5 alpha reduction of testosterone to the more potent androgen dihydrotestosterone (DHT) is essential for fetal male genital development. T is converted to DHT by at least two steroid 5α-reductase (5AR) isoenzymes, 5AR types 1 and 2. 5AR-1 is found in liver, skin, brain, ovary, prostate, and testis whereas 5AR-2 is expressed in prostate, seminal vesicle, epididymis and skin. Approximately 20% of the circulating DHT in men is secreted by the testes, and the remainder is derived from the bioconversion of testosterone in tissues. Because of a high level of expression of 5AR-2 in prostate, testosterone is effectively converted to DHT in that tissue, in which the level of DHT exceeds the peripheral blood concentration by 5-10-fold. The concentration of DHT in adult male serum is only about 10% of the value for testosterone, however. Therefore, an assay with negligible cross-reactivity with testosterone is needed for an accurate result. An LC-MS method is now used by most reference laboratories. With gradient elution, the separation is totally complete (53). Patients with 5α-reductase deficiency type 2 have ambiguous genitalia, and are generally detected as neonates, although a few patients have only microphallus or cryptorchidism. A rise in testosterone but not DHT following hCG stimulation, producing a ratio of more than 10:1, is characteristic in most of these patients (54). However, DHT production by 5AR1 can reduce the reliability of the ratio for diagnosis (55). The diagnosis is most often made by urinary steroid profiling by GC-MS, together with mutational analysis. DHT levels are often measured in epidemiological studies and in clinical research on prostate cancer and its treatments, and testosterone treatment results in a dose-dependent increase in serum DHT concentrations (56). Yet DHT levels are probably not useful in most clinical situations.
ESTRADIOL AND ESTRONE
Estrogens are important male hormones. They regulate the hypothalamic-pituitary-testicular axis, influence the function of the testes and prostate, increase growth hormone and IGF-1 secretion, modify lipid metabolism and other hepatic proteins, and play an important role in male skeletal health, body fat, and perhaps sexual functioning (57, 58). Serum levels of estradiol and estrone are often measured in men with gynecomastia (59) or with unexplained gonadotropin deficiency. These conditions are rarely, but occasionally, due to estrogen-producing tumors, or to acquired (60) or genetic (61) abnormalities in which estrogen production is increased. Moreover, the accurate measurement of estradiol may be helpful when SERMs, aromatase inhibitors, or hCG are used are used to increase testosterone levels, and in research on the role of estrogens in males (58, 62). Estradiol is produced from testosterone, and estrone is produced from androstenedione, by aromatase P450, the product of the CYP19 gene. This enzyme is expressed in Leydig cells and in the adrenal cortex, as well as in adipose- and skin-stromal cells, aortic smooth muscle cells, kidney, skeletal muscle cells, and the brain. The promoter sequences of the P450 aromatase genes are tissue-specific, but the translated protein appears to be the same in all tissues. Increased aromatase expression in adipose and skin stroma with obesity is the most common cause for mild estrogen excess in men. Interestingly, most studies of the age-associated decline in testosterone levels do not find a parallel fall in plasma estradiol levels perhaps because of increasing aromatase activity and fat mass as men grow older (63).
Because of low levels in males, traditional immunoassays for estrogens employed large volumes of plasma (2-5 ml) that were extracted with organic solvents. Because those assays are time-consuming and expensive to perform, non-radioactive automated methods that were optimized for the higher values normally found in pre-menopausal women were employed. However, the very small sample volumes used in automated assays may produce unexpectedly high values. Results for estradiol in male plasma determined in platform assays are unreliable. The lack of agreement between laboratories is shown in Figure 6 which depicts the results for the low potency proficiency sample (mean 199 pg/mL) distributed in 2018 by the College of American Pathologists to 1450 participating laboratories. While many assays produced similar results, overall values ranged from 151 to 415 pg/ml (554-1523 pmol/L), with a coefficient of variation of 33%, compared to the goal of ±12.5%. The mean value of the sample far exceeds normal levels in males, however, and testing at a lower potency would no doubt have produced even more dramatic differences.

Figure 6.
Estradiol levels (±SD) in a mid-range proficiency sample (Y-05) distributed by the College of American Pathologists assayed using 15 different instruments in 1499 participating laboratories in 2018. The most frequently used (#6) was used by 341 laboratories. Mass spectrometry assays (n=11) are represented in column 4.
Mass spectrometry assays for estradiol and estrone, with a limit of detection of 1 pg/ml, have replaced conventional radio-immunoassays in most reference laboratories (24), and are highly recommended. The result with LC-MS is lower than with many immunoassays which presumably also detect interfering substances. Moreover, the between laboratory coefficient of variation for laboratories using MS methods (column 4) was also acceptably low at 10.3%.
Most of the circulating estradiol in men is loosely bound to albumin or is unbound (64), and only about 20% is thought to be bound to SHBG. Therefore, the serum level of SHBG was not predicted to appreciably influence the actions of estradiol. Measurement of free estradiol may be useful, however (65). In a series of studies, calculated non-SHBG-bound estradiol levels correlated more strongly with low bone mineral density and with indexes of high bone turnover in older men than did levels of total estradiol (66). On the other hand, increased mortality over 12 years of follow-up was seen among those with either low or high total as well as calculated free estradiol levels at baseline when compared to those in the middle tertile (67). Akin to testosterone, non-SHBG (bioavailable) estradiol levels can be determined by ammonium sulfate precipitation using 3H-estradiol, or by equilibrium dialysis with or without using LC-MS. Estimated levels are < 1 pg/mL. Calculated values require an accurate total estradiol assay, affinity constants are less certain than for testosterone, and the influence of testosterone and other steroids that also bind SHBG on the calculated estradiol level is no doubt important. Age-specific free estradiol levels were reported but total estradiol was measured using an electro-chemiluminescence immunoassay (68); results using LC-MS methods are needed.
GONADOTROPINS
FSH and LH, together with TSH and hCG, form a closely related family of heterodimeric glycoprotein hormones. Each consists of a common α-subunit that is non-covalently linked to a specific β-subunit. The α–β dimer is held together by a ‘seatbelt’ structure formed by the C-terminal amino acids of the β-subunit wrapped around the α-subunit. Both subunits have asparagine-linked carbohydrate chains (2 for human α-subunit, 2 for FSH-β, and 1 for LH-β). The oligosaccharides project from the peptide skeleton, and by shielding of epitopes and altering the tertiary structure of the hormone, the sugars may impact receptor activation and bioactivity as well as antibody binding. Glycosylation also prolongs hormone clearance.
Most laboratories utilize fully automated, commercial assay systems for peptide hormones including LH and FSH. Detection is generally by chemiluminescence, avoiding the use of radioactive tracers. Many assays achieve specificity by utilizing a biotinylated monoclonal antibody to the α-subunit as a capture antibody. A second monoclonal antibody to the β-subunit is labeled with an organic ester that produces chemiluminescence in the presence of hydrogen peroxide (indicator antibody). Various pituitary and recombinant preparations are used for the standard curve but most assays are calibrated in terms of IU/L of International Reference Preparations (IRP) of highly purified human LH and FSH. The various standards have differing sugar sequences and branch patterns producing some variation in results between laboratories and assays, however, differences are relatively small. When defined as a level of precision of replicate determinations of <10%, the sensitivity of these assays approximates 0.1 U/L.

Figure 7.
Serum LH and FSH levels in normal males measured by an ICMA assay. Redrawn from Resende et al. (69).
Figure 7 shows mean levels of LH and FSH across the stages of pubertal development in boys measured with an automated ICMA (69). Values with an immunofluometric assay were slightly higher. FSH levels tend to exceed LH before puberty in boys, and both gonadotropins rise progressively during puberty with substantial overlap among the various pubertal stages.
Prepubertal children have low amplitude but discrete pulsatile patterns of LH secretion that are amplified during sleep as puberty begins (70). In adult men, LH is released in robust pulses every 1-2 h throughout the day and night with within subject variation in pulse height and between-pulse interval (Figure 8). In part because of pulsatility, the normal range for LH is wide, with typical ranges for adult men in terms of 2nd IRP of 1.6–8.0 IU/L for LH and 1.3–8.4 IU/L for FSH. Because of this pulsatility, pooling of 3 samples taken 20–30 min apart may provide a more accurate estimate of a person’s LH value than does a single sample.
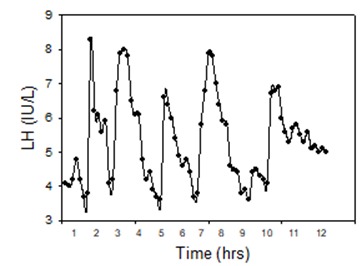
Figure 8.
Pulsatile patterns of LH secretion. Blood samples were drawn every 10 min for 12h starting at 0800 h from a 32-year-old normal man whose testosterone level was 474 ng/dL. Winters, SJ (unpublished).
Men with elevated levels of LH and FSH generally have testicular damage. Testes are usually small, there is oligo or azoospermia, and testosterone levels are low. Circulating estradiol levels are generally normal, however, in part because testicular aromatase is stimulated by the high level of LH. In some men with high LH and FSH levels, testosterone levels remain normal, sometimes because of elevated SHBG. The term “mild” or “compensated” hypogonadism is often applied (71). The diagnosis of gonadotroph adenoma is sometimes entertained, but long-standing infertility and small testes are a clue to the correct diagnosis. Much like the controversy surrounding thyroid hormone replacement in individuals with “subclinical” hypothyroidism, men with compensated hypogonadism who are not seeking fertility, mighty be viewed as candidates for testosterone replacement even though testosterone levels are within the reference range. This is often the case in teenagers with Klinefelter syndrome (72).
As men grow older, there is a decrease in sperm quality, the testes tend to be smaller, and there are fewer germ cells with more hyalinization and thickening of the tunica albuginea. Inhibin-B levels fall and FSH levels are often elevated. Mean LH levels also rise, but most often remain within the reference range (73).
Men treated with SERMs or aromatase inhibitors (74), and the rare men with inactivating mutations of the estrogen receptor-α (75) often have elevated LH and normal or elevated testosterone levels. These findings imply that estradiol is a major mediator of testicular negative feedback control of GnRH-LH secretion. Elevated LH and testosterone levels are also characteristic of men with androgen insensitivity syndromes (76) and men treated with anti-androgens (77), and reveal that there is additional negative feedback control of gonadotropins through the androgen receptor.
FSH levels may be increased selectively because FSH production is regulated not only by GnRH and gonadal steroids but also by a paracrine control mechanism that involves pituitary activin and follistatin, and gonadal inhibin (see below). When Sertoli cells fail to function normally, inhibin-B production declines, and the paracrine effects of pituitary activin stimulation of FSH-β gene expression is unopposed. Most men with high FSH levels have small testes and oligo- or azoospermia (78); however, FSH levels may sometimes be within the reference range (note that in some instances the reference range for FSH may be too high and some experts feel that the upper level for FSH is 8IU/L) in infertile men with severe damage to the germinal epithelium (79) who may have either normal sized or small testes. In these cases, testicular biopsy is sometimes needed for a definitive diagnosis which is most often maturation arrest at the primary spermatocyte stage.
An elevated serum FSH level may rarely indicate an FSH-producing pituitary adenoma (80). These tumors are generally macroadenomas that present with headache and a visual disturbance, and often co-secrete free α-subunit. The testes may occasionally enlarge (81). The LH level is usually suppressed even when the tumors are LH-β immunoreactive, and testosterone levels are generally low. LH secretion by a pituitary tumor is very rare (82, 83). Most pituitary adenomas that are immunoreactive for LH or FSH are clinically silent with little or no hormone secretion.
The diagnosis of hypogonadotropic hypogonadism (HH) is based upon finding a low serum testosterone level with inappropriately low/normal LH and FSH concentrations. It is important to verify testosterone deficiency since a low SHBG level may result in the misdiagnosis of testosterone deficiency, and low LH may overlap with the reference range because of the pulsatile nature of LH secretion. Thus, the diagnosis of secondary hypogonadism (84) is sometimes problematic. The differential diagnosis of true hypogonadotropic hypogonadism is broad, and includes congenital HH due to a variety of genetic mutations including Kallmann syndrome (85), and acquired disorders such as prolactinoma, other sellar and suprasellar tumors or cysts, infiltrative diseases and vascular causes, iron overload, head trauma and others. HH in childhood limits pubertal development while in adults HH results in the regression of secondary sexual characteristics, albeit slowly.
Isolated deficiency of FSH due to inactivating mutation in the FSH-β gene is a rare disorder (86-88). Affected men have been azoospermic, with borderline or low testosterone and increased LH levels. Pathological examination revealed narrowed seminiferous tubules, decreased Sertoli cell numbers, absence or aplasia of germ cells, and Leydig cell hyperplasia. Most mutations disrupt the ability of the mutant FSH- β to combine with the alpha-subunit. The low testosterone and increased LH levels may be explained by impaired signaling from the dysfunctional seminiferous tubules to Leydig cells (89). A few hypogonadal men with inactivating mutations of the LH-β gene have also been described (90-92). Immunoreactive LH may be present or absent in serum depending on the nature of the mutation, but if present is bioinactive (92). Testosterone is low, and FSH is increased. As placental hCG, rather than LH, stimulates male sexual differentiation, affected males have a normal male phenotype at birth while bioactive LH deficiency results in testosterone deficiency, and prevents normal puberty.
Two-site assays can occasionally be "too specific". Polymorphisms that affect immunoassay detection by monoclonal antibodies may lead to misdiagnoses. For example, there is a relatively common polymorphism in the LH-β gene that is characterized by two point mutations in codons 8 and 15 resulting in two amino acid substitutions and an extra glycosylation site (88). Even though men and women with the LH variant appear to be normal and fertile, the serum LH level is low or undetectable using certain monoclonal antibodies whereas the result with other assays is normal. Clearly, when a man with a low or undetectable LH with a normal testosterone level is encountered, or a disparity between LH and FSH levels cannot be readily explained, a second assay method should be used.
Various glycoforms of LH and FSH with structurally heterogeneous glycans are found in the pituitary and in the circulation, and glycosylation has been shown to influence hormone clearance and biological activity (93). Both sex steroids and activin have been reported to affect gonadotropin glycosylation. Highly sialylated glycoforms, with an acidic pH, tend to have a longer circulating half-life whereas the more alkaline forms tend to exhibit greater bioactivity in vitro. There is evidence that LH and FSH glycosylation is physiologically important. The ideal immunoassay would detect accurately only the total bioactive LH or FSH in the sample, but clearly this goal is difficult to accomplish. As an alternative approach, in vitro bioassays for LH and FSH can be used to assess the function of the gonadotropins. An in vitro bioassay based on the production of testosterone by cultured mouse or rat Leydig cells (94) was developed to assess LH function, and the production of estradiol by rat granulosa cells or immature Sertoli cells can be used to assay FSH bioactivity (95). Bioassays based on cAMP production by cell lines stably expressing gonadotropin receptors, with quantification using cAMP-responsive promoters linked to a luciferase reporter, have also been developed (34). While useful for the study of the biological properties of recombinant or purified proteins, the clinical use of these assays is limited by the nonspecific effects of serum. In fact, many findings reported in patients using in vitro LH bioassays were found subsequently to be methodological artifacts.
Glycoprotein α-Subunit
Glycoprotein α-subunit is secreted in bursts that coincide with LH secretory episodes, implying pre-eminent regulation by GnRH (96) Accordingly, serum α-subunit levels increase at puberty (97) and are elevated in men with testicular failure and in postmenopausal women. α-Subunit is also expressed in thyrotrophs, and levels are increased in patients with primary hypothyroidism (98).
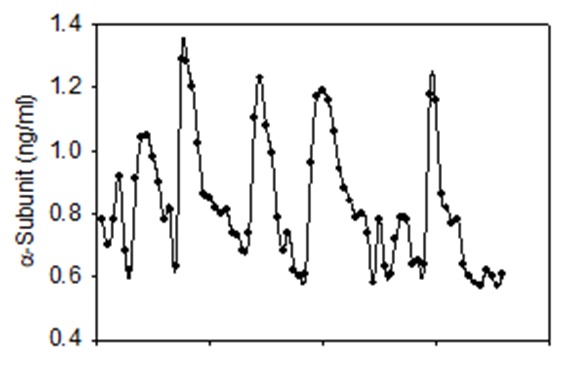
Figure 9.
Pulsatile patterns of α-subunit secretion. Blood samples were drawn every 10 min for 12h starting at 0800 h from a 32-year-old normal man whose testosterone level was 474 ng/dL. Winters, SJ (unpublished)
α-Subunit levels are low, but measurable, in normal children and in patients with congenital hypogonadotropic hypogonadism. Because peak α-subunit levels after GnRH stimulation tend to be lower in IHH patients than in prepubertal boys, this test has been used to distinguish between these two patient groups, although some overlap occurs (99). α-Subunit is produced by as many as 20% of pituitary adenomas (100) often together with FSH or sometimes GH. Because it is cleared by renal excretion, α-subunit levels are high in patients with renal failure (101) .
INSULIN-LIKE FACTOR 3
Insulin-like factor-3 (INSL3) is a peptide hormone member of the relaxin-insulin hormone family that is secreted by Leydig cells following LH stimulation. INSL3 affects testicular descent through effects on the gubernaculum (102) and plays a role in spermatogonial differentiation. At the time of puberty, INSL3 levels rise in parallel with testosterone (103). INSL3 concentrations in the blood of normal adult men are approximately 1 ng/mL (0.4-1.5 ng/mL). Patients with Leydig cell dysfunction have lower levels, and the very low concentrations in men with hypogonadotropic hypogonadism increase following hCG administration (104) but are unaffected by testosterone treatment (105).
INHIBIN-B
Inhibin, a glycoprotein hormone produced by the testes as well as the ovaries, is responsible for the selective negative feedback control of FSH secretion, and functions as an intra-gonadal regulator (106). Most evidence supports the idea that inhibin decreases FSH-β mRNA levels by blocking pituitary activin-stimulated FSH-b transcription (107). Inhibin is a 32-kDa heterodimer composed of an α-subunit, and one of two β subunits, βAor βB. Inhibin-B (α-βB) is the form produced by testicular Sertoli cells; inhibin-A (α-βA), produced by the corpus luteum and placenta, is undetectable in adult male plasma. Higher molecular weight forms of the uncombined inhibin α-subunit that lack bioactivity are released into the circulation in excess of dimeric inhibin-B.
The Gen II two site inhibin-B ELISA assay uses a capture antibody raised to a peptide from the βB-subunit, and a biotinylated detection antibody raised to a peptide from the inhibin α-subunit (108). Wells are then incubated with a streptavidin-labeled horseradish peroxidase followed by the substrate tetramethylbenzidine which produces a colorometric signal that is proportional to the amount of inhibin-B in the sample. The assay is not automated and no performance statistics could be located. Older assays were more complex, and added methionine and an oxidation step with hydrogen peroxide to facilitate capture, and heating with sodium dodecyl sulfate solution to enhance specificity.
Inhibin-B is produced by the fetal testis and is measurable in serum at term. Levels increase substantially in newborns coincident with the rise in gonadotropins and testosterone (mini-puberty), remain elevated for 2-4 months and then decline (109). In contrast to barely detectable levels of gonadotropins and testosterone during childhood, however, circulating inhibin-B is readily measurable in sera from prepubertal boys, implying that production is partly gonadotropin-independent. Serum inhibin-B levels increase to adult values at the time of puberty, and decline as men grow older (110). Serum inhibin levels are partly determined by gonadotropin stimulation (111) but based on studies in monkeys, also reflect Sertoli cell number (112). With the updated assay, results for normospermic men were (2.5-95th percentile) 32-416 with a median value of 174 pg/mL (113).
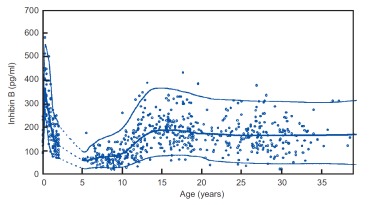
Figure 10.
Inhibin B levels in males in relation to age. Reprinted from Andersson A-M (114) with permission of the publisher (Elsevier).
Although there is no remarkable pulsatile fluctuation in circulating inhibin-B levels, there is a diurnal variation in adult men with the highest values in the morning and nadir values approximately 35% lower in the evening. This diurnal pattern parallels that of testosterone (115).
Inhibin has been extensively studied as a biomarker of spermatogenesis. In keeping with its function to suppress FSH production, circulating inhibin-B levels are inversely correlated with FSH levels in adult men, and are more strongly correlated inversely when values from men with primary testicular failure are included in the analysis (111). Inhibin-B levels are higher in fertile than infertile men but there is substantial overlap between the groups (113, 116). There is also a demonstrable, albeit weak, positive correlation with sperm count (117), and with the germ cell score in testicular biopsy specimens among infertile men (118). Inhibin-B levels are low in men with testicular failure, and are very low in men with Klinefelter syndrome in whom seminiferous tubules are hyalinized and Sertoli cells are essentially absent (119). Low but measurable inhibin-B levels in men following chemotherapy (120) or testicular irradiation (121), some of whom develop germinal cell aplasia, suggest that germ cell factors regulate SC inhibin production. Low inhibin-B levels predict persistent azoospermia in men with testicular cancer who undergo orchidectomy, chemotherapy and irradiation (122). Serum inhibin levels changed little in normal men who participated in a male contraceptive clinical trial of testosterone together with a progestin, and developed azoospermia or severe oligospermia (123).
Inhibin-B levels have been studied as a biomarker in the male partners of infertile couples undergoing testicular sperm extraction (TESE) as a predictor of success of intra-cytoplasmic sperm injection (ICSI). While mean inhibin B levels tend to be lower (and FSH higher) in men with no sperm found at TESE, there is no level of inhibin-B that reproducibly predicts either the presence or absence of spermatozoa in TESE samples, or successful in vitro fertilization (124). Thus, measurement of inhibin-B is not recommended in the decision making for fertility potential among azoospermic men undergoing TESE and ICSI. Further, very large doses of FSH are needed to increase circulating inhibin-B levels in men, so that an FSH stimulation test for inhibin-B is also not helpful in a clinical evaluation of hypospermatogenesis.
Inhibin-B levels are reduced in gonadotropin-deficient men (125). In those with the complete form of congenital hypogonadotropic hypogonadism, values are lower than in men with partial gonadotropin deficiency who have some spontaneous pubertal development. This quantitative difference is partly due to a presumed larger mass of Sertoli cells (as reflected by larger testicular size) in the latter group of men. Low inhibin-B levels have been reported to differentiate boys with constitutional delay of puberty (CDP) from those with congenital hypogonadoptropic hypogonadism (CHH) with 80-100% sensitivity and specificity (126). While plasma inhibin B concentrations in CHH and CDP may overlap, in one study of boys age 14-18 years with delayed puberty, among those who were genital stage 1 (testis volume ≤ 3ml), inhibin-B levels <35 pg/ml predicted persistent hypogonadism (testosterone levels < 3 nmol/L), and presumably the diagnosis of CHH, after 2 years of follow-up (127). Inhibin-B is undetectable in most boys with congenital anorchia as in castrates, and is therefore a useful test to help distinguish these patients from boys with intra-abdominal testes (128).
High inhibin-B levels have been reported in a few boys with FSH-producing pituitary tumors (129), in patients with Sertoli cell tumors (130) and in boys with McCune Albright syndrome with macro-orchidism and autonomous function of Sertoli cells (131). Adrenal tumors often express the inhibin α-subunit, and there is one report of high serum dimeric inhibin level in an adult man with an adrenal neoplasm (132).
ANTI- MULLERIAN HORMONE
Anti-Mullerian hormone (AMH, also known as Mullerian inhibitory hormone) is a 140 KDa homodimeric member of the TGF-β family of growth and differentiation factors (133). It is produced by fetal Sertoli cells and causes regression of Mullerian structures during male sexual development (134). AMH production increases in response to FSH and is inhibited by androgens. AMH is readily detectable in the serum of prepubertal boys in concentrations of 10-70 ng/ml, and declines to levels of 2-5 ng/ml with entry into adolescence (135). AMH remains detectable at low values throughout adulthood (104) but how AMH functions in males beyond fetal life is uncertain. AMH levels are elevated in untreated men with congenital hypogonadotropic hypogonadism and decline following treatment with hCG (136). AMH is absent from the plasma of most prepubertal boys with congenital anorchia but is generally detectable in boys with bilateral cryptorchidism (134). Therefore, measuring AMH is useful in evaluating boys with non-palpable gonads. AMH levels are low in men with seminiferous tubular failure but overlap with normal in men with oligospermia, and do not predict testicular sperm retrieval for ICSI (137). Levels tend be low in obesity (138),

Figure 11.
Serum concentrations of AMH in healthy males from birth (cord blood) to age 69 years. From Aksglaede L et al (139). Red dots are values from men with bilateral anorchia. Republished with permission of The Endocrine Society.
FUNCTIONAL TESTS
hCG Stimulation
Human chorionic gonadotropin (hCG) can be used as a test agent to examine Leydig cell steroidogenesis in prepubertal boys who secrete little or no endogenous gonadotropins. A variety of protocols have been used. In one study, serum testosterone levels rose to greater than 300 ng/dL (10.5 nmol/L) in healthy prepubertal boys administered hCG 1500 IU intramuscularly every other day for seven doses. The effect of hCG to increase circulating levels of testosterone, precursor steroids and DHT can be used in conjunction with mutation analysis to help evaluate patients with a 46,XY karyotype who have ambiguous genitalia which may be due to androgen insensitivity, defects in testosterone biosynthesis, or 5α-reductase deficiency (140). When the basal and hCG-stimulated profile indicates accumulation of steroid precursors upstream of an enzymatic defect, sequencing can be performed to identify the disorder. A raised serum testosterone to DHT ratio would suggest the diagnosis of 5α-reductase deficiency type 2. However, DHT production by 5α-RD1 can reduce the reliability of the ratio for diagnosis (55).
Testosterone levels are generally unaffected by hCG in boys with congenital bilateral anorchia but increase in boys with bilateral intra-abdominal testes (141). Therefore, this test, together with measurement of inhibin-B and AMH, help establish which boys have intra-abdominal testes and should undergo laparoscopy, and orchidopexy or orchiectomy (128).
Adult men with primary testicular failure have elevated endogenous serum LH concentrations, and hCG will predictably increase serum testosterone levels less in these men than in eugonadal men. In gonadotropin-deficient men, the testosterone response to short-term administration of hCG is also blunted because Leydig cell steroidogenic enzymes are down-regulated. Overall, hCG testing nowadays provides little clinically useful information in either group of adult men.
Blockade of Steroid Hormone Biosynthesis and Action
Lowering circulating sex steroid levels with pharmacological inhibitors, or blocking steroid hormone action with receptor antagonists, can be used to assess the integrity of the hypothalamic-pituitary-testicular unit as a research tool, and clinically as a treatment for adult men with adult onset hypogonadism (142).
Ketoconazole, a competitive inhibitor of cytochrome P450 cholesterol side chain cleavage (P450scc) and C17/20 lyase in the biosynthetic pathway to testosterone is used in the treatment of endemic mycoses and off-label in other conditions including Cushing syndrome, but causes a dose-dependent reduction in circulating testosterone and estradiol levels. (143). Because ketoconazole also lowers cortisol production, glucocorticoids are co-administered with ketoconazole to prevent the symptoms of cortisol deficiency.
Estrogens produced by the testes, peripheral tissues, and the CNS play an important role in the physiological feedback regulation of gonadotropin secretion in men. Selective estrogen response modulators (SERMs) bind to estrogen receptors (ER-α and/or ER-β) and exert estrogen-like effects, or inhibit estrogen effects, in a tissue-specific manner. SERMs also have non-classical extra-nuclear (membrane) signaling mechanisms in certain cells. By blocking estradiol negative feedback, most SERMS, such as clomiphene (144) and tamoxifen, increase plasma LH and FSH levels. Similarly, aromatase inhibitors (145) such as anastrazole, reduce circulating estradiol levels, and increase LH in men. Dosages of 25 mg/day of clomiphene produced a mean two-fold increase in serum testosterone levels in adult men (Helo 2015). (142)., and while higher doses are more effective, estradiol levels may increase, and gynecomastia and galactorrhea may occur (144). Two-fold increments in LH were produced by anastrazole 10 mg daily within 3-4 days. Both blocking estrogen negative feedback and decreasing estradiol production increased LH pulse frequency indicating an effect of estradiol on the GnRH pulse generator. A normal response implies functional integrity of GnRH-LH-testosterone pathways; however, responses among normal subjects are variable, and diagnostic tests using SERMS are not thought to be useful clinically. So far, these agents are not FDA-approved for any use in men.
Nonsteroidal antiandrogens increase serum LH levels in men by blocking the androgen negative feedback effect on GnRH secretion (146) and can be used to test GnRH-LH integrity.
GnRH Test
GnRH is used as a research tool to examine the responsiveness of gonadotrophs to their physiological stimulus. In normal adult men, the intravenous administration of 100 µg of GnRH increases serum LH levels three- to- six-fold while serum FSH levels rise by about 50%. Generally, the total and incremental release of LH and FSH following GnRH administration is directly proportional to the basal hormone level, although exceptions do occur.
The GnRH test was introduced as a method to diagnose hypogonadism, and to distinguish hypogonadism due to hypothalamic from pituitary disorders. The LH and FSH response to GnRH is subnormal when gonadotrophs are damaged or destroyed by pituitary tumors or by other pathologies. However, the gonadotropin response to stimulation with GnRH is also diminished in patients with GnRH deficiency since GnRH up-regulates its receptor on gonadotrophs, as well as the expression level of each of the gonadotropin subunit genes and thereby LH and FSH production. Thus, the LH response to GnRH stimulation is attenuated in these patients even though the pituitary is essentially normal. Therefore, when evaluating gonadotropin deficient adult men, the GnRH test generally provides little information beyond that of the basal testosterone, LH and FSH levels, and is not recommended for clinical purposes.
GnRH analog (naferelin, leuprolide, buserelin, triptorelin) testing may help distinguish prepubertal boys with constitutional delay of puberty (CDP) from those with congenital hypogonadotropic hypogonadism (147-149). These agents bind the GnRH receptor with higher affinity and have a longer circulating half-life than does native GnRH. The LH and testosterone responses at 4h and 24h in boys with CDP generally exceed those of HH. In one study, prepubertal boys age 13.7-17.5 years with testicular volume ≤ 4ml whose LH level at 4h following 0.1 mg sc triptorelin exceeded 5.3 U/L all progressed to testicular enlargement, testis size ≥ 8 ml, over 18 months. Those boys with lower LH basal ≤ 2U/L and stimulated ≤5.3 mIU/ml values did not progress to this level. The CHH group also had low levels of inhibin-B (<111 pg/ml) at baseline (127). Thus, the test seems to be of use in evaluating prepubertal boys with delayed puberty. In patients with CHH who are homozygotes or compound heterozygotes for mutations of the GnRH receptor gene, GnRH binding to its receptor may be either absent or reduced in affinity, or receptor signal transduction may be impaired, and the LH response to GnRH stimulation in these patients may be absent or reduced. The LH response in those with partial hypogonadotropic hypogonadism overlaps with the normal response (150), and while these tests are helpful so far there is no gold-standard diagnostic test to fully differentiate boys with CHH from those with CDP.
SEMEN ANALYSIS
Laboratory testing for men who present for an evaluation of infertility begins with the semen analysis (151). Most laboratories report the semen volume, the sperm density (million/mL), motility (% with progressive motion on the microscopic slide), and the sperm morphology (% with oval-shaped sperm heads), and follow WHO guidelines in their methods and reports (152). The test helps predict fertility potential but is not a test of fertility since there is substantial overlap between results for fertile and infertile men (153). Moreover, lack of complete adherence to standardized methods, and insufficiently skilled laboratory staff, may substantially limit the diagnostic value of the semen analysis. The sample can be collected by masturbation, or by interrupted intercourse, generally after 2-3 days of abstinence from ejaculation. Most of the sperm are in the first portion of the ejaculate so it is important to collect a complete sample. Condom collection is less desirable since condoms contain spermicides, but if so, the sample should be immediately placed into a sterile jar. It is important to analyze the sample within 60 min of collection, as motility decreases over time. In cold climates, the sample should be kept warm in transit by holding it within outer clothing adjacent to the body. Even with optimized collection methods, there is a physiological day-to-day variation in sperm production, so 2-3 samples may provide more insight that a single sample. A man can be considered to be sterile if there are no sperm in the ejaculate (azoospermia).
Table 6.
WHO Guidelines for an Adequate Semen Analysis
Semen volume | ≥1.4 mL |
Sperm concentration | ≥15 million/mL |
Total sperm output | ≥40 million sperm |
Sperm progressive (total) motility | ≥32 (≥40%) |
Sperm morphology | ≥4% |
GENETIC TESTING
Assisted reproductive techniques have enabled azoospermic and severely oligospermic (<5 million/mL) men to reproduce. Therefore, there is a need to identify and discuss the consequences of possible mutations with the infertile couples. Genetic abnormalities are found in 3% to 5% of men with severe oligosperma, and 14% to 19% of men with non-obstructive azoospermia (NOA). The peripheral blood karyotype should be analyzed in these men unless there is a likely cause for hypospermatogenesis (e.g. cancer chemotherapy or orchitis). Of those with azoospermia, 2/3 have Klinefelter syndrome (47,XXY and mosaics) (154). Rarely, azoospermic men have a 46,XX chromosome constitution with Y-chromosome material detectable on the X-chromosome by FISH analysis using an antibody to the transcription factor encoded by the SRY gene. In studies of various ethnic groups, 4-15% of men with idiopathic non-obstructive azoospermic and 1–10% of men with severe oligospermia have microdeletions of the AZF regions of the Y chromosome (155, 156). Thresholds of 0.5-5 million sperm/mL have been suggested for genetic testing for higher specificity and overall lower cost (157) although some authors would like to see all infertile men tested. Microdeletions (undetectable in standard karyotypes; YCMD) are detected by PCR and may partially or completely eliminate the azoospermia factor (AZF)a or the AZFb/c region including genes that are important for spermatogenesis. YCMD are detected by PCR. Couples are counselled that male offspring will inherit the AZF microdeletion, and will be at risk for infertility. In azoospermic men Y deletion testing is essential prior to considering surgical sperm retrieval because AZFa , AZFb or AZFB+c deletion are associated with very severe spermatogenic defects such as germ cell arrest or Sertoli cell only syndrome with an essentially zero prospect of success (160). On the other hand, even azoospermic men with AZFc deletions have ~ 50% chance of surgical sperm recovery (160).
Obstructive azoospermia may be recognized by a low-volume ejaculate with low pH. The testes of these men are generally normal in size, and FSH and inhibin-B levels are in the reference range. Obstruction can be demonstrated by ultrasound (158). If bilateral absence of the vas deferens (CBAVD) is found, approximately 75% of men will have cystic fibrosis, sometimes with little respiratory or pancreatic disease (159). When Cystic Fibrosis is diagnosed by genetic testing, the next step is to screen the female partner for Cystic Fibrosis gene abnormalities in order to assess the risk to any offspring.
REFERENCES
- 1.
- Rosner W., Vesper H., Endocrine S. Toward excellence in testosterone testing: a consensus statement. The Journal of clinical endocrinology and metabolism. 2010;95:4542–4548. American Association for Clinical, C., American Association of Clinical, E., Androgen Excess, P.S., American Society for, B., Mineral, R., American Society for Reproductive, M., American Urological, A., et al. [PubMed: 20926540]
- 2.
- Khera M., Adaikan G., Buvat J., Carrier S., El-Meliegy A., Hatzimouratidis K., McCullough A., Morgentaler A., Torres L.O., Salonia A. Diagnosis and Treatment of Testosterone Deficiency: Recommendations From the Fourth International Consultation for Sexual Medicine (ICSM 2015). J Sex Med. 2016;13:1787–1804. [PubMed: 27914560]
- 3.
- Bhasin S., Brito J.P., Cunningham G.R., Hayes F.J., Hodis H.N., Matsumoto A.M., Snyder P.J., Swerdloff R.S., Wu F.C., Yialamas M.A. Testosterone Therapy in Men With Hypogonadism: An Endocrine Society Clinical Practice Guideline. The Journal of clinical endocrinology and metabolism. 2018;103:1715–1744. [PubMed: 29562364]
- 4.
- Mulhall J.P., Trost L.W., Brannigan R.E., Kurtz E.G., Redmon J.B., Chiles K.A., Lightner D.J., Miner M.M., Murad M.H., Nelson C.J., et al. Evaluation and Management of Testosterone Deficiency: AUA Guideline. J Urol. 2018;200:423–432. [PubMed: 29601923]
- 5.
- Forest M.G., Sizonenko P.C., Cathiard A.M., Bertrand J. Hypophyso-gonadal function in humans during the first year of life. 1. Evidence for testicular activity in early infancy. The Journal of clinical investigation. 1974;53:819–828. [PMC free article: PMC333063] [PubMed: 4812441]
- 6.
- Grumbach M.M. A window of opportunity: the diagnosis of gonadotropin deficiency in the male infant. The Journal of clinical endocrinology and metabolism. 2005;90:3122–3127. [PubMed: 15728198]
- 7.
- Boyar R.M., Rosenfeld R.S., Kapen S., Finkelstein J.W., Roffwarg H.P., Weitzman E.D., Hellman L. Human puberty. Simultaneous augmented secretion of luteinizing hormone and testosterone during sleep. The Journal of clinical investigation. 1974;54:609–618. [PMC free article: PMC301594] [PubMed: 4852310]
- 8.
- Kulle A.E., Riepe F.G., Melchior D., Hiort O., Holterhus P.M. A novel ultrapressure liquid chromatography tandem mass spectrometry method for the simultaneous determination of androstenedione, testosterone, and dihydrotestosterone in pediatric blood samples: age- and sex-specific reference data. The Journal of clinical endocrinology and metabolism. 2010;95:2399–2409. [PubMed: 20200336]
- 9.
- Plant T.M. The neurobiological mechanism underlying hypothalamic GnRH pulse generation: the role of kisspeptin neurons in the arcuate nucleus. F1000Res. 2019:8. [PMC free article: PMC6600864] [PubMed: 31297186]
- 10.
- Veldhuis J.D., King J.C., Urban R.J., Rogol A.D., Evans W.S., Kolp L.A., Johnson M.L. Operating characteristics of the male hypothalamo-pituitary-gonadal axis: pulsatile release of testosterone and follicle-stimulating hormone and their temporal coupling with luteinizing hormone. The Journal of clinical endocrinology and metabolism. 1987;65:929–941. [PubMed: 3117834]
- 11.
- Winters S.J., Troen P. Testosterone and estradiol are co-secreted episodically by the human testis. The Journal of clinical investigation. 1986;78:870–873. [PMC free article: PMC423704] [PubMed: 3760188]
- 12.
- Spratt D.I., O'Dea L.S., Schoenfeld D., Butler J., Rao P.N., Crowley W.F. Jr. Neuroendocrine-gonadal axis in men: frequent sampling of LH, FSH, and testosterone. The American journal of physiology. 1988;254:E658–666. [PubMed: 3129947]
- 13.
- Brambilla D.J., Matsumoto A.M., Araujo A.B., McKinlay J.B. The effect of diurnal variation on clinical measurement of serum testosterone and other sex hormone levels in men. The Journal of clinical endocrinology and metabolism. 2009;94:907–913. [PMC free article: PMC2681273] [PubMed: 19088162]
- 14.
- Winters S.J. Diurnal rhythm of testosterone and luteinizing hormone in hypogonadal men. Journal of andrology. 1991;12:185–190. [PubMed: 1917682]
- 15.
- Andersson A.M., Carlsen E., Petersen J.H., Skakkebaek N.E. Variation in levels of serum inhibin B, testosterone, estradiol, luteinizing hormone, follicle-stimulating hormone, and sex hormone-binding globulin in monthly samples from healthy men during a 17-month period: possible effects of seasons. The Journal of clinical endocrinology and metabolism. 2003;88:932–937. [PubMed: 12574235]
- 16.
- Caronia LM, Dwyer AA, Hayden D, Amati F, Pitteloud N, Hayes FJ. Abrupt decrease in serum testosterone levels after an oral glucose load in men: implications for screening for hypogonadism. Clin Endocrinol (Oxf). 2013;78(2):291–6. [PubMed: 22804876]
- 17.
- Vermeulen A., Verdonck G. Representativeness of a single point plasma testosterone level for the long term hormonal milieu in men. The Journal of clinical endocrinology and metabolism. 1992;74:939–942. [PubMed: 1548361]
- 18.
- Wu F.C., Tajar A., Beynon J.M., Pye S.R., Silman A.J., Finn J.D., O'Neill T.W., Bartfai G., Casanueva F.F., Forti G., et al. Identification of late-onset hypogonadism in middle-aged and elderly men. The New England journal of medicine. 2010;363:123–135. [PubMed: 20554979]
- 19.
- Araujo A.B., Esche G.R., Kupelian V., O'Donnell A.B., Travison T.G., Williams R.E., Clark R.V., McKinlay J.B. Prevalence of symptomatic androgen deficiency in men. The Journal of clinical endocrinology and metabolism. 2007;92:4241–4247. [PubMed: 17698901]
- 20.
- Antonio L., Wu F.C., O'Neill T.W., Pye S.R., Ahern T.B., Laurent M.R., Huhtaniemi I.T., Lean M.E., Keevil B.G., Rastrelli G., et al. Low Free Testosterone Is Associated with Hypogonadal Signs and Symptoms in Men with Normal Total Testosterone. The Journal of clinical endocrinology and metabolism. 2016;101:2647–2657. [PubMed: 26909800]
- 21.
- Vesper H.W., Bhasin S., Wang C., Tai S.S., Dodge L.A., Singh R.J., Nelson J., Ohorodnik S., Clarke N.J., Salameh W.A., et al. Interlaboratory comparison study of serum total testosterone (corrected) measurements performed by mass spectrometry methods. Steroids. 2009;74:498–503. [PubMed: 19428438]
- 22.
- Salameh W.A., Redor-Goldman M.M., Clarke N.J., Reitz R.E., Caulfield M.P. Validation of a total testosterone assay using high-turbulence liquid chromatography tandem mass spectrometry: total and free testosterone reference ranges. Steroids. 2010;75:169–175. [PubMed: 19925815]
- 23.
- Cawood M.L., Field H.P., Ford C.G., Gillingwater S., Kicman A., Cowan D., Barth J.H. Testosterone measurement by isotope-dilution liquid chromatography-tandem mass spectrometry: validation of a method for routine clinical practice. Clinical chemistry. 2005;51:1472–1479. [PubMed: 15961553]
- 24.
- Kushnir M.M., Rockwood A.L., Roberts W.L., Pattison E.G., Bunker A.M., Fitzgerald R.L., Meikle A.W. Performance characteristics of a novel tandem mass spectrometry assay for serum testosterone. Clinical chemistry. 2006;52:120–128. [PubMed: 16299050]
- 25.
- Sartorius G., Spasevska S., Idan A., Turner L., Forbes E., Zamojska A., Allan C.A., Ly L.P., Conway A.J., McLachlan R.I., et al. Serum testosterone, dihydrotestosterone and estradiol concentrations in older men self-reporting very good health: the healthy man study. Clinical endocrinology. 2012;77:755–763. [PubMed: 22563890]
- 26.
- Harman S.M., Metter E.J., Tobin J.D., Pearson J., Blackman M.R. Longitudinal effects of aging on serum total and free testosterone levels in healthy men. Baltimore Longitudinal Study of Aging. The Journal of clinical endocrinology and metabolism. 2001;86:724–731. and Baltimore Longitudinal Study of, A. [PubMed: 11158037]
- 27.
- Travison T.G., Araujo A.B., Kupelian V., O'Donnell A.B., McKinlay J.B. The relative contributions of aging, health, and lifestyle factors to serum testosterone decline in men. The Journal of clinical endocrinology and metabolism. 2007;92:549–555. [PubMed: 17148559]
- 28.
- Richard A., Rohrmann S., Zhang L., Eichholzer M., Basaria S., Selvin E., Dobs A.S., Kanarek N., Menke A., Nelson W.G., et al. Racial variation in sex steroid hormone concentration in black and white men: a meta-analysis. Andrology. 2014;2:428–435. [PMC free article: PMC4327897] [PubMed: 24648111]
- 29.
- Bhasin S., Pencina M., Jasuja G.K., Travison T.G., Coviello A., Orwoll E., Wang P.Y., Nielson C., Wu F., Tajar A., et al. Reference ranges for testosterone in men generated using liquid chromatography tandem mass spectrometry in a community-based sample of healthy nonobese young men in the Framingham Heart Study and applied to three geographically distinct cohorts. The Journal of clinical endocrinology and metabolism. 2011;96:2430–2439. [PMC free article: PMC3146796] [PubMed: 21697255]
- 30.
- Elmlinger M.W., Kuhnel W., Wormstall H., Doller P.C. Reference intervals for testosterone, androstenedione and SHBG levels in healthy females and males from birth until old age. Clin Lab. 2005;51:625–632. [PubMed: 16329620]
- 31.
- Mazur A. The age-testosterone relationship in black, white, and Mexican-American men, and reasons for ethnic differences. Aging Male. 2009;12:66–76. [PubMed: 19639516]
- 32.
- Wu T.S., Hammond G.L. Naturally occurring mutants inform SHBG structure and function. Mol Endocrinol. 2014;28:1026–1038. [PMC free article: PMC5414826] [PubMed: 24892637]
- 33.
- Winters S.J., Kelley D.E., Goodpaster B. The analog free testosterone assay: are the results in men clinically useful? Clinical chemistry. 1998;44:2178–2182. [PubMed: 9761253]
- 34.
- Albanese C., Christin-Maitre S., Sluss P.M., Crowley W.F., Jameson J.L. Development of a bioassay for FSH using a recombinant human FSH receptor and a cAMP responsive luciferase reporter gene. Molecular and cellular endocrinology. 1994;101:211–219. [PubMed: 9397955]
- 35.
- Brand J.S., van der Tweel I., Grobbee D.E., Emmelot-Vonk M.H., van der Schouw Y.T. Testosterone, sex hormone-binding globulin and the metabolic syndrome: a systematic review and meta-analysis of observational studies. Int J Epidemiol. 2011;40:189–207. [PubMed: 20870782]
- 36.
- Laaksonen D.E., Niskanen L., Punnonen K., Nyyssonen K., Tuomainen T.P., Valkonen V.P., Salonen R., Salonen J.T. Testosterone and sex hormone-binding globulin predict the metabolic syndrome and diabetes in middle-aged men. Diabetes care. 2004;27:1036–1041. [PubMed: 15111517]
- 37.
- Hammoud A., Gibson M., Hunt S.C., Adams T.D., Carrell D.T., Kolotkin R.L., Meikle A.W. Effect of Roux-en-Y gastric bypass surgery on the sex steroids and quality of life in obese men. The Journal of clinical endocrinology and metabolism. 2009;94:1329–1332. [PMC free article: PMC2682482] [PubMed: 19174499]
- 38.
- Pasquali R., Macor C., Vicennati V., Novo F. Effects of acute hyperinsulinemia on testosterone serum concentrations in adult obese and normal-weight men. Metabolism: clinical and experimental. 1997;46:526–529. De lasio, R., Mesini, P., Boschi, S., Casimirri, F., and Vettor, R. [PubMed: 9160819]
- 39.
- Xie X., Liao H., Dang H., Pang W., Guan Y., Wang X., Shyy J.Y., Zhu Y., Sladek F.M. Down-regulation of hepatic HNF4alpha gene expression during hyperinsulinemia via SREBPs. Mol Endocrinol. 2009;23:434–443. [PMC free article: PMC2667705] [PubMed: 19179483]
- 40.
- Simo R., Saez-Lopez C., Barbosa-Desongles A., Hernandez C., Selva D.M. Novel insights in SHBG regulation and clinical implications. Trends Endocrinol Metab. 2015;26:376–383. [PubMed: 26044465]
- 41.
- Winters S.J., Gogineni J., Karegar M., Scoggins C., Wunderlich C.A., Baumgartner R., Ghooray D.T. Sex hormone-binding globulin gene expression and insulin resistance. The Journal of clinical endocrinology and metabolism. 2014;99:E2780–2788. [PubMed: 25226295]
- 42.
- Goldman A.L., Bhasin S., Wu F.C.W., Krishna M., Matsumoto A.M., Jasuja R. A Reappraisal of Testosterone's Binding in Circulation: Physiological and Clinical Implications. Endocr Rev. 2017;38:302–324. [PMC free article: PMC6287254] [PubMed: 28673039]
- 43.
- Pardridge W.M. Serum bioavailability of sex steroid hormones. Clinics in endocrinology and metabolism. 1986;15:259–278. [PubMed: 3521955]
- 44.
- Keevil B.G., Adaway J. Assessment of free testosterone concentration. The Journal of steroid biochemistry and molecular biology. 2019;190:207–211. [PubMed: 30970279]
- 45.
- Chen Y., Yazdanpanah M., Wang X.Y., Hoffman B.R., Diamandis E.P., Wong P.Y. Direct measurement of serum free testosterone by ultrafiltration followed by liquid chromatography tandem mass spectrometry. Clinical biochemistry. 2010;43:490–496. [PubMed: 20026023]
- 46.
- Ly L.P., Sartorius G., Hull L., Leung A., Swerdloff R.S., Wang C., Handelsman D.J. Accuracy of calculated free testosterone formulae in men. Clinical endocrinology. 2010;73:382–388. [PubMed: 20346001]
- 47.
- Vermeulen A., Verdonck L., Kaufman J.M. A critical evaluation of simple methods for the estimation of free testosterone in serum. The Journal of clinical endocrinology and metabolism. 1999;84:3666–3672. [PubMed: 10523012]
- 48.
- Watts E.L., Appleby P.N., Albanes D., Black A., Chan J.M., Chen C., Cirillo P.M., Cohn B.A., Cook M.B., Donovan J.L., et al. Circulating sex hormones in relation to anthropometric, sociodemographic and behavioural factors in an international dataset of 12,300 men. PloS one. 2017;12:e0187741. [PMC free article: PMC5744924] [PubMed: 29281666]
- 49.
- Damassa D.A., Lin T.M., Sonnenschein C., Soto A.M. Biological effects of sex hormone-binding globulin on androgen-induced proliferation and androgen metabolism in LNCaP prostate cells. Endocrinology. 1991;129:75–84. [PubMed: 2055206]
- 50.
- Kapoor P., Luttrell B.M., Williams D. The free androgen index is not valid for adult males. The Journal of steroid biochemistry and molecular biology. 1993;45:325–326. [PubMed: 8499341]
- 51.
- Fritz K.S., McKean A.J., Nelson J.C., Wilcox R.B. Analog-based free testosterone test results linked to total testosterone concentrations, not free testosterone concentrations. Clinical chemistry. 2008;54:512–516. [PubMed: 18171714]
- 52.
- Need E.F., O'Loughlin P.D., Armstrong D.T., Haren M.T., Martin S.A., Tilley W.D., Florey Adelaide Male Aging S., Wittert G.A., Buchanan G. Serum testosterone bioassay evaluation in a large male cohort. Clinical endocrinology. 2010;72:87–98. [PubMed: 19508600]
- 53.
- van der Veen A., van Faassen M., de Jong W.H.A., van Beek A.P., Dijck-Brouwer D.A.J., Kema I.P. Development and validation of a LC-MS/MS method for the establishment of reference intervals and biological variation for five plasma steroid hormones. Clinical biochemistry. 2019;68:15–23. [PubMed: 30922617]
- 54.
- Maimoun L., Philibert P., Cammas B., Audran F., Bouchard P., Fenichel P., Cartigny M., Pienkowski C., Polak M., Skordis N., et al. Phenotypical, biological, and molecular heterogeneity of 5alpha-reductase deficiency: an extensive international experience of 55 patients. The Journal of clinical endocrinology and metabolism. 2011;96:296–307. [PubMed: 21147889]
- 55.
- Chan A.O., But B.W., Lee C.Y., Lam Y.Y., Ng K.L., Tung J.Y., Kwan E.Y., Chan Y.K., Tsui T.K., Lam A.L., et al. Diagnosis of 5alpha-reductase 2 deficiency: is measurement of dihydrotestosterone essential? Clinical chemistry. 2013;59:798–806. [PubMed: 23513070]
- 56.
- Thirumalai A., Cooper L.A., Rubinow K.B., Amory J.K., Lin D.W., Wright J.L., Marck B.T., Matsumoto A.M., Page S.T. Stable Intraprostatic Dihydrotestosterone in Healthy Medically Castrate Men Treated With Exogenous Testosterone. The Journal of clinical endocrinology and metabolism. 2016;101:2937–2944. [PMC free article: PMC4929843] [PubMed: 27172434]
- 57.
- Russell N., Grossmann M. MECHANISMS IN ENDOCRINOLOGY: Estradiol as a male hormone. Eur J Endocrinol. 2019;181:R23–R43. [PubMed: 31096185]
- 58.
- Finkelstein J.S., Lee H., Burnett-Bowie S.A., Pallais J.C., Yu E.W., Borges L.F., Jones B.F., Barry C.V., Wulczyn K.E., Thomas B.J., et al. Gonadal steroids and body composition, strength, and sexual function in men. The New England journal of medicine. 2013;369:1011–1022. [PMC free article: PMC4142768] [PubMed: 24024838]
- 59.
- Narula H.S., Carlson H.E. Gynaecomastia--pathophysiology, diagnosis and treatment. Nature reviews. Endocrinology. 2014;10:684–698. [PubMed: 25112235]
- 60.
- Gandhi G.Y., Basu R., Dispenzieri A., Basu A., Montori V.M., Brennan M.D. Endocrinopathy in POEMS syndrome: the Mayo Clinic experience. Mayo Clinic proceedings. 2007;82:836–842. [PubMed: 17605964]
- 61.
- Shozu M., Fukami M., Ogata T. Understanding the pathological manifestations of aromatase excess syndrome: lessons for clinical diagnosis. Expert review of endocrinology & metabolism. 2014;9:397–409. [PMC free article: PMC4162655] [PubMed: 25264451]
- 62.
- Hess R.A., Cooke P.S. Estrogen in the male: a historical perspective. Biology of reproduction. 2018;99:27–44. [PMC free article: PMC6044326] [PubMed: 29438493]
- 63.
- Wu A., Shi Z., Martin S., Vincent A., Heilbronn L., Wittert G. Age-related changes in estradiol and longitudinal associations with fat mass in men. PloS one. 2018;13:e0201912. [PMC free article: PMC6072119] [PubMed: 30071117]
- 64.
- Dunn J.F., Nisula B.C., Rodbard D. Transport of steroid hormones: binding of 21 endogenous steroids to both testosterone-binding globulin and corticosteroid-binding globulin in human plasma. The Journal of clinical endocrinology and metabolism. 1981;53:58–68. [PubMed: 7195404]
- 65.
- Rosner W. Free estradiol and sex hormone-binding globulin. Steroids. 2015;99:113–116. [PubMed: 25453337]
- 66.
- Khosla S. Estrogen and bone: insights from estrogen-resistant, aromatase-deficient, and normal men. Bone. 2008;43:414–417. [PMC free article: PMC2553890] [PubMed: 18567553]
- 67.
- Laouali N., Brailly-Tabard S., Helmer C., Ancelin M.L., Tzourio C., Elbaz A., Guiochon-Mantel A., Canonico M. Oestradiol level, oestrogen receptors, and mortality in elderly men: The three-city cohort study. Clinical endocrinology. 2018;89:514–525. [PubMed: 29935032]
- 68.
- Chadid S., Barber J.R., Rohrmann S., Nelson W.G., Yager J.D., Kanarek N.F., Bradwin G., Dobs A.S., McGlynn K.A., Platz E.A. Age-Specific Serum Total and Free Estradiol Concentrations in Healthy Men in US Nationally Representative Samples. J Endocr Soc. 2019;3:1825–1836. [PMC free article: PMC6749840] [PubMed: 31555753]
- 69.
- Resende E.A., Lara B.H., Reis J.D., Ferreira B.P., Pereira G.A., Borges M.F. Assessment of basal and gonadotropin-releasing hormone-stimulated gonadotropins by immunochemiluminometric and immunofluorometric assays in normal children. The Journal of clinical endocrinology and metabolism. 2007;92:1424–1429. [PubMed: 17284632]
- 70.
- Goji K., Tanikaze S. Spontaneous gonadotropin and testosterone concentration profiles in prepubertal and pubertal boys: temporal relationship between luteinizing hormone and testosterone. Pediatric research. 1993;34:229–236. [PubMed: 8233730]
- 71.
- Ventimiglia E., Ippolito S., Capogrosso P., Pederzoli F., Cazzaniga W., Boeri L., Cavarretta I., Alfano M., Vigano P., Montorsi F., et al. Primary, secondary and compensated hypogonadism: a novel risk stratification for infertile men. Andrology. 2017;5:505–510. [PubMed: 28409903]
- 72.
- Pacenza N., Pasqualini T., Gottlieb S., Knoblovits P., Costanzo P.R., Stewart Usher J., Rey R.A., Martinez M.P., Aszpis S. Clinical Presentation of Klinefelter's Syndrome: Differences According to Age. International journal of endocrinology. 2012;2012:324835. [PMC free article: PMC3265068] [PubMed: 22291701]
- 73.
- Kaufman J.M., Lapauw B., Mahmoud A. Aging and the Male Reproductive System. Endocr Rev. 2019;40:906–972. T'Sjoen, G., and Huhtaniemi, I.T. [PubMed: 30888401]
- 74.
- Awouters M., Vanderschueren D., Antonio L. Aromatase inhibitors and selective estrogen receptor modulators: Unconventional therapies for functional hypogonadism? Andrology. 2019 [PubMed: 31696669]
- 75.
- Bulun S.E. Aromatase and estrogen receptor alpha deficiency. Fertility and sterility. 2014;101:323–329. [PMC free article: PMC3939057] [PubMed: 24485503]
- 76.
- Arnhold I.J., Melo K., Costa E.M., Danilovic D., Inacio M., Domenice S., Mendonca B.B. 46,XY disorders of sex development (46,XY DSD) due to androgen receptor defects: androgen insensitivity syndrome. Adv Exp Med Biol. 2011;707:59–61. [PubMed: 21691956]
- 77.
- Verhelst J., Denis L., Van Vliet P., Van Poppel H., Braeckman J., Van Cangh P., Mattelaer J., D'Hulster D., Mahler C. Endocrine profiles during administration of the new non-steroidal anti-androgen Casodex in prostate cancer. Clinical endocrinology. 1994;41:525–530. [PubMed: 7525125]
- 78.
- de Kretser D.M., Burger H.G., Hudson B. The relationship between germinal cells and serum FSH levels in males with infertility. The Journal of clinical endocrinology and metabolism. 1974;38:787–793. [PubMed: 4823921]
- 79.
- Sikaris K., McLachlan R.I., Kazlauskas R., de Kretser D., Holden C.A., Handelsman D.J. Reproductive hormone reference intervals for healthy fertile young men: evaluation of automated platform assays. The Journal of clinical endocrinology and metabolism. 2005;90:5928–5936. [PubMed: 16118337]
- 80.
- Ntali G., Capatina C., Grossman A., Karavitaki N. Clinical review: Functioning gonadotroph adenomas. The Journal of clinical endocrinology and metabolism. 2014;99:4423–4433. [PubMed: 25166722]
- 81.
- Heseltine D., White M.C., Kendall-Taylor P., De Kretser D.M., Kelly W. Testicular enlargement and elevated serum inhibin concentrations occur in patients with pituitary macroadenomas secreting follicle stimulating hormone. Clinical endocrinology. 1989;31:411–423. [PubMed: 2627747]
- 82.
- Chamoun, R., Layfield, L., and Couldwell, W.T. (2013). Gonadotroph adenoma with secondary hypersecretion of testosterone. World Neurosurg 80, 900 e907-911. [PubMed: 23201183]
- 83.
- Thakkar A., Kannan S., Hamrahian A., Prayson R.A., Weil R.J., Faiman C. Testicular "hyperstimulation" syndrome: a case of functional gonadotropinoma. Case reports in endocrinology. 2014;2014:194716. [PMC free article: PMC3926319] [PubMed: 24592337]
- 84.
- Tajar A., Forti G., O'Neill T.W., Lee D.M., Silman A.J., Finn J.D., Bartfai G., Boonen S., Casanueva F.F., Giwercman A., et al. Characteristics of secondary, primary, and compensated hypogonadism in aging men: evidence from the European Male Ageing Study. The Journal of clinical endocrinology and metabolism. 2010;95:1810–1818. [PubMed: 20173018]
- 85.
- Young J., Xu C., Papadakis G.E., Acierno J.S., Maione L., Hietamaki J., Raivio T., Pitteloud N. Clinical Management of Congenital Hypogonadotropic Hypogonadism. Endocr Rev. 2019;40:669–710. [PubMed: 30698671]
- 86.
- Berger K., Souza H., Brito V.N., d'Alva C.B., Mendonca B.B., Latronico A.C. Clinical and hormonal features of selective follicle-stimulating hormone (FSH) deficiency due to FSH beta-subunit gene mutations in both sexes. Fertility and sterility. 2005;83:466–470. [PubMed: 15705395]
- 87.
- Zheng J., Mao J., Cui M., Liu Z., Wang X., Xiong S., Nie M., Wu X. Novel FSHbeta mutation in a male patient with isolated FSH deficiency and infertility. European journal of medical genetics. 2017;60:335–339. [PubMed: 28392474]
- 88.
- Lamminen T., Huhtaniemi I. A common genetic variant of luteinizing hormone; relation to normal and aberrant pituitary-gonadal function. European journal of pharmacology. 2001;414:1–7. [PubMed: 11230989]
- 89.
- Winters S.J., Moore J.P. Jr, Clark B.J. Leydig cell insufficiency in hypospermatogenesis: a paracrine effect of activin-inhibin signaling? Andrology. 2018;6:262–271. [PubMed: 29409132]
- 90.
- Basciani S., Watanabe M., Mariani S., Passeri M., Persichetti A., Fiore D., Scotto d'Abusco A., Caprio M., Lenzi A., Fabbri A., et al. Hypogonadism in a patient with two novel mutations of the luteinizing hormone beta-subunit gene expressed in a compound heterozygous form. The Journal of clinical endocrinology and metabolism. 2012;97:3031–3038. [PubMed: 22723313]
- 91.
- Yang X., Ochin H., Shu L., Liu J., Shen J., Liu J., Lin C., Cui Y. Homozygous nonsense mutation Trp28X in the LHB gene causes male hypogonadism. Journal of assisted reproduction and genetics. 2018;35:913–919. [PMC free article: PMC5984881] [PubMed: 29476300]
- 92.
- Weiss J., Axelrod L., Whitcomb R.W., Harris P.E., Crowley W.F., Jameson J.L. Hypogonadism caused by a single amino acid substitution in the beta subunit of luteinizing hormone. The New England journal of medicine. 1992;326:179–183. [PubMed: 1727547]
- 93.
- Campo S., Andreone L., Ambao V., Urrutia M., Calandra R.S., Rulli S.B. Hormonal Regulation of Follicle-Stimulating Hormone Glycosylation in Males. Frontiers in endocrinology. 2019;10:17. [PMC free article: PMC6361742] [PubMed: 30761084]
- 94.
- Dufau M.L., Pock R., Neubauer A., Catt K.J. In vitro bioassay of LH in human serum: the rat interstitial cell testosterone (RICT) assay. The Journal of clinical endocrinology and metabolism. 1976;42:958–969. [PubMed: 1270585]
- 95.
- Dahl K.D., Stone M.P. FSH isoforms, radioimmunoassays, bioassays, and their significance. Journal of andrology. 1992;13:11–22. [PubMed: 1551802]
- 96.
- Winters S.J., Troen P. Pulsatile secretion of immunoreactive alpha-subunit in man. The Journal of clinical endocrinology and metabolism. 1985;60:344–348. [PubMed: 2578134]
- 97.
- Styne D.M., Kaplan S.L., Grumbach M.M. Plasma glycoprotein hormone alpha-subunit in the neonate and in prepubertal and pubertal children: effects of luteinizing hormone-releasing hormone. The Journal of clinical endocrinology and metabolism. 1980;50:450–455. [PubMed: 6987251]
- 98.
- Kourides I.A., Weintraub B.D., Ridgway E.C., Maloof F. Pituitary secretion of free alpha and beta subunit of human thyrotropin in patients with thyroid disorders. The Journal of clinical endocrinology and metabolism. 1975;40:872–885. [PubMed: 1127093]
- 99.
- Mainieri A.S., Elnecave R.H. Usefulness of the free alpha-subunit to diagnose hypogonadotropic hypogonadism. Clinical endocrinology. 2003;59:307–313. [PubMed: 12919153]
- 100.
- Oppenheim D.S., Kana A.R., Sangha J.S., Klibanski A. Prevalence of alpha-subunit hypersecretion in patients with pituitary tumors: clinically nonfunctioning and somatotroph adenomas. The Journal of clinical endocrinology and metabolism. 1990;70:859–864. [PubMed: 1690748]
- 101.
- Medri G., Carella C., Padmanabhan V., Rossi C.M., Amato G., De Santo N.G., Beitins I.Z., Beck-Peccoz P. Pituitary glycoprotein hormones in chronic renal failure: evidence for an uncontrolled alpha-subunit release. Journal of endocrinological investigation. 1993;16:169–174. [PubMed: 7685785]
- 102.
- Bay K., Main K.M., Toppari J., Skakkebaek N.E. Testicular descent: INSL3, testosterone, genes and the intrauterine milieu. Nat Rev Urol. 2011;8:187–196. [PubMed: 21403659]
- 103.
- Johansen M.L., Anand-Ivell R., Mouritsen A., Hagen C.P., Mieritz M.G., Soeborg T., Johannsen T.H., Main K.M., Andersson A.M., Ivell R., et al. Serum levels of insulin-like factor 3, anti-Mullerian hormone, inhibin B, and testosterone during pubertal transition in healthy boys: a longitudinal pilot study. Reproduction (Cambridge, England). 2014;147:529–535. [PubMed: 24459206]
- 104.
- Ferlin A., Arredi B., Zuccarello D., Garolla A., Selice R., Foresta C. Paracrine and endocrine roles of insulin-like factor 3. Journal of endocrinological investigation. 2006;29:657–664. [PubMed: 16957417]
- 105.
- Trabado S., Maione L., Bry-Gauillard H., Affres H., Salenave S., Sarfati J., Bouvattier C., Delemer B., Chanson P., Le Bouc Y., et al. Insulin-like peptide 3 (INSL3) in men with congenital hypogonadotropic hypogonadism/Kallmann syndrome and effects of different modalities of hormonal treatment: a single-center study of 281 patients. The Journal of clinical endocrinology and metabolism. 2014;99:E268–275. [PubMed: 24243640]
- 106.
- Makanji Y., Zhu J., Mishra R., Holmquist C., Wong W.P., Schwartz N.B., Mayo K.E., Woodruff T.K. Inhibin at 90: from discovery to clinical application, a historical review. Endocr Rev. 2014;35:747–794. [PMC free article: PMC4167436] [PubMed: 25051334]
- 107.
- Gray P.C., Bilezikjian L.M., Vale W. Antagonism of activin by inhibin and inhibin receptors: a functional role for betaglycan. Molecular and cellular endocrinology. 2002;188:254–260. [PubMed: 11911962]
- 108.
- Ludlow H., Muttukrishna S., Hyvonen M., Groome N.P. Development of a new antibody to the human inhibin/activin betaB subunit and its application to improved inhibin B ELISAs. J Immunol Methods. 2008;329:102–111. [PubMed: 17991484]
- 109.
- Andersson A.M., Toppari J., Haavisto A.M., Petersen J.H., Simell T., Simell O., Skakkebaek N.E. Longitudinal reproductive hormone profiles in infants: peak of inhibin B levels in infant boys exceeds levels in adult men. The Journal of clinical endocrinology and metabolism. 1998;83:675–681. [PubMed: 9467591]
- 110.
- Mahmoud A.M., Goemaere S., De Bacquer D., Comhaire F.H., Kaufman J.M. Serum inhibin B levels in community-dwelling elderly men. Clinical endocrinology. 2000;53:141–147. [PubMed: 10931093]
- 111.
- Anawalt B.D., Bebb R.A., Matsumoto A.M., Groome N.P., Illingworth P.J., McNeilly A.S., Bremner W.J. Serum inhibin B levels reflect Sertoli cell function in normal men and men with testicular dysfunction. The Journal of clinical endocrinology and metabolism. 1996;81:3341–3345. [PubMed: 8784094]
- 112.
- Abdelrahaman E., Raghavan S., Baker L., Weinrich M., Winters S.J. Racial difference in circulating sex hormone-binding globulin levels in prepubertal boys. Metabolism: clinical and experimental. 2005;54:91–96. [PubMed: 15562386]
- 113.
- Barbotin A.L., Ballot C., Sigala J., Ramdane N., Duhamel A., Marcelli F., Rigot J.M., Dewailly D., Pigny P., Mitchell V. The serum inhibin B concentration and reference ranges in normozoospermia. Eur J Endocrinol. 2015;172:669–676. [PubMed: 25740852]
- 114.
- Andersson A.M. Inhibin B in the assessment of seminiferous tubular function. Baillieres Best Pract Res Clin Endocrinol Metab. 2000;14:389–397. [PubMed: 11517906]
- 115.
- Crofton P.M., Evans A.E., Groome N.P., Taylor M.R., Holland C.V., Kelnar C.J. Inhibin B in boys from birth to adulthood: relationship with age, pubertal stage, FSH and testosterone. Clinical endocrinology. 2002;56:215–221. [PubMed: 11874413]
- 116.
- Myers G.M., Lambert-Messerlian G.M., Sigman M. Inhibin B reference data for fertile and infertile men in Northeast America. Fertility and sterility. 2009;92:1920–1923. [PubMed: 19006797]
- 117.
- Pierik F.H., Vreeburg J.T., Stijnen T., De Jong F.H., Weber R.F. Serum inhibin B as a marker of spermatogenesis. The Journal of clinical endocrinology and metabolism. 1998;83:3110–3114. [PubMed: 9745412]
- 118.
- von Eckardstein S., Simoni M., Bergmann M., Weinbauer G.F., Gassner P., Schepers A.G., Nieschlag E. Serum inhibin B in combination with serum follicle-stimulating hormone (FSH) is a more sensitive marker than serum FSH alone for impaired spermatogenesis in men, but cannot predict the presence of sperm in testicular tissue samples. The Journal of clinical endocrinology and metabolism. 1999;84:2496–2501. [PubMed: 10404826]
- 119.
- Christiansen P., Andersson A.M., Skakkebaek N.E. Longitudinal studies of inhibin B levels in boys and young adults with Klinefelter syndrome. The Journal of clinical endocrinology and metabolism. 2003;88:888–891. [PubMed: 12574229]
- 120.
- Wallace E.M., Groome N.P., Riley S.C., Parker A.C., Wu F.C. Effects of chemotherapy-induced testicular damage on inhibin, gonadotropin, and testosterone secretion: a prospective longitudinal study. The Journal of clinical endocrinology and metabolism. 1997;82:3111–3115. [PubMed: 9284754]
- 121.
- Petersen P.M., Andersson A.M., Rorth M., Daugaard G., Skakkebaek N.E. Undetectable inhibin B serum levels in men after testicular irradiation. The Journal of clinical endocrinology and metabolism. 1999;84:213–215. [PubMed: 9920086]
- 122.
- Isaksson S., Eberhard J., Stahl O., Cavallin-Stahl E., Cohn-Cedermark G., Arver S., Lundberg Giwercman Y., Giwercman A. Inhibin B concentration is predictive for long-term azoospermia in men treated for testicular cancer. Andrology. 2014;2:252–258. [PubMed: 24519955]
- 123.
- Martin C.W., Riley S.C., Everington D., Groome N.P., Riemersma R.A., Baird D.T., Anderson R.A. Dose-finding study of oral desogestrel with testosterone pellets for suppression of the pituitary-testicular axis in normal men. Human reproduction (Oxford, England). 2000;15:1515–1524. [PubMed: 10875859]
- 124.
- Mitchell V., Boitrelle F., Pigny P., Robin G., Marchetti C., Marcelli F., Rigot J.M. Seminal plasma levels of anti-Mullerian hormone and inhibin B are not predictive of testicular sperm retrieval in nonobstructive azoospermia: a study of 139 men. Fertility and sterility. 2010;94:2147–2150. [PubMed: 20172518]
- 125.
- Seminara S.B., Boepple P.A., Nachtigall L.B., Pralong F.P., Khoury R.H., Sluss P.M., Lecain A.E., Crowley W.F. Jr. Inhibin B in males with gonadotropin-releasing hormone (GnRH) deficiency: changes in serum concentration after shortterm physiologic GnRH replacement--a clinical research center study. The Journal of clinical endocrinology and metabolism. 1996;81:3692–3696. [PubMed: 8855824]
- 126.
- Coutant R., Biette-Demeneix E., Bouvattier C., Bouhours-Nouet N., Gatelais F., Dufresne S., Rouleau S., Lahlou N. Baseline inhibin B and anti-Mullerian hormone measurements for diagnosis of hypogonadotropic hypogonadism (HH) in boys with delayed puberty. The Journal of clinical endocrinology and metabolism. 2010;95:5225–5232. [PubMed: 20826577]
- 127.
- Binder G., Schweizer R., Blumenstock G., Braun R. Inhibin B plus LH vs GnRH agonist test for distinguishing constitutional delay of growth and puberty from isolated hypogonadotropic hypogonadism in boys. Clinical endocrinology. 2015;82:100–105. [PubMed: 25207430]
- 128.
- Brauner R., Neve M., Allali S., Trivin C., Lottmann H., Bashamboo A., McElreavey K. Clinical, biological and genetic analysis of anorchia in 26 boys. PloS one. 2011;6:e23292. [PMC free article: PMC3154292] [PubMed: 21853106]
- 129.
- Clemente M., Caracseghi F., Gussinyer M., Yeste D., Albisu M., Vazquez E., Ortega A., Carrascosa A. Macroorchidism and panhypopituitarism: two different forms of presentation of FSH-secreting pituitary adenomas in adolescence. Horm Res Paediatr. 2011;75:225–230. [PubMed: 21196695]
- 130.
- Bergada I., Del Toro K., Katz O., Chemes H., Campo S. Serum inhibin B concentration in a prepubertal boy with gynecomastia and Peutz-Jeghers syndrome. J Pediatr Endocrinol Metab. 2000;13:101–103. [PubMed: 10689645]
- 131.
- Coutant R., Lumbroso S., Rey R., Lahlou N., Venara M., Rouleau S., Sultan C., Limal J.M. Macroorchidism due to autonomous hyperfunction of Sertoli cells and G(s)alpha gene mutation: an unusual expression of McCune-Albright syndrome in a prepubertal boy. The Journal of clinical endocrinology and metabolism. 2001;86:1778–1781. [PubMed: 11297617]
- 132.
- Fragoso M.C., Kohek M.B., Martin R.M., Latronico A.C., Lucon A.M., Zerbini M.C., Longui C.A., Mendonca B.B., Domenice S. An inhibin B and estrogen-secreting adrenocortical carcinoma leading to selective FSH suppression. Hormone research. 2007;67:7–11. [PubMed: 16974107]
- 133.
- Xu H.Y., Zhang H.X., Xiao Z., Qiao J., Li R. Regulation of anti-Mullerian hormone (AMH) in males and the associations of serum AMH with the disorders of male fertility. Asian journal of andrology. 2019;21:109–114. [PMC free article: PMC6413543] [PubMed: 30381580]
- 134.
- Josso N., Rey R.A., Picard J.Y. Anti-mullerian hormone: a valuable addition to the toolbox of the pediatric endocrinologist. International journal of endocrinology. 2013;2013:674105. [PMC free article: PMC3870610] [PubMed: 24382961]
- 135.
- Sharpe R.M., McKinnell C., Kivlin C., Fisher J.S. Proliferation and functional maturation of Sertoli cells, and their relevance to disorders of testis function in adulthood. Reproduction (Cambridge, England). 2003;125:769–784. [PubMed: 12773099]
- 136.
- Young J., Rey R., Couzinet B., Chanson P., Josso N., Schaison G. Antimullerian hormone in patients with hypogonadotropic hypogonadism. The Journal of clinical endocrinology and metabolism. 1999;84:2696–2699. [PubMed: 10443662]
- 137.
- Tuttelmann F., Dykstra N., Themmen A.P., Visser J.A., Nieschlag E., Simoni M. Anti-Mullerian hormone in men with normal and reduced sperm concentration and men with maldescended testes. Fertility and sterility. 2009;91:1812–1819. [PubMed: 18423454]
- 138.
- Andersen J.M., Herning H., Aschim E.L., Hjelmesaeth J., Mala T., Hanevik H.I., Bungum M., Haugen T.B., Witczak O. Body Mass Index Is Associated with Impaired Semen Characteristics and Reduced Levels of Anti-Mullerian Hormone across a Wide Weight Range. PloS one. 2015;10:e0130210. [PMC free article: PMC4466334] [PubMed: 26067627]
- 139.
- Aksglaede L., Sorensen K., Boas M., Mouritsen A., Hagen C.P., Jensen R.B., Petersen J.H., Linneberg A., Andersson A.M., Main K.M., et al. Changes in anti-Mullerian hormone (AMH) throughout the life span: a population-based study of 1027 healthy males from birth (cord blood) to the age of 69 years. The Journal of clinical endocrinology and metabolism. 2010;95:5357–5364. [PubMed: 20843948]
- 140.
- Bertelloni S., Russo G., Baroncelli G.I. Human Chorionic Gonadotropin Test: Old Uncertainties, New Perspectives, and Value in 46,XY Disorders of Sex Development. Sexual development : genetics, molecular biology, evolution, endocrinology, embryology, and pathology of sex determination and differentiation. 2018;12:41–49. [PubMed: 29131089]
- 141.
- McEachern R., Houle A.M., Garel L., Van Vliet G. Lost and found testes: the importance of the hCG stimulation test and other testicular markers to confirm a surgical declaration of anorchia. Hormone research. 2004;62:124–128. [PubMed: 15286448]
- 142.
- Wheeler K.M., Sharma D., Kavoussi P.K., Smith R.P., Costabile R. Clomiphene Citrate for the Treatment of Hypogonadism. Sexual medicine reviews. 2019;7:272–276. [PubMed: 30522888]
- 143.
- Glass A.R., Eil C. Ketoconazole-induced reduction in serum 1,25-dihydroxyvitamin D. The Journal of clinical endocrinology and metabolism. 1986;63:766–769. [PubMed: 3755445]
- 144.
- Winters S.J., Troen P. Evidence for a role of endogenous estrogen in the hypothalamic control of gonadotropin secretion in men. The Journal of clinical endocrinology and metabolism. 1985;61:842–845. [PubMed: 2413063]
- 145.
- Hayes F.J., Seminara S.B., Decruz S., Boepple P.A., Crowley W.F. Jr. Aromatase inhibition in the human male reveals a hypothalamic site of estrogen feedback. The Journal of clinical endocrinology and metabolism. 2000;85:3027–3035. [PubMed: 10999781]
- 146.
- Veldhuis J.D., Zwart A., Mulligan T., Iranmanesh A. Muting of androgen negative feedback unveils impoverished gonadotropin-releasing hormone/luteinizing hormone secretory reactivity in healthy older men. The Journal of clinical endocrinology and metabolism. 2001;86:529–535. [PubMed: 11158004]
- 147.
- Ghai K., Cara J.F., Rosenfield R.L. Gonadotropin releasing hormone agonist (nafarelin) test to differentiate gonadotropin deficiency from constitutionally delayed puberty in teen-age boys--a clinical research center study. The Journal of clinical endocrinology and metabolism. 1995;80:2980–2986. [PubMed: 7559884]
- 148.
- Wilson D.A., Hofman P.L., Miles H.L., Unwin K.E., McGrail C.E., Cutfield W.S. Evaluation of the buserelin stimulation test in diagnosing gonadotropin deficiency in males with delayed puberty. J Pediatr. 2006;148:89–94. [PubMed: 16423605]
- 149.
- Segal T.Y., Mehta A., Anazodo A., Hindmarsh P.C., Dattani M.T. Role of gonadotropin-releasing hormone and human chorionic gonadotropin stimulation tests in differentiating patients with hypogonadotropic hypogonadism from those with constitutional delay of growth and puberty. The Journal of clinical endocrinology and metabolism. 2009;94:780–785. [PubMed: 19017752]
- 150.
- Harrington J., Palmert M.R. Clinical review: Distinguishing constitutional delay of growth and puberty from isolated hypogonadotropic hypogonadism: critical appraisal of available diagnostic tests. The Journal of clinical endocrinology and metabolism. 2012;97:3056–3067. [PubMed: 22723321]
- 151.
- Barratt C.L.R., Bjorndahl L., De Jonge C.J., Lamb D.J., Osorio Martini F., McLachlan R., Oates R.D., van der Poel S., St John B., Sigman M., et al. The diagnosis of male infertility: an analysis of the evidence to support the development of global WHO guidance-challenges and future research opportunities. Hum Reprod Update. 2017;23:660–680. [PMC free article: PMC5850791] [PubMed: 28981651]
- 152.
- Organization, W.H. WHO Laboratory Manual for the Examination and Processing of Human Semen, (Cambridge Univeristy Press, New York).
- 153.
- Guzick D.S., Overstreet J.W., Factor-Litvak P., Brazil C.K., Nakajima S.T., Coutifaris C., Carson S.A., Cisneros P., Steinkampf M.P., Hill J.A., et al. Sperm morphology, motility, and concentration in fertile and infertile men. The New England journal of medicine. 2001;345:1388–1393. [PubMed: 11794171]
- 154.
- Yatsenko A.N., Yatsenko S.A., Weedin J.W., Lawrence A.E., Patel A., Peacock S., Matzuk M.M., Lamb D.J., Cheung S.W., Lipshultz L.I. Comprehensive 5-year study of cytogenetic aberrations in 668 infertile men. J Urol. 2010;183:1636–1642. [PMC free article: PMC2969182] [PubMed: 20172548]
- 155.
- Rives N. Y chromosome microdeletions and alterations of spermatogenesis, patient approach and genetic counseling. Ann Endocrinol (Paris). 2014;75:112–114. [PubMed: 24786699]
- 156.
- Zhang F., Li L., Wang L., Yang L., Liang Z., Li J., Jin F., Tian Y. Clinical characteristics and treatment of azoospermia and severe oligospermia patients with Y-chromosome microdeletions. Mol Reprod Dev. 2013;80:908–915. [PubMed: 24002933]
- 157.
- Johnson M., Raheem A., De Luca F., Hallerstrom M., Zainal Y., Poselay S., Mohammadi B., Moubasher A., Johnson T.F., Muneer A., et al. An analysis of the frequency of Y-chromosome microdeletions and the determination of a threshold sperm concentration for genetic testing in infertile men. BJU Int. 2019;123:367–372. [PubMed: 30113756]
- 158.
- Mittal P.K., Little B., Harri P.A., Miller F.H., Alexander L.F., Kalb B., Camacho J.C., Master V., Hartman M., Moreno C.C. Role of Imaging in the Evaluation of Male Infertility. Radiographics. 2017;37:837–854. [PubMed: 28410062]
- 159.
- de Souza D.A.S., Faucz F.R., Pereira-Ferrari L., Sotomaior V.S., Raskin S. Congenital bilateral absence of the vas deferens as an atypical form of cystic fibrosis: reproductive implications and genetic counseling. Andrology. 2018;6:127–135. [PMC free article: PMC5745269] [PubMed: 29216686]
- 160.
- Krausz C, Hoefsloot L, Simoni M, Tüttelmann F., European Academy of Andrology. European Molecular Genetics Quality Network. EAA/EMQN best practice guidelines for molecular diagnosis of Y-chromosomal microdeletions: state-of-the-art 2013. Andrology. 2014 Jan;2(1):5–19. [PMC free article: PMC4065365] [PubMed: 24357628]
- Evaluation of the hypothalamic-pituitary-gonadal axis in eugonadal men with type 2 diabetes mellitus.[Andrology. 2014]Evaluation of the hypothalamic-pituitary-gonadal axis in eugonadal men with type 2 diabetes mellitus.Costanzo PR, Suárez SM, Scaglia HE, Zylbersztein C, Litwak LE, Knoblovits P. Andrology. 2014 Jan; 2(1):117-24. Epub 2013 Nov 26.
- Multiple forms of hypogonadism of central, peripheral or combined origin in males with Prader-Willi syndrome.[Clin Endocrinol (Oxf). 2012]Multiple forms of hypogonadism of central, peripheral or combined origin in males with Prader-Willi syndrome.Radicioni AF, Di Giorgio G, Grugni G, Cuttini M, Losacco V, Anzuini A, Spera S, Marzano C, Lenzi A, Cappa M, et al. Clin Endocrinol (Oxf). 2012 Jan; 76(1):72-7.
- Testicular function in a birth cohort of young men.[Hum Reprod. 2015]Testicular function in a birth cohort of young men.Hart RJ, Doherty DA, McLachlan RI, Walls ML, Keelan JA, Dickinson JE, Skakkebaek NE, Norman RJ, Handelsman DJ. Hum Reprod. 2015 Dec; 30(12):2713-24. Epub 2015 Sep 25.
- Review [Exploration of testicular function].[Endocrinol Nutr. 2009]Review [Exploration of testicular function].Bassas Arnau L. Endocrinol Nutr. 2009 Jan; 56(1):18-31. Epub 2009 Mar 1.
- Review Male hypogonadism: an extended classification based on a developmental, endocrine physiology-based approach.[Andrology. 2013]Review Male hypogonadism: an extended classification based on a developmental, endocrine physiology-based approach.Rey RA, Grinspon RP, Gottlieb S, Pasqualini T, Knoblovits P, Aszpis S, Pacenza N, Stewart Usher J, Bergadá I, Campo SM. Andrology. 2013 Jan; 1(1):3-16. Epub 2012 Oct 9.
- Laboratory Assessment of Testicular Function - EndotextLaboratory Assessment of Testicular Function - Endotext
Your browsing activity is empty.
Activity recording is turned off.
See more...