By agreement with the publisher, this book is accessible by the search feature, but cannot be browsed.
NCBI Bookshelf. A service of the National Library of Medicine, National Institutes of Health.
Janeway CA Jr, Travers P, Walport M, et al. Immunobiology: The Immune System in Health and Disease. 5th edition. New York: Garland Science; 2001.
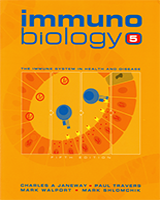
Immunobiology: The Immune System in Health and Disease. 5th edition.
Show detailsImmunodeficiencies occur when one or more components of the immune system is defective. The commonest cause of immune deficiency worldwide is malnutrition; however, in developed countries, most immunodeficiency diseases are inherited, and these are usually seen in the clinic as recurrent or overwhelming infections in very young children. Less commonly, acquired immunodeficiencies with causes other than malnutrition can manifest later in life. Although the pathogenesis of many of these acquired disorders has remained obscure, some are caused by known agents, such as drugs or irradiation that damage lymphocytes, or infection with measles or HIV. By examining which infections accompany a particular inherited or acquired immunodeficiency, we can see which components of the immune system are important in the response to particular infectious agents. The inherited immunodeficiency diseases also reveal how interactions between different cell types contribute to the immune response and to the development of T and B lymphocytes. Finally, these inherited diseases can lead us to the defective gene, often revealing new information about the molecular basis of immune processes and providing the necessary information for diagnosis, for genetic counseling, and eventually for gene therapy.
11-6. A history of repeated infections suggests a diagnosis of immunodeficiency
Patients with immune deficiency are usually detected clinically by a history of recurrent infection. The type of infection is a guide to which part of the immune system is deficient. Recurrent infection by pyogenic bacteria suggests a defect in antibody, complement, or phagocyte function, reflecting the role of these parts of the immune system in host defense against such infections. By contrast, a history of recurrent viral infections is more suggestive of a defect in host defense mediated by T lymphocytes.
To determine the competence of the immune system in patients with possible immunodeficiency, a battery of tests is usually conducted (Fig. 11.8); these focus with increasing precision as the nature of the defect is narrowed down to a single element. The presence of the various cell types in blood is determined by routine hematology, often followed by FACS analysis (see Appendix I, Section A-22) of lymphocyte subsets, and the measurement of serum immunoglobulins. The phagocytic competence of freshly isolated polymorphonuclear leukocytes and monocytes is tested, and the efficiency of the complement system is determined by testing the dilution of serum required for lysis of 50% of antibody-coated red blood cells (this is denoted the CH50).

Figure 11.8
Evaluation of immune competence.
In general, if such tests reveal a defect in one of these broad compartments of immune function, more specialized testing is then needed to determine the precise nature of the defect. Tests of lymphocyte function are often valuable, starting with the ability of polyclonal mitogens to induce T-cell proliferation and B-cell secretion of immunoglobulin in tissue culture (see Appendix I, Section A-31). These tests can eventually pinpoint the cellular defect in immunodeficiency.
11-7. Inherited immunodeficiency diseases are caused by recessive gene defects
Before the advent of highly effective antibiotic therapy, it is likely that most individuals with inherited immune defects died in infancy or early childhood because of their susceptibility to particular classes of pathogen (Fig. 11.9). Such cases would not have been easy to identify, as many normal infants also died of infection. Thus, although many inherited immunodeficiency diseases have now been identified, the first immunodeficiency disease was not described until 1952. Most of the gene defects that cause these inherited immunodeficiencies are recessive and, for this reason, many of the known immunodeficiencies are caused by mutations in genes on the X chromosome. Recessive defects cause disease only when both chromosomes are defective. However, as males have only one X chromosome, all males who inherit an X chromosome carrying a defective gene will manifest disease, whereas female carriers, having two X chromosomes, are perfectly healthy. Immunodeficiency diseases that affect various steps in B- and T-lymphocyte development have been described, as have defects in surface molecules that are important for T- or B-cell function. Defects in phagocytic cells, in complement, in cytokines, in cytokine receptors, and in molecules that mediate effector responses also occur (see Fig. 11.9). Thus, immunodeficiency can be caused by defects in either the adaptive or the innate immune system. Individual examples of these diseases will be described in later sections.

Figure 11.9
Human immunodeficiency syndromes. The specific gene defect, the consequence for the immune system, and the resulting disease susceptibilities are listed for some common and some rare human immunodeficiency syndromes. ADA, adenosine deaminase; PNP, purine (more...)
More recently, the use of gene knockout techniques in mice has allowed the creation of many immunodeficient states that are adding rapidly to our knowledge of the contribution of individual molecules to normal immune function. Nevertheless, human immunodeficiency disease is still the best source of insight into normal pathways of host defense against infectious diseases in humans. For example, a deficiency of antibody, of complement, or of phagocytic function each increases the risk of infection by certain pyogenic bacteria. This shows that the normal pathway of host defense against such bacteria is the binding of antibody followed by fixation of complement, which allows the uptake of opsonized bacteria by phagocytic cells. Breaking any one of the links in this chain of events leading to bacterial killing causes a similar immunodeficient state.
The study of immunodeficiency also teaches us about the redundancy of mechanisms of host defense against infectious disease. The first two humans to be discovered with a hereditary deficiency of complement were healthy immunologists. This teaches us two lessons. The first is that there are multiple protective immune mechanisms against infection; for example, although there is abundant evidence that complement deficiency increases susceptibility to pyogenic infection, not every human with complement deficiency suffers from recurrent infections. The second lesson concerns the phenomenon of ascertainment artifact. When an unusual observation is made in a patient with disease, there is a temptation to seek a causal link. However, no one would suggest that complement deficiency causes a genetic predisposition to becoming an immunologist. Complement deficiency was discovered in immunologists because they used their own blood in their experiments. If a particular measurement is made only in a highly selected group of patients with a particular disease, it is inevitable that the only abnormal results will be discovered in patients with that disease. This is an ascertainment artifact and emphasizes the importance of studying appropriate controls.
11-8. The main effect of low levels of antibody is an inability to clear extracellular bacteria
Pyogenic, or pus-forming, bacteria have polysaccharide capsules that are not directly recognized by the receptors on macrophages and neutrophils that stimulate phagocytosis. They therefore escape immediate elimination by the innate immune response and are successful extracellular pathogens. Normal individuals can clear infections by such bacteria because antibody and complement opsonize the bacteria, making it possible for phagocytes to ingest and destroy them. The principal effect of deficiencies in antibody production is therefore a failure to control this class of bacterial infection. In addition, susceptibility to some viral infections, most notably those caused by enteroviruses, is also increased, because of the importance of antibodies in neutralizing infectious viruses that enter the body through the gut (see Chapter 10).
The first description of an immunodeficiency disease was Ogden C. Bruton's
account, in 1952, of the failure of a male child to produce antibody. As this
defect is inherited in an X-linked fashion and is characterized by the absence
of immunoglobulin in the serum, it was called Bruton's X-linked agammaglobulinemia (XLA) (X-linked Agammaglobulinemia, in
Case Studies in Immunology, see Preface for details).
The absence of antibody can be detected using immunoelectrophoresis (Fig. 11.10). Since then, many more
diseases of antibody production have been described, most of them the
consequence of failures in the development or activation of B lymphocytes.
Infants with these diseases are usually identified as a result of recurrent
infections with pyogenic bacteria such as Streptococcus
pneumoniae.

Figure 11.10
Immunoelectrophoresis reveals the absence of several distinct immunoglobulin isotypes in serum from a patient with X-linked agammaglobulinemia (XLA). Serum samples from a normal control and from a patient with recurrent bacterial infection caused by the (more...)
The defective gene in XLA is now known to encode a protein tyrosine kinase called Btk (Bruton's tyrosine kinase) which is a member of the recently described family called Tec kinases (see Section 6-10). This protein is expressed in neutrophils as well as in B cells, although only B cells are defective in these patients, in whom B-cell maturation halts at the pre-B-cell stage. Thus it is likely that Btk is required to couple the pre-B-cell receptor (which consists of heavy chains, surrogate light chains, and Igα and Igβ) to nuclear events that lead to pre-B-cell growth and differentiation (see Section 7-9). In patients with Btk deficiencies, some B cells mature despite the defect in the signaling kinase, suggesting that signals transmitted by these kinases are not absolutely required.
As the gene responsible for XLA is found on the X chromosome, it is possible to identify female carriers by analyzing X-chromosome inactivation in their B cells. During development, female cells randomly inactivate one of their two X chromosomes. Because the product of a normal btk gene is required for normal B-lymphocyte development, only cells in which the normal allele of btk is active can develop into mature B cells. Thus, in female carriers of mutant btk genes, all B cells have the normal X chromosome as the active X. By contrast, the active X chromosomes in the T cells and macrophages of carriers are an equal mixture of the normal and btk mutant X chromosomes. This fact allowed female carriers of XLA to be identified even before the nature of btk was known. Nonrandom X inactivation only in B cells also demonstrates conclusively that the btk gene is required for normal B-cell development but not for the development of other cell types, and that Btk must act within B cells rather than on stromal cells or other cells required for B-cell development (Fig. 11.11).

Figure 11.11
The product of the btk gene is important for B-cell development. In X-linked agamma-globulinemia (XLA), a protein tyrosine kinase of the Tec family called Btk, encoded on the X chromosome, is defective. In normal individuals, B-cell development proceeds through (more...)
The commonest humoral immune defect is the transient deficiency in immunoglobulin production that occurs in the first 6–12 months of life. The newborn infant has initial antibody levels comparable to those of the mother, because of the transplacental transport of maternal IgG (see Chapter 9). As the transferred IgG is catabolized, antibody levels gradually decrease until the infant begins to produce useful amounts of its own IgG at about 6 months of age (Fig. 11.12). Thus, IgG levels are quite low between the ages of 3 months and 1 year and active IgG antibody responses are poor. In some infants this can lead to a period of heightened susceptibility to infection. This is especially true for premature babies, who begin with lower levels of maternal IgG and also reach immune competence later after birth.

Figure 11.12
Immunoglobulin levels in newborn infants fall to low levels around 6 months of age. Newborn babies have high levels of IgG, transported across the placenta from the mother during gestation. After birth, the production of IgM starts almost immediately; (more...)
The most common inherited form of immunoglobulin deficiency is selective IgA deficiency, which is seen in about 1 person in 800. Although no obvious disease susceptibility is associated with selective IgA defects, they are commoner in people with chronic lung disease than in the general population. Lack of IgA might thus result in a predisposition to lung infections with various pathogens and is consistent with the role of IgA in defense at the body's surfaces. The genetic basis of this defect is unknown but some data suggest that a gene of unidentified function mapping in the class III region of the MHC could be involved. A related syndrome called common variable immunodeficiency, in which there is usually a deficiency in both IgG and IgA, also maps to the MHC region.
People with pure B-cell defects resist many pathogens successfully. However, effective host defense against a subset of extracellular pyogenic bacteria, including staphylococci and streptococci, requires opsonization of these bacteria with specific antibody. These infections can be suppressed with antibiotics and periodic infusions of human immunoglobulin collected from a large pool of donors. As there are antibodies against many pathogens in this pooled immunoglobulin, it serves as a fairly successful shield against infection.
11-9. T-cell defects can result in low antibody levels
Patients with X-linked hyper IgM
syndrome (Hyper IgM Immunodeficiency, in
Case Studies in Immunology, see Preface for details) have normal B- and T-cell development and high serum levels
of IgM but make very limited IgM antibody responses against T-cell dependent
antigens and produce immunoglobulin isotypes other than IgM and IgD only in
trace amounts. This makes them highly susceptible to infection with
extracellular pathogens. The molecular defect in this disease is in the CD40
ligand expressed on activated T cells, which therefore cannot engage the CD40
molecule on B cells; the B cells themselves are normal. We learned in Chapter 9 that CD40 ligand is
critical in the T-cell dependent activation of B-cell proliferation and these
patients show that CD40 ligand is also essential for the induction of the
isotype switch and formation of germinal centers (Fig. 11.13). There are also defects in cell-mediated
immunity in these individuals. For example, they are susceptible to infection
with the opportunistic lung pathogen Pneumocystis carinii,
which is normally killed by activated macrophages. The susceptibility is thought
to be due, at least in part, to the inability of the T cells to deliver an
activating signal to infected macrophages by engaging the CD40 expressed on
these cells (see Section 8-29). A defect
in T-cell activation could also contribute to the profound immunodeficiency
suffered by these patients, as studies on mice that lack CD40 ligand have
revealed a failure of antigen-specific T cells to expand in response to primary
immunization with antigen.

Figure 11.13
Patients with X-linked hyper IgM syndrome are unable to activate their B cells fully. Lymphoid tissues in patients with hyper IgM syndrome are devoid of germinal centers (top panel), unlike a normal lymph node (bottom panel). B-cell activation by T cells (more...)
In XLA, the hunt for the cause of the disease led to the discovery of a previously unidentified gene product. In X-linked hyper IgM syndrome, the gene for CD40 ligand was cloned independently and only then identified as the defective gene in this disorder. Thus, inherited immunodeficiencies can either lead us to new genes or help us to determine the roles of known genes in normal immune system function.
11-10. Defects in complement components cause defective humoral immune function
Not surprisingly, the spectrum of infections associated with complement deficiencies overlaps substantially with that seen in patients with deficiencies in antibody production. Defects in the activation of C3, and in C3 itself, are associated with a wide range of pyogenic infections, emphasizing the important role of C3 as an opsonin, promoting the phagocytosis of bacteria (Fig. 11.14). In contrast, defects in the membrane-attack components of complement (C5–C9) have more limited effects and result exclusively in susceptibility to Neisseria species. This indicates that host defense against these bacteria, which are capable of intracellular survival, is mediated by extracellular lysis by the membrane-attack complex of complement. Accurate data from large population studies in Japan, where endemic N. meningitidis infection is rare, show that the risk each year to a normal person of infection with this organism is approximately 1/2,000,000. This compares with a risk of 1/200 in the same population to a person with inherited deficiency of one of the membrane-attack complex proteins—a 10,000-fold increase in risk compared to a person with normal complement activity. The early components of the classical complement pathway are particularly important for the elimination of immune complexes and apoptotic cells, which can cause significant pathology in autoimmune diseases such as systemic lupus erythematosus. This aspect of inherited complement deficiency is discussed in Chapter 13.

Figure 11.14
Defects in complement components are associated with susceptibility to certain infections and accumulation of immune complexes. Defects in the early components of the alternative pathway and in C3 lead to susceptibility to extracellular pathogens, particularly pyogenic (more...)
Another set of diseases are caused by defects in complement control proteins (see
Section 2-14). People lacking
decay-accelerating factor (DAF) and CD59, which protect a person's own cell
surfaces from complement activation, destroy their own red blood cells. This
results in the disease paroxysmal nocturnal hemoglobinuria, as we learned in
Chapter 2. A more striking
consequence of the loss of a regulatory protein is seen in patients with
C1-inhibitor defects. C1-inhibitor irreversibly inhibits the activity of several
serine proteinase enzymes. These include two enzymes that participate in the
contact activation system, factor XIIa (activated Hageman factor) and
kallikrein, in addition to the two enzymes that together initiate the classical
pathway of complement, C1r and C1s. Deficiency of C1-inhibitor leads to failure
to regulate these two pathways. Their unregulated activity results in the
excessive production of vasoactive mediators that cause fluid accumulation in
the tissues and epiglottal swelling that can lead to suffocation. These
mediators are bradykinin, produced by the cleavage of high molecular weight
kininogen by kallikrein and the C2 kinin, produced by the activity of C1s on
C2a. This syndrome is called hereditary
angioneurotic edema (Hereditary Angioneurotic Edema, in
Case Studies in Immunology, see Preface for details).
11-11. Defects in phagocytic cells permit widespread bacterial infections
Defects in the recruitment of phagocytic cells to extravascular sites of infection can cause serious immunodeficiency. Leukocytes reach such sites by emigrating from blood vessels in a tightly regulated process consisting of three stages. The first is the rolling adherence of leukocytes to endothelial cells, through the binding of a fucosylated tetrasaccharide ligand known as sialyl-Lewisx to E-selectin and P-selectin. Sialyl-Lewisx is expressed on monocytes and neutrophils, whereas E-selectin and P-selectin are expressed on endothelium activated by mediators from the site of inflammation. The second stage is the tight adherence of the leukocytes to the endothelium through the binding of leukocyte β2 integrins such as CD11b:CD18 (Mac-1:CR3) to counterreceptors on endothelial cells. The third and final stage is the transmigration of leukocytes through the endothelium along gradients of chemotactic molecules originating from the site of tissue injury. Neutrophil recruitment is illustrated in Fig. 2.36 and described in more detail in Section 2-22.
Deficiencies in the molecules involved in each of these stages can prevent
neutrophils and macrophages from reaching sites of infection to ingest and
destroy bacteria. Reduced rolling adhesion has been described in patients with a
lack of sialyl-Lewisx caused by a deficiency in the fucosylation
pathway responsible for its biosynthesis. Similarly, deficiencies in the
leukocyte integrin common β2 subunit CD18 have been identified and
these prevent leukocyte migration to sites of infection because of a lack of
tight leukocyte adhesion, causing the leukocyte adhesion deficiency syndrome (Leukocyte Adhesion Deficiency, in
Case Studies in Immunology, see Preface for details). All these deficiencies lead
to infections that are resistant to antibiotic treatment and that persist
despite an apparently effective cellular and humoral adaptive immune response. A
deficiency of neutrophmononils (neutropenia) associated with chemotherapy,
malignancy, or aplastic anemia is also associated with a similar spectrum of
severe pyogenic bacterial infections.
Most of the other known defects in phagocytic cells affect their ability to kill
intracellular and/or ingested extracellular bacteria (Fig. 11.15). In chronic granulomatous disease (Chronic Granulomatous Disease, in
Case Studies in Immunology, see Preface for details), phagocytes cannot produce the
superoxide radical and their antibacterial activity is thereby seriously
impaired. Several different genetic defects, affecting any one of the four
constituent proteins of the NADPH oxidase system, can cause this. Patients with
this disease have chronic bacterial infections, which in some cases lead to the
formation of granulomas. Deficiencies in the enzymes glucose-6-phosphate
dehydrogenase and myeloperoxidase also impair intracellular killing and lead to
a similar, although less severe, phenotype. Finally, in Chediak-Higashi
syndrome, a complex syndrome characterized by partial albinism,
abnormal platelet function, and severe immunodeficiency, a defect in a gene
encoding a protein involved in intracellular vesicle formation causes a failure
to fuse lysosomes properly with phagosomes; the phagocytes in these patients
have enlarged granules and impaired intracellular killing.

Figure 11.15
Defects in phagocytic cells are associated with persistence of bacterial infection. Defects in the leukocyte integrins with a common β2 subunit (CD18) or defects in the selectin ligand, sialyl-Lewisx, prevent phagocytic cell adhesion and migration (more...)
11-12. Defects in T-cell function result in severe combined immunodeficiencies
Although patients with B-cell defects can deal with many pathogens adequately, patients with defects in T-cell development are highly susceptible to a broad range of infectious agents. This demonstrates the central role of T cells in adaptive immune responses to virtually all antigens. As such patients make neither specific T-cell dependent antibody responses nor cell-mediated immune responses, and thus cannot develop immunological memory, they are said to suffer from severe combined immunodeficiency (SCID).
Several different defects can lead to the SCID phenotype. In X-linked SCID (X-linked SCID, in
Case Studies in Immunology, see Preface for details), which
is the commonest form of SCID, T cells fail to develop because of a mutation in
the common γ chain of several cytokine receptors, including those for the
interleukins IL-2, IL-4, IL-7, IL-9, and IL-15. We will examine this defect
further in Section 11-13. The commonest
forms of autosomally inherited SCID are due to adenosine deaminase
(ADA) deficiency (
Adenosine Deaminase Deficiency, in
Case Studies in Immunology, see Preface for details) and purine nucleotide phosphorylase (PNP)
deficiency. These enzyme defects affect purine degradation, and
both result in an accumulation of nucleotide metabolites that are particularly
toxic to developing T cells. B cells are also somewhat compromised in these
patients.
One class of SCID individuals lack expression of all MHC class II gene products
on their cells (MHC Class II Deficiency, in
Case Studies in Immunology, see Preface for details). This condition is also referred to as the bare lymphocyte syndrome as MHC class
II molecules are not expressed on lymphocytes or thymic epithelial cells. As the
thymus in such individuals lacks MHC class II molecules, CD4 T cells cannot be
positively selected and therefore few develop. The antigen-presenting cells in
these individuals also lack MHC class II molecules and so the few CD4 T cells
that do develop cannot be stimulated by antigen. In these individuals, MHC class
I expression is normal and CD8 T cells develop normally. However, such people
suffer from severe combined immunodeficiency, illustrating the central
importance of CD4 T cells in adaptive immunity to most pathogens. The syndrome
is caused not by mutations in the MHC genes themselves, but by mutations in one
of several different genes encoding gene-regulatory proteins that are required
for the transcriptional activation of MHC class II promoters. Four complementing
gene defects (known as Groups A, B, C, and D) have been defined in patients who
fail to express MHC class II molecules, which implies that at least four
different genes are required for normal MHC class II gene expression. One of
these, named the MHC class II
transactivator, or CIITA, is the gene mutated in Group
A. The genes mutated in Groups B, C, and D are named RFXANK, RFX5, and RFXAP.
These genes encode three proteins that are components of a multimeric
transcriptional complex, RFX, which binds a sequence named an X box, present in
the promoter of all MHC class II genes.
In contrast, a more limited immunodeficiency, associated with chronic respiratory bacterial infections and skin ulceration with vasculitis, has been observed in a small number of patients showing almost complete absence of cell-surface MHC class I molecules. This condition has been labeled bare lymphocyte syndrome (MHC class I). Affected individuals have normal levels of mRNA encoding MHC class I molecules and normal production of MHC class I proteins, but these proteins reach the cell surface in severely reduced numbers. The defect was shown to be similar to that in the TAP mutant cells mentioned in Section 5-2 and, indeed, affected patients have been found to have mutations in the TAP1 or TAP2 genes that encode the two subunits of the peptide transporter. The absence of MHC class I molecules at the cell surface leads to a lack of CD8 T cells expressing the α:β T cell receptor, but these patients do have CD8 T cells that bear the γ:δ receptor. It is surprising that they are not abnormally susceptible to viral infections, given the key role of MHC class I presentation and cytotoxic CD8 α:β T cells in the control of viral infections. However, there is evidence for TAP-independent pathways of antigen presentation by MHC class I molecules of certain peptides. The clinical phenotype of TAP1- and TAP2-deficient patients illustrates that these pathways may be sufficient to allow the control of viral infections.
Another set of defects leading to SCID are those that cause failures of DNA
rearrangement in developing lymphocytes. For example, defects in either the
RAG-1 or RAG-2 genes result in the arrest
of lymphocyte development because of a failure to rearrange the antigen receptor
genes. Thus there is a complete lack of T and B cells in mice with genetically
engineered defects in the RAG genes, and in patients with
autosomally inherited forms of SCID who lack a functional RAG protein. There are
other patients with mutations in either the RAG-1 or
RAG-2 genes who can nonetheless make a small amount of
functional RAG protein, allowing a small amount of V(D)J recombination activity.
They suffer from a distinctive and severe disease called Omenn's syndrome (Omenn Syndrome, in
Case Studies in Immunology, see Preface for details), in
which, in addition to increased susceptibility to multiple opportunistic
infections, there are also clinical features very similar to graft-versus-host
disease (see Section 13-21) with rashes,
eosinophilia, diarrhea, and enlargement of the lymph nodes. Normal or elevated
numbers of T cells, all of which are activated, are found in these unfortunate
children. A possible explanation for this phenotype is that very low levels of
RAG activity allow some limited T-cell receptor gene
recombination. However, no B cells are found and it may be that B cells have
more stringent requirements for RAG activity. The T cells that
are produced in these patients show an abnormal and highly restricted receptor
repertoire, both in the thymus and in the periphery, where they have undergone
clonal expansion and activation. The clinical features strongly suggest that
these peripheral T cells are autoreactive and responsible for the
graft-versushost phenotype.
Another group of patients with autosomal SCID have a phenotype very similar to that of a mutant mouse strain called scid; scid mice suffer from an abnormal sensitivity to ionizing radiation as well as from severe combined immuno-deficiency. They produce very few mature B and T cells, as there is a failure of DNA rearrangement in their developing lymphocytes; only rare VJ or VDJ joints are seen and most of these have abnormal features. The underlying defect has now been shown to be in the enzyme DNA-dependent protein kinase (DNA-PK), which binds to the end of the double-stranded breaks that occur during the process of antigen receptor gene rearrangement. These ends are found as DNA hairpin structures in the immature thymocytes of scid mice. Thus, it seems likely that DNA-PK is involved in resolving the hairpin structure (see Section 4-5).
Other defects in DNA repair and metabolizing enzymes are associated with a combination of immunodeficiency, increased sensitivity to the damaging effects of ionizing radiation, and cancer development. One example is Bloom's syndrome, a disease caused by mutations in a DNA helicase enzyme, which unwinds DNA. Another is ataxia telangiectasia (AT), in which the underlying defect is in a protein called ATM, which contains a kinase domain thought to be involved in intracellular signaling in response to DNA damage. Because repair of double-stranded DNA breaks and lymphocyte division are central to the function of the adaptive immune system, it is not surprising that defects such as these are associated with the development of immunodeficiency.
Finally, in patients with DiGeorge's syndrome the thymic epithelium fails to develop normally. Without the proper inductive environment T cells cannot mature, and both T-cell dependent antibody production and cell-mediated immunity are absent. Such patients have some serum immuno-globulin and variable numbers of B and T cells. As with all the severe combined immuo-deficiency diseases, it is the defect in T cells that is crucial. These diseases abundantly illustrate the central role of T cells in virtually all adaptive immune responses. In many cases B-cell development is normal, yet the response to nearly all pathogens is profoundly impaired.
11-13. Defective T-cell signaling, cytokine production, or cytokine action can cause immunodeficiency
As we learned in Chapter 8, virtually all adaptive immune responses require the activation of antigen-specific T lymphocytes and their differentiation into cells producing cytokines that act on specific cytokine receptors. Several gene defects have been described that interfere with these processes. Thus, patients who lack CD3γ chains have low levels of surface T-cell receptors and defective T-cell responses. Patients making low levels of mutant CD3ε chains are also deficient in T-cell activation. Patients who make a defective form of the cytosolic protein tyrosine kinase ZAP-70, which transmits signals from the T-cell receptor (see Section 6-9) have recently been described. Their CD4 T cells emerge from the thymus in normal numbers, whereas CD8 T cells are absent. However, the CD4 T cells that mature fail to respond to stimuli that normally activate via the T-cell receptor and the patients are thus very immunodeficient.
Another group of patients show a lack of IL-2 production upon receptor ligation, and these patients have a severe immunodeficiency; however, T-cell development is normal in these individuals, as it is in mice in which mutations have been made in their IL-2 genes by gene knockout (see Appendix I, Section A-47). These IL-2-negative patients have heterogeneous defects; some of them fail to activate the transcription factor NFAT (see Section 6-11), which induces the transcription of several cytokine genes in addition to the IL-2 gene. This might explain why their immunodeficiency is more profound than that of mice whose IL-2 gene has been disrupted. IL-2-deficient mice can mount adaptive immune responses through an IL-2-independent pathway, possibly involving the cytokine IL-15, which shares many activities with IL-2; nevertheless, they are susceptible to a variety of infectious agents.
In contrast to the normal development of T cells in patients deficient in IL-2,
there is a failure of T-cell development in patients with X-linked severe combined
immunodeficiency (X-linked SCID) (X-linked SCID, in
Case Studies in Immunology, see Preface for details), which is caused by a
defect in the γ chain of the IL-2 receptor. Thus, this disease showed that the
common γ chain (γc) must be important in T-cell development for
reasons unrelated to IL-2 binding or IL-2 responses. The demonstration that the
IL-2 receptor γc chain is also part of other cytokine receptors,
including the IL-7 receptor, helps to explain its role in early T-cell
development. The γ chain seems to function in transducing the signal from this
group of receptors and interacts with a kinase, JAK3 kinase, which is known to
be defective in patients with an autosomally inherited immunodeficiency similar
in phenotype to X-linked SCID.
As in all serious T-cell deficiencies, X-linked SCID patients do not make effective antibody responses to most antigens, although their B cells seem normal. However, as the gene defect is on the X chromosome, one can determine whether the lack of B-cell function is solely a consequence of the lack of T-cell help by examining X-chromosome inactivation (see Section 11-7) in B cells of unaffected carriers. The majority of naive IgM-positive B cells from female carriers of X-linked SCID have inactivated the defective X chromosome rather than the normal one, showing that B-cell development is affected by, but not wholly dependent on, the common γ chain. However, mature memory B cells that have switched to isotypes other than IgM have inactivated the defective X chromosome almost without exception. This might reflect the fact that the IL-2 receptor γ chain is also part of the IL-4 receptor. Thus, B cells that lack this chain will have defective IL-4 receptors and will not proliferate in T-cell-dependent antibody responses. X-linked SCID is so severe that children who inherit it can survive only in a completely pathogen-free environment, unless given antibodies and successfully treated by bone marrow transplantation. A famous case in Houston became known as the ‘bubble baby’ because of the plastic bubble in which he was enclosed to protect him from infection.
Wiskott-Aldrich syndrome
(WAS) (Wiskott-Aldrich Syndrome, in
Case Studies in Immunology, see Preface for details) is a disease that has shed new light on the molecular
basis of T-cell signaling and its importance for immune function. The disease
affects platelets and was first described as a blood-clotting disorder, but it
is also associated with immunodeficiency due to impaired T-cell function,
reduced T-cell numbers, and a failure of antibody responses to encapsulated
bacteria. WAS is caused by a defective gene on the X chromosome, encoding a
protein called WAS protein (WASP). This protein has been shown to bind Cdc42, a
small GTP-binding protein that is known to regulate the organization of the
actin cytoskeleton and to be important for the effective collaboration of T and
B cells. WASP might have a role in regulating changes in the actin cytoskeleton
in response to external stimuli. It has the ability to bind SH3 domains, which,
as we saw in Chapter 6, have an
affinity for amino acid sequences rich in proline that are found on some
proteins of intracellular signaling pathways. In WAS patients, and in mice whose
WASP gene has been knocked out, T cells fail to respond normally to mitogens or
to the cross-linking of surface receptors. Cytotoxic T-cell responses are also
impaired, and T-cell help for B-cell responses to polysaccharide antigens is
lacking. WASP is expressed in all hematopoietic cell lineages and is likely to
be a key regulator of lymphocyte and platelet development and function.
11-14. The normal pathways for host defense against intracellular bacteria are illustrated by genetic deficiencies of IFN-γ and IL-12 and their receptors
A small number of families have been identified containing several individuals
who suffer from persistent and eventually fatal attacks by intracellular
pathogens, especially mycobacteria and salmonellae. Typically these patients
suffer from the ubiquitous, environmental nontuberculous strains of
myco-bacteria, such as Mycobacterium avium. They may also
develop disseminated infection after vaccination with Mycobacterium
bovis bacillus Calmette-Guérin, the strain of M.
bovis that is used as a live vaccine against M.
tuberculosis. The molecular bases of the susceptibility to these
infections are null mutations in one of the following genes: IL-12, the IL-12
receptor β1 chain, or either of the two protein subunits, R1 and R2, of the
receptor for IFN-γ. Similar susceptibility to intracellular bacterial infection
is seen in mice with induced mutations in these same genes and also in mice
lacking TNF-α or the TNF p55 receptor gene. All these genes must therefore play
a critical part in the normal mechanisms of host defense against infection by
these intracellular bacteria.(Interferon-γ Receptor Deficiency, in
Case Studies in Immunology, see Preface for details)
Mycobacteria and salmonellae enter dendritic cells and macrophages, where they can reproduce and multiply. At the same time they provoke an immune response that involves several stages and eventually controls the infection with the help of CD4 T cells (Fig. 11.16). First, lipoproteins from the surface of the bacteria ligate receptors on macrophages and dendritic cells as they enter the cells. These receptors include the Toll-like receptors (see Section 2-16), particularly TLR-2, and their ligation stimulates nitric oxide (NO) production within the cells, which is toxic to the bacteria. Signaling by these Toll-like receptors also stimulates the release of IL-12 which, in turn, stimulates CD4 T cells to release IFN-γ and TNF-α. These cytokines activate and recruit more mononuclear phagocytic cells to the site of infection, resulting in the formation of granulomas. The key role of IFN-γ in activating macrophages to kill intracellular bacteria is illustrated dramatically by the failure to control infection in patients who are genetically deficient in either of the two subunits of this receptor. In the total absence of IFN-γ receptor expression, granuloma formation is much reduced, showing a role for this receptor in the development of granulomas. In contrast, if the underlying mutation is associated with the presence of low levels of functional receptor, granulomas form, but the macrophages within the granulomas are not sufficiently activated to be able to control the division and spread of the mycobacteria. It is important to appreciate that this cascade of cytokine reactions is occurring in the context of cognate interactions between the macrophages and dendritic cells harboring the intracellular bacteria and antigen-specific CD4 T cells. T-cell receptor ligation and co-stimulation of the phagocyte by, for example, CD40–CD40 ligand interaction are important components that augment the capacity of T cells to effectively activate the infected phagocytes to kill the intracellular bacteria (see Sections 8-26 and 8-28).

Figure 11.16
The expression of Toll-like receptor 2 (TLR-2) allows macrophages to respond effectively to mycobacteria. TLR-2 is activated on binding the polysaccharide coat of mycobacteria, stimulating internalization of the bound bacteria, and the expression of nitric (more...)
11-15. X-linked lymphoproliferative syndrome is associated with fatal infection by Epstein-Barr virus and with the development of lymphomas
Epstein-Barr virus is a herpes virus that infects the majority of the human race and remains latent in B cells throughout life after primary infection. EBV infection can transform B lymphocytes and is used as a technique for immortalizing clones of B cells in the laboratory. This does not normally happen in vivo in humans because EBV infection is actively controlled and maintained in a latent state by cytotoxic T cells with specificity for B cells expressing EBV antigens (see Section 11-2). In the presence of T-cell immunodeficiency, this control mechanism can break down and a potentially lethal B-cell lymphoma may develop. One of the situations in which this occurs is the rare immuo-deficiency, X-linked lymphoproliferative syndrome, which results from mutations in a gene named SH2-domain containing gene 1A (SH2D1A). Boys with this deficiency typically develop overwhelming EBV infection during childhood, and sometimes lymphomas. EBV infection in this condition is usually fatal and is associated with necrosis of the liver. Thus SH2D1A must play a vital, nonredundant role in the normal control of EBV infection.
The function of SH2D1A is partly understood. The SH2 domain of the protein interacts with the cytoplasmic tails of two transmembrane receptors, SLAM and 2B4, which are structurally homologous to each other, and to the T-cell adhesion molecule CD2 (see Section 8-4). SLAM (signaling lymphocyte activation molecule) is expressed on activated T cells, whereas 2B4 is found on T cells, B cells, and NK cells. Activation of these receptors initiates a signaling pathway by the recruitment of the tyrosine phosphatase, SHP-2 (see Section 6-14). It appears that the function of SH2D1A is to inhibit the recruitment of SHP-2 and thereby to inhibit cellular activation by SLAM and 2B4. There are two hypotheses to explain the pathogenesis of the fatal EBV infection seen in children with defects in SH2D1A. The first is that failure of T cells to kill B cells expressing antigens from multiplying EBV allows uncontrolled infection. The second is that B cells presenting EBV peptides uncontrollably activate T cells and that cytotoxic T cells cause tissue necrosis and death. Some cases of lymphoma in young boys have now been found associated with mutations in the SH2D1A gene in the absence of any evidence of EBV infection. This raises the possibility that SH2D1A may be a tumor suppressor gene in its own right, in addition to controlling a virus that can contribute to tumor formation.
11-16. Bone marrow transplantation or gene therapy can be useful to correct genetic defects
It is frequently possible to correct the defects in lymphocyte development that lead to the SCID phenotype by replacing the defective component, generally by bone marrow transplantation. The major difficulties in these therapies result from MHC polymorphism. To be useful, the graft must share some MHC alleles with the host. As we learned in Section 7-20, the MHC alleles expressed by the thymic epithelium determine which T cells can be positively selected. When bone marrow cells are used to restore immune function to individuals with a normal thymic stroma, both the T cells and the antigen-presenting cells are derived from the graft. Therefore, unless the graft shares at least some MHC alleles with the recipient, the T cells that are selected on host thymic epithelium cannot be activated by graft-derived antigen-presenting cells (Fig. 11.17). There is also a danger that mature, post-thymic T cells in donor bone marrow might recognize the host as foreign and attack it, causing graft-versus-host disease (GVHD) (Fig. 11.18, top panel). This can be overcome by depleting the donor bone marrow of mature T cells. Bone marrow recipients are usually treated with irradiation that kills their own lymphocytes, thus making space for the grafted bone marrow cells and minimizing the threat of host-versus-graft disease (HVGD) (Fig. 11.18, third panel). In patients with the SCID phenotype, however, there is little problem with the host response to the transplanted bone marrow, as the patient is immunodeficient.

Figure 11.17
Bone marrow donor and recipient must share at least some MHC molecules to restore immune function. In an allogeneic bone marrow transplant, the donor marrow cells share some MHC molecules with the recipient. The shared MHC type is designated b and illustrated (more...)

Figure 11.18
Bone marrow grafting can be used to correct immuno-deficiencies caused by defects in lymphocyte maturation but two problems can arise. First, if there are mature T cells in the bone marrow, they can attack cells of the host by recognizing their MHC antigens, (more...)
Now that specific gene defects are being identified, a different approach to correcting these inherited immune deficiencies can be attempted. The strategy involves extracting a sample of the patient's own bone marrow cells, inserting a normal copy of the defective gene into them, and returning them to the patient by transfusion. This approach, called somatic gene therapy, should correct the gene defect. Moreover, in immunodeficient patients, it might be possible to reinfuse the bone marrow into the patient without the usual irradiation used to suppress the recipient's bone marrow function. There is no risk of graft-versus-host disease in this case, although the host might respond to the replaced gene product and reject the engineered cells. Although this kind of approach is theoretically attractive, efficient transfer of genes into bone marrow stem cells is technically difficult and has been achieved only in mouse models. The first trials of gene therapy for correcting immunodeficiency, such as the treatment of a child with ADA deficiency at the National Institute of Health (NIH) in 1990, used the patient's lymphocytes as the vehicle for gene introduction. However, because most lymphocytes divide regularly, thus diluting out the new gene, the treatment had to be repeated regularly. In another study, bone marrow stem cells, obtained from cord blood from three patients with ADA deficiency were transduced with the ADA gene and reinfused. At the age of 4 years, these children expressed up to 10% of normal ADA levels only in T cells and not in other bone marrow-derived cells, and they remained immunodeficient in the absence of treatment with ADA enzyme replacement. More recently, however, there has been a successful attempt at correcting the phenotype of two X-linked SCID patients using a Moloney retrovirus-derived construct containing the γc chain to infect bone marrow stem cells.
Summary
Genetic defects can occur in almost any molecule involved in the immune response. These defects give rise to characteristic deficiency diseases, which, although rare, provide a great deal of information about the development and functioning of the immune system in normal humans. Inherited immuo-deficiencies illustrate the vital role of the adaptive immune response and T cells in particular, without which both cell-mediated and humoral immunity fail. They have provided information about the separate roles of B lymphocytes in humoral immunity and of T lymphocytes in cell-mediated immunity, the importance of phagocytes and complement in humoral and innate immunity, and the specific functions of several cell-surface or signaling molecules in the adaptive immune response. There are also some inherited immune disorders whose causes we still do not understand. The study of these diseases will undoubtedly teach us more about the normal immune response and its control.
- A history of repeated infections suggests a diagnosis of immunodeficiency
- Inherited immunodeficiency diseases are caused by recessive gene defects
- The main effect of low levels of antibody is an inability to clear extracellular bacteria
- T-cell defects can result in low antibody levels
- Defects in complement components cause defective humoral immune function
- Defects in phagocytic cells permit widespread bacterial infections
- Defects in T-cell function result in severe combined immunodeficiencies
- Defective T-cell signaling, cytokine production, or cytokine action can cause immunodeficiency
- The normal pathways for host defense against intracellular bacteria are illustrated by genetic deficiencies of IFN-γ and IL-12 and their receptors
- X-linked lymphoproliferative syndrome is associated with fatal infection by Epstein-Barr virus and with the development of lymphomas
- Bone marrow transplantation or gene therapy can be useful to correct genetic defects
- Summary
- Inherited immunodeficiency diseases - ImmunobiologyInherited immunodeficiency diseases - Immunobiology
Your browsing activity is empty.
Activity recording is turned off.
See more...