S. David Webb And Neil D. Opdyke
University of Florida
Abstract
The Cenozoic succession of continental mammalian faunas reflects the climatic shift from a "greenhouse" to an "icehouse" world. In midcontinental North America, where the most nearly complete record of terrestrial biotic succession has been assembled, floral and faunal evidence chronicle a broad progression of biome change from tropical forest to savanna to steppe. Thanks to improved land mammal chronologies, the tempo of faunal turnover is now seen to be strikingly syncopated. Stately chronofaunas, made up of stable sets of slowly evolving taxa, persist on the order of 107 yr. Chronofaunas are terminated by rapid turnover episodes in which some native taxa experience rapid evolutionary rates and other taxa appear as intercontinental immigrants.
We focus here on pulses of immigration and their correlation with major climatic shifts. The Tertiary record of North American land mammals registers a total of 140 immigrant genera. The chronology of most immigrant arrivals can be placed within the nearest million years (m.y.) or less. The pattern of immigration is decidedly nonrandom, with the largest two episodes involving 15 and 14 genera each within about 1 m.y. We categorize immigrant arrivals into first-, second-, and third-order episodes, with the first consisting of at least nine genera within less than 1 m.y. The seven first-order episodes occur at 58.5, 57, 40, 20, 18.5, 5, and 2.5 m.y. ago (Ma). The first-order episode at ~40 Ma may represent an artifact due to tallying at the end of a long interval of poorly fossiliferous deposits (Uintan). A striking generalization is that the six other first-order episodes occur as three pairs separated by only about 2 m.y.
Review of land mammal immigration and rapid turnover episodes on other continents tends to confirm the "universality" of major immigration episodes at ~20, ~5, and ~2.5 Ma.
We consider two hypotheses that might causally relate high intercontinental immigration rates to global climate change. The first proposes a correlation between high immigration rates and the availability of land bridges during low sea-level intervals. In this view, the marine record of positive oxygen isotopes excursions can be read as a proxy for eustatic sea-level lowering. The second hypothesis relates first-order immigration episodes to high faunal turnover intervals and considers them to represent fundamental reorganizations of the continental ecosystem. In this view, the largest positive oxygen isotope excursions record major cooling episodes, which also radically alter terrestrial ecosystems. We consider both hypotheses as we compare the immigration data from the North American mammal record with oxygen isotope data from the marine realm.
The predicted correlation between first-order immigration episodes and rapid climatic cooling or broader land bridges, as represented by the oxygen isotope record, is corroborated in some cases but miscorrelated in others. Two clearly contradictory episodes are the Oligocene (35 to 30 Ma) and the Middle Miocene (16 to 6 Ma) when, despite major global cooling events, immigration rates were exceedingly low in North America. Possibly no land bridges were accessible in the Oligocene, but this explanation is less likely during the Middle Miocene when Beringian routes were probably accessible. It is more likely that these intervals carried few immigrants because they represent the two most stable chronofaunal phases in North American mammalian history. The Clarendonian chronofauna of the Middle Miocene, despite several episodes of global cooling and severe sea-level excursions, remained nearly closed to intercontinental immigrants. During such robust chronofaunal intervals the continental mammal fauna stood near its ecological capacity.
This analysis of the land mammal record indicates that climatic shifts represented by first-order changes in the isotopic record are necessary but not sufficient causes of first-order immigration episodes into the North American continent. Clearly the question of why the continental ecosystem was open to immigration during certain times of isotopic excursions and resilient to others has fundamental significance in understanding the stability of present and future continental ecosystems.
Introduction
The rich record of Cenozoic land mammals provides not only a panoramic perspective on evolutionary processes, but also a valuable history of environmental change on the continents. As presently known, Cenozoic mammals number about 4500 genera, including 1051 living genera (M. McKenna, American Museum of Natural History, personal communication, 1992). Excluding bats, the number of extinct genera exceeds that of living genera by a factor of about four. Taxonomically and stratigraphically, land mammals are especially well documented in North America and Europe; on most other continents they are moderately well documented but with significant chronological gaps. Even on Antarctica, fossil mammals are now represented, albeit by only a few Paleogene genera from Seymour Island. Improved methods for sampling smaller forms (e.g., screenwashing and flotation) and continued success in discovering fossils in previously unsampled regions have greatly strengthened the fabric of the global mammalian succession.
Similarly, the chronostratigraphic framework for recording the Cenozoic history of land mammals has vastly improved in the past two decades. The succession of fossils, including evolutionary events, immigrations, and extinctions, provides the fundamental data set, but these biostratigraphic data are integrated via the stratigraphic context with other methodologies, most importantly with radiometric dates (principally K/Ar and more recently Ar/ Ar) and paleomagnetic chronology (Lindsay et al., 1984). In North America, the Cenozoic Era is divided into 19 land mammal ages, including 52 (mainly informal but widely recognized) subdivisions (Woodburne, 1987). Thus 66 m.y. of Cenozoic mammalian history in North America can be divided into units that average less than 1.3 m.y. In the late Cenozoic the precision of mammal faunal dates approaches 0.5 m.y., and in favorable sections, wherein paleomagnetic and radiometric data are integrated with biostratigraphic data, particular strata may be resolved chronologically to less than 0.1 m.y. Such sections are not uncommon in western North America (Woodburne, 1987).
As the history of mammalian evolution has become more precisely calibrated, it has become increasingly evident that its tempo is strongly syncopated. Nowhere is that more evident than in the record of land mammals that immigrated to North America. We show that about 70% of immigrant genera were concentrated during about 10% of the Tertiary Period. Of the seven first-order immigration episodes that we define, one may be artifactual, and the six "real" ones occur in closely yoked pairs. In this chapter we investigate the global and regional environmental significance of such major land mammal immigration episodes.
Present societal concerns about global climatic change focus primarily on possible drastic alterations of continental ecosystems. Paradoxically, the most useful perspective on climatic history comes from the marine record of the Cenozoic Era, particularly from stable isotopic analyses of deep-sea cores. Such marine records do not directly yield the greater extremes of temperature and other environmental signals that continents experience. Accordingly, a critical area of current research concerns avenues for linkage between marine climatic records and the behavior of terrestrial ecosystems. That is why the record of land mammal faunas, by providing sufficient geochronological precision to be correlated with the marine record, holds special relevance to current considerations about global change.
Land mammal faunal succession may reflect climatic change in two somewhat distinct manners. First, the adaptive features of a given assemblage may be viewed in relation to the presumed conditions for which it was adapted; also, by analogy with living communities of similar structure, its composition may indicate the kind of landscape in which it lived. Secondly, in a largely independent and nonbiological manner, quantitative analysis of faunal turnover helps pinpoint times of ecosystem stability or, alternatively, of rapid reorganization. Rapid faunal turnover episodes may be correlated with key events in floristics, sedimentary regimes, isotopic signals, or other independent evidence of climate change episodes.
We pursue both of these approaches in the context of Cenozoic land mammals of North America. First, we review the land mammal record of North America and, together with other botanical and sedimentological evidence, indicate the general succession of ecosystems and landscapes. Second, we quantify the record of intercontinental immigration to North America, placing special emphasis on first-order episodes. Finally, we correlate this continental record with the marine record of global climate change. We show that the continental ecosystem did not respond uniformly to climatic episodes indicated in the marine record. We conclude that such shifts were a necessary but not a sufficient condition for faunal turnover and terrestrial ecosystem reorganization.
Land Mammal Faunas and Their Response to Climatic Change
The richest continental record of Cenozoic mammals is that of North America. We concentrate on that record and methods of recognizing its response to climatic change. Several recent reviews of the mammalian succession may be noted. Woodburne (1987) offers a modern geochronological revision of the North American mammalian succession, and Lindsay et al. (1990) refine and update the biostratigraphy of Neogene mammal faunas in Europe. Savage and Russell (1983) provide comprehensive coverage of all continental and marine mammal faunas. Stucky (1990) reviews paleoecology and diversity change in North American Paleogene faunas, and Webb (1977, 1983a, 1989) treats Neogene faunal dynamics in North America. Figure 11.1 presents the geochronological and biostratigraphic framework of North American land mammals during the Tertiary.

Figure 11.1
Land mammal immigration episodes in the Cenozoic of North America. Mammal ages, as correlated with Cenozoic Epochs, magnetic time scale, and time in millions of years, follow Woodburne (1987). First-order immigration episodes (with eight or more genera) (more...)
Chronofaunas and Turnover Pulses
Vertebrate paleontologists refer to persistent faunas of essentially uniform taxonomic composition and stable diversity as chronofaunas following Olson (1952). In ecological terms, chronofaunas are thought to represent stable coadapted sets of species, and their detailed histories (under various perturbations) shed light on the theory of how communities or community complexes are structured (Olson, 1983; Webb, 1987). Five chronofaunas account for most of the stable intervals of the North American Cenozoic. They are the Eocene chronofauna (Krause and Maas, 1990); the White River chronofauna (Emry, 1981; Krishtalka et al., 1987); and in the Miocene, the Runningwater, the Sheep Creek, and the Clarendonian chronofaunas (Webb, 1983a; Tedford et al., 1987). Only in the extreme phases of the icehouse world, during the Pliocene and the Pleistocene, did the stability and persistence of mammalian chronofaunas breakdown.
These stable chronofaunas are followed by relatively brief episodes of rapid faunal turnover. Such rapid turnover episodes (RTE) have been recognized and studied during the past decade, following the establishment of relatively precise geochronometry. Several students of mammalian faunas have documented that even during an RTE the correlation between numbers of first appearances and last appearances is maintained, indicating an equilibrium process in which faunal interactions maintain a balance between gains and losses (Gingerich, 1984; Webb, 1989; Stucky, 1990). Gingerich (1984) proposed that the high peak of land mammal extinctions in the late Pleistocene of North America was an equilibrium response compensating for the high number of immigrations in the Late Pliocene and early Pleistocene; the 2-m.y. time lag tended to conceal the correlation. Others have explained RTE patterns in terms of climatic forcing. Webb (1983b) distinguished two types of rapid turnover episodes, the criterion being whether extinction waves or immigration waves peaked first, and suggested that the former (E-type RTE) were probably caused by climatic changes. He also showed that during the Neogene, six land mammal extinction episodes (including the two largest in North America) were correlated with positive oxygen isotope excursions in
the marine record and thus with global climatic episodes (Webb, 1984). When chronofaunal stability prevails, member taxa generally experience stabilizing selection. Only when climatic change perturbs the terrestrial ecosystem sufficiently does it produce an RTE.
Wing and Tiffney (1987) described similar patterns in floristic stability and turnover. They found that "the chief mechanism for drastic reorganization in community structure" was "severe climatic change." A similar view was formally proposed as the turnover-pulse hypothesis by Vrba (1985a, p. 232) in the following terms:
Speciation does not occur unless forced (initiated) by changes in the physical environment. Similarly, forcing by the physical environment is required to produce extinctions and most migration events. Thus, most lineage turnover in the history of life has occurred in pulses, nearly (geologically) synchronous across diverse phylogenies, and in synchrony with changes in the physical environment.
This view underlies our analysis of immigration episodes in land mammal faunas. We attempt to test such terrestrial turnover patterns by comparing them with the isotopic record of Cenozoic climate change, which is derived independently from marine sediments.
Importance of Immigrants
In the following analysis we take the number of immigrant genera in each land mammal age as a key to faunal turnover episodes. Immigrants (first appearances) are recognized on the basis of two criteria: (1) the absence of that genus or closely related genera in prior North American records and (2) the presence of related forms (sister group) earlier in another continental fauna. Mammalian biostratigraphers have increasingly focused on immigrant taxa as the best diagnostic basis for defining land mammal ages, as well as informal subages or zones (Woodburne, 1987). Thus, for both its environmental and its stratigraphic significance, the record of immigrant mammals in North America has been well documented.
Figure 11.1 identifies major episodes of mammalian immigrations into North America during the Tertiary; we designate as first-order immigration episodes those that number nine or more genera. Second-order episodes are those with five to seven genera. These data are more fully explained in the following sections on results. Biostratigraphy and chronostratigraphy follow Woodburne (1987). These data will continue to be refined, by addition of immigrant genera, by extension of their known ranges into earlier intervals, and by refinements in the geochronological framework.
Three land bridges controlled the access of Cenozoic land mammals to North America. In the latest Paleocene and early Eocene the North Atlantic Thulean route between Europe and North America provided the basis for a strong faunal resemblance between these two continents. The next major immigration episode into North America took place in the Late Eocene. The mammals of this episode may have come across the Bering Strait, but more probably they again tracked the Thulean land bridge, which did not founder to great depth until the Oligocene (Rowley and Lottes, 1988). Subsequent episodes of faunal dispersal from the Old World must have crossed via the Bering land bridge. The very high latitude of this route (at the North Pole in the Cretaceous) placed increasingly severe ecological constraints on the immigrants that traversed it. Even as Beringian access shifted to somewhat lower latitudes during the Neogene, climatic regimes became more severe, so that Beringia continued to function as a narrow ecological filter. During the Pliocene (Blancan land mammal age) the Bering land bridge provided passage for temperate woodland species, such as an extinct deer (Bretzia) and an extinct panda (Parailurus), to the Pacific Northwest (Tedford and Gustafson, 1974). By the Pleistocene, however, the Bering filtered out all but steppe and steppe-tundra species, with the exception of humans who by then had developed highly specialized cultural adaptations to life in Beringian steppe-tundra.
A third route for intercontinental access to North America came from the south via the Panama (isthmian) land bridge. By Late Pliocene time it facilitated immigrations from South America into North America, mainly representing species adapted to subtropical savanna (Stehli and Webb, 1985).
In the following section we review the environmental history of mammalian faunas in North America, with an indication of the predominant biome during each chronofauna. We also briefly discuss the immigration (and faunal turnover) episodes that punctuate the chronofaunal succession. A major unresolved problem stems from the different durations of the land mammal ages in current use. The Duchesnean, for example, may span as much as 4 m.y. and the preceding Uintan as long as 6 m.y. Although it might be appropriate to treat immigrations as a rate by making the assumption that immigration events were uniformly distributed through a mammal age, in the present context we make no a priori assumptions about how immigrations may have been distributed within a mammal age subdivision, placing them simply at the stratigraphic level advocated by the best available empirical studies. We follow with the caveat that long intervals are more likely to produce artificially high immigration numbers, and note the need for continued refinement of fossiliferous local sections, especially in the mid-Cenozoic. Before we examine the possible correlations between immigration episodes and global climatic shifts, we briefly review the history of changing biomes in North America.
Recognition of Land Mammal Biomes
Several decades before the evolution of horses was documented in western North America, Kowalevsky (1873), working in Europe, recognized that hypsodonty in hipparionine horses reflected the spread of grasslands in the Miocene. Such reconstructions of environmental history from fossil mammal assemblages rest on two fundamental premises. The first is that the fossil record adequately samples the kinds and numbers of land mammal genera that lived during successive stages of the Cenozoic. The second premise is that samples of once-living mammals, through their morphologies, distributions, and numbers, and also through their sedimentary and taphonomic contexts, bear evidence of their environments. Such relationships are well established between living mammals and present-day environments; for example, the diversity and structure of African ungulate faunas reflect the vegetative cover and rainfall in which they range (Coe et al., 1976; Sinclair and Norton-Griffiths, 1979; Vrba, 1985b; McNaughton and Georgiadis, 1986). Webb (1984) and Janis (1984) independently analyzed the distribution of body size and hypsodonty in fossil ungulate faunas from North America to develop convincing analogues with modern ungulate faunas in Africa. More generally, Andrews et al. (1979) empirically determined the patterns of body size and other gross features that characterize modern mammalian faunas from various terrestrial biomes regardless of the continent on which they occur.
One particular method of faunal inference that has been applied with considerable success in recent years is called the cenogram. A cenogram displays the whole spectrum of estimated body sizes for all species from a relatively complete faunal sample, for comparison with other such cenograms representing known biomes. Legendre (1986) convincingly used this method to show that the land mammal cenogram for the Late Eocene Phosphorites of Quercy in France had the same pattern as a modern equatorial rain forest fauna, notably a predominance of small-bodied (arboreal) forms, and Gingerich (1989) applied the same method with excellent results to still earlier Eocene (Wasatchian) faunas in Wyoming. Other examples are reviewed in Behrensmeyer et al. (1992).
Actualistic studies of taphonomic processes in the deposition of mammal remains provide the critical link between modern and fossil ecosystems (Behrensmeyer and Hill, 1980; Damuth, 1982; Behrensmeyer et al., 1992). Thus, a legitimate train of logic and experiment connects the comparison of modern ecosystems with depositional settings in the recent past. It should be stressed that such relationships must be interpreted cautiously, and that data from ancient mammalian faunas apply most reasonably at the biome level in well-studied parts of the fossil record.
Another largely independent approach involves the study of ecomorphology. For example, the teeth of mammals are among their most important adaptations, and many features of their energetic and often highly specialized life styles depend to a great extent on their masticatory capabilities. The relationship between tooth morphology and ecology is particularly clear among large herbivores that must adaptively reconcile their long lives and high metabolic activities with relatively poor-quality protein resources (often further complicated by high fiber and high toxin contents). Fortunately, the fossil record of herbivore teeth is excellent and provides a clear history in diverse mammalian taxa of progressive change in their capacity to process increasingly coarse fodder. The dental adaptations of various mammalian herbivores are cited frequently in the following discussion as evidence of the prevailing conditions in North American environments. Such studies were well summarized by Scott (1937).
The most obvious such dental adaptation that many herbivore groups develop is hypsodonty (i.e., high-crowned teeth). A hypsodont animal may be defined as one in which several of its teeth (typically the molars but not uncommonly a whole battery of cheek teeth) have the crown height greater than one dimension of the crown wear surface (length, width, or the average of the two). If one imagines a cubic tooth in an unworn condition it would have a hypsodonty index of one and would be on the threshold of attaining hypsodonty. In modern ungulates and rodents it is not uncommon to find teeth with crown heights many times greater than their crown surface dimension, and some groups develop hypselodonty (i.e., ever-growing teeth).
The adaptations of large mammalian herbivores also contribute strongly to shaping their environments. For example, large mixed herds of grazers facilitate the food resources of one another and also help maintain optimum savanna settings, sometimes by grazing and sometimes by fostering fires (Sinclair and Norton-Griffiths, 1979; McNaughton et al., 1988; Owen-Smith, 1988).
With these interpretive tools in hand we scan the history of land mammal faunas and associated floras to envision the major succession of biomes in North America.
Mammalian Faunal Succession in North America
Paleocene Chronofauna: Tropical Forest
The earliest mammal faunas of the North American Cenozoic consisted of diverse small to medium-sized arboreal and scansorial forms. Most were frugivorous, omnivorous, or insectivorous; specialized carnivores and herbivores of larger body sizes came somewhat later. Four orders, Multituberculata, Insectivora, Primates, and Condylarthra, held over from the Cretaceous, comprise almost the entire Paleocene fauna. Analysis of virtually complete skeletal remains of the multituberculate genus Ptilodus demonstrated that its arboreal adaptations converged closely on those of a tree squirrel (Jenkins and Krause, 1983). The Condylarthra doubled and redoubled their generic numbers every few million years, and soon accounted for several distinct families including some moderately large herbivores (Van Valen, 1978). The distant forerunners of Carnivora (sometimes placed in their own extinct order, Creodonta) appeared by mid-Paleocene. By Late Paleocene, three relatively rare orders of larger mammals immigrated from Asia: the Pantodonta were partly amphibious molluscivores; the Taeniodonta were precociously hypsodont herbivores; and the Dinocerata were the first horned, browsing, herd animals of the Cenozoic. The Torrejonian mammal age included seven immigrant genera if one combines Torrejonian stages 2 and 3, as registered by Stucky (1990).
Predominant environments of the Paleocene land mammals were multistratal evergreen forests and cypress swamps extending north to at least 70°N latitude (Wolfe, 1985; Wing and Tiffney, 1987). During the Tiffanian (ca. 63 Ma), cooler climates—evidenced by increased percentage of deciduous trees and decreased diversity—produced "dramatically lower species richness and evenness" (Rose, 1981, p. 386), presumably because there were less reliable vegetational resources on a year-round basis. Krause and Maas (1990) introduced an enlarged data set on this interval that may weaken this conclusion.
The cooler climate and lower mammal diversity persisted into the Clarkforkian. Small mammals that had previously predominated became relatively rare, while larger forms such as phenacodont condylarths and carnivorous mammals increased in abundance. More importantly, the beginning of the Clarkforkian registers a first-order immigration episode, including Asiatic origins of the extinct order Tillodontia, the modern order Rodentia, and the pantodont family Coryphodontidae (Rose, 1981; Krause and Maas, 1990). Nine immigrant genera reached North America, if one combines Stucky's (1990) records for units 2 and 3. Although the Clarkforkian records the earliest first-order immigration episode in the history of North American land mammals, it is soon superseded by an even larger episode.
Eocene Chronofauna: Subtropical Forest
The wave of immigrations that began in the Clarkforkian intensified in the earliest Eocene or Wasatchian land mammal age. Rose (1981, p. 379) commented as follows:
The most striking aspect of the Wasatchian assemblages is their domination by several taxa that were unknown or exceedingly rare before the Wasatchian. Most of these taxa appeared for the first time in North America at or near the beginning of the Wasatchian and rapidly became the most common members of the fauna. . . . They include the first known members of the orders Perissodactyla (Hyracotherium) and Artiodactyla (Diacodexis), the primate families Adapidae (Pelycodus) and Omomyidae, and the creodont family Hyaenodontidae.
Land mammal immigrants of the early Wasatchian (see Figure 11.3 and Table 11.2) constitute the largest immigration cohort in the North American record. In Wasatchian 1 alone Stucky (1990) records 10 immigrant genera. Krause and Maas (1990, p. 90) more cautiously acknowledge that "many of the early Wasatchian first appearances appear to be immigrants from other continents." Mammals evidently crossed the North Atlantic freely in both directions via the Thulean bridge. According to Savage and Russell (1983), at least 50% of mammalian genera in the Sparnacian of Europe were shared with the Rocky Mountain region, by far the closest degree of transatlantic resemblance that occurred at any time during the Cenozoic.
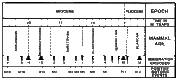
Figure 11.3
Land mammal immigrations in the Miocene of North America, including first-order (triangles) as well as second- and third-order (rectangles) episodes, juxtaposed with δ18O positive excursions as numbered by Miller et al. (1991) based on detailed (more...)
TABLE 11.2
First-Order Immigration Episodes of North American Land Mammal Genera.
The early stages of the Wasatchian are well constrained by stratigraphic studies in the Bighorn Basin of Wyoming. There the base of the earliest Wastachian lies within the reversed magnetic interval below stratigraphic anomaly 24. For this reason, its age lies between 56 and 57 Ma (Butler et al., 1991).
Climatic conditions inferred from faunas and floras of this time suggest that warm equable conditions had returned during the Early Eocene (Rose, 1981; Wolfe, 1985). Primates and other arboreal mammals reach peak richness and abundance by the Middle Eocene, declining thereafter (Stucky, 1990). Rodents and Perissodactyla diversify profoundly, each accounting for about 20% of known mid-Eocene species (Savage and Russell, 1983). Even within the Arctic Circle on Ellesmere Island, diverse arboreal, frugivorous mammals, such as prosimian primates and dermopterans (frugivores distantly related to Asiatic flying foxes), persist along with a subtropical flora (West and Dawson, 1978).
White River Chronofauna: Woodland Savanna
In the continental record for North America a major faunal and environmental shift took place within the Late Eocene (late Duchesnean). Chronometric control on this episode needs further refinement, but it lies between 37 and 42 Ma, and so on an interim basis we call it 40 Ma. For a full discussion of the problem, see Krishtalka et al. (1987).
In the Middle Eocene the first indications of seasonal aridity appeared in the Rocky Mountain region. The great lakes of the Green River region, such as Lake Gosiute, formed extensive evaporites and were encroached on by deeply oxidized redbed deposits. By the Late Eocene, woodland savanna had become the predominant biome in the midcontinent (Webb, 1977; Wing and Tiffney, 1987). The dramatic shift from subtropical forest to predominantly woodland savanna in the Rocky Mountain region soon led to major faunal changes. It is unfortunate that this important turnover interval is not well documented by continuously fossiliferous sedimentary sequences.
The Duchesnean land mammal age is marked by a major faunal turnover episode, including the last appearances of such archaic groups as Condylarthra, Tillodontia, and Dinocerata. More important are the arrivals of the first eubrontothere (Duchesneodus) and several more modern taxa evidently dispersed from Asia (Emry, 1981; Krishtalka et al., 1987). Stucky (1990) recognizes a total of nine genera in Duchesnean 1 and 2. After the Duchesnean the number of browsing herbivore genera rose from 8 to about 40 (Stucky, 1990), and the species numbers of all herbivores cited in Savage and Russell (1983) rose from less than 40 to about 90 during the Eocene-Oligocene transition and remained at this level throughout the Oligocene (Webb, 1989). Emry (1981) labeled this persistent mammalian fauna of the Late Eocene and Oligocene the "White River chronofauna."
The Duchesnean immigration was preceded by a number of other immigration events apparently spread through the Uintan. Unfortunately this interval is about 6 m.y. long, and particular first appearance data are not tightly correlated. We arbitrarily place a second-order episode near the mid-Uintan. The following modern mammal families appear in North America during the Late Eocene: soricid insectivores; sciurid, castorid, cricetid, and heteromyid rodents; leporid lagomorphs; canid and mustelid carnivores; and ungulates-camelids, tayassuids, and rhinocerotids. Several of the newly appearing groups can be shown to have entered North America from Asia, among them the rabbits (Mytonolagus), the amynodont rhinocerotids, and most of the selenodont artiodactyls including camelids, hypertragulids, leptomerycids, and oreodonts (Webb, 1977; Webb and Taylor, 1980; Emry, 1981).
Most of the Late Eocene immigrant herbivores are characterized by adaptations for masticating coarse fodder. At least some members of the following groups developed hypsodont dentitions during the Late Eocene: taeniodonts, leporids, castorids, eomyids, rhinocerotids, hypertragulids, oromerycids, and "oreodonts" (Webb, 1977). For example, Wood (1980, p. 38) characterized the cheek teeth of the eomyid genus Paradjidaumo as ". . . more hypsodont and progressively more lophodont than in Adjidaumo." Also, although most oromerycids are brachydont, Prothero (1986, p. 461) observed that in the new genus Montanatylopus "the molars are much more hypsodont than in any other oromerycid." Thus it is fair to recognize the Late Eocene and Oligocene White River chronofauna as the first in North America to sustain a substantial diversity of hypsodont herbivorous mammals.
The larger mammalian herbivores may be divided into two broadly distinct habitat groups: one group lived primarily along watercourses; the other lived mainly on the interfluves. The flat-skulled leptaucheniine "oreodonts" and the trunk-bearing amynodont rhinos were short-legged, semiamphibious forms that cropped lush vegetation in or near stream courses (Scott, 1937; Wall, 1982). On the other hand, most selenodont artiodactyls, as well as the horse Mesohippus, had relatively long slender limbs and ranged widely in open habitats. Several studies of faunal facies in the White River deposits, reviewed in Webb (1977), provided direct statistical evidence that selenodont artiodactyls and rabbits occurred predominantly in open-country or upland habitats.
Several of the White River ungulates, notably Leptomeryx, ranged together in herds (Clark et al., 1967). The adaptive relationships among social behavior, body size, and feeding mode, developed by Estes (1974), Jarman (1974), and others on the basis of the modern African ungulate fauna, suggest that the appropriate comparison for leptomerycids and other selenodonts is with moderate-sized herding forms such as the gazelles (Jarman's category C). Such forms are mixed feeders, relying on grasses only in their most nutritious new-growth stages and shifting to browsing in the dry season (Janis, 1982).
A third adaptive zone, that of the small burrowing herbivore (rhizovore) and insectivore, is extensively occupied during the White River chronofauna. This implies extensive development of well-drained soils supporting shrubby vegetation. At the same time the diversity of arboreal mammal genera declines (Webb, 1977; Stucky, 1990).
With such mammalian evidence in mind, one may look for other indications that savannas were opening the landscape of the Late Eocene and Oligocene. Hutchison (1982) recognized the severe impact of increasing aridity and seasonality on the aquatic reptile fauna during that interval in the Rocky Mountain region, and in Early Oligocene floras of North America, notably the Florissant in Colorado, the dramatic decrease in the percentage of entire-margined leaves indicates approximately a 10°C drop to about 12.5°C mean annual temperature (MacGinitie, 1962; Wolfe, 1985). Retallack's (1983) pedological studies of White River sediments provide a fascinating look at local paleosols underlying various habitats, and hint at an overall trend toward increasingly grassy and shrubby environments following the Early Oligocene climatic deterioration.
In attempting to understand ecosystems of the Eocene-Oligocene, one may ask which plants the abundant browsing and mixed-feeding herbivores might have eaten. Since grass is still rare in the Eocene-Oligocene, it may be necessary to postulate an emphasis on woody shrubs such as Sarcobatus, Ephedra, and Equisetum. Huber (1982) postulates that just such herb-dominated savannas precede an abundance of true grass savannas in Tertiary floristic history. On the other hand, grass was present and may be underrepresented due to taphonomic conditions in paleofloral sites. Grasses were established in the mid-Eocene of Australia (Truswell and Harris, 1982, cited in Wolfe, 1985) and Europe (Litke, 1968). On present evidence, Wolfe (1985, p. 364) recognized ''. . . a distinctive Eocene savanna (i.e., shrubs and widely spaced trees but no grass on the interfluves)." The extent of grasses within this chaparral and woodland savanna setting remains in doubt.
The quantum jump in mixed-feeding herbivores noted for the White River chronofauna probably helped expand the extent of woodland savanna. The new fauna and flora probably formed a positive feedback loop: large herbivores preferred feeding in more open woodlands; in turn, expansion of open formations facilitated evolution of mixed-feeding herbivores. Among the subtle changes within the history of that chronofauna ("chronofaunal creep") was a decline in the rich array of browsers (e.g., loss of titanotheres after the Chadronian), and an increase in the body sizes and numbers of shrub eaters (quasi-grazers). Such changes indicate an increasing percentage of open-country patches, and tend to be corroborated by the limited botanical and pedological data.
Runningwater Chronofauna: Transitional Savanna
The next major immigration episode in the record of North American mammals takes place in the Early Miocene. Miocene immigration patterns in North American mammals were thoroughly revised by Tedford et al. (1987). Their analysis recognizes six immigrant genera in the latest Arikareean and 14 genera in the earliest Hemingfordian (20 Ma). Native members characteristic of the Runningwater chronofauna of the late Arikareean and early Hemingfordian include flat-incisored beavers (notably Palaeocastor with its deep corkscrew-shaped burrows), hypsodont oreodonts such as Merychyus and Merycochoerus, oxydactyline and protolabine camels (especially Michenia), and the mixed-feeding horse, Parahippus. Several immigrant groups also constitute a vital and characteristic part of the Runningwater chronofauna, notably the primitive bear, Phoberocyon; such genera of amphicyonids (an extinct family also known as "bear-dogs") as Cynelos and Amphicyon; raccoons including Edaphocyon and Amphictis; and several mustelid carnivores including Leptarctus. The first representatives of three ruminant families arrived from Asia at this time: Blastomeryx represents the Moschidae (family of the living Chinese musk deer); Paracosoryx is the scion of the autochthonous North American family Antilocapridae; and Barbouromeryx establishes the presence of the giraffe-like family Palaeomerycidae. Each of these immigrant ruminant groups evolves and diversifies in the course of the Miocene.
The paleoecology of Arikaree assemblages in the midcontinent is thoroughly analyzed by Hunt (1990). Earlier in the Arikareean, widespread eolian distribution of tuffaceous sediments in a seasonally arid climate played an important role in preserving rich samples of mammals in burrows and other nontransported settings. Both the mammalian taxa and the sedimentological evidence indicate ". . . well vegetated stream border communities, spatially separated by open interchannel reaches occupied by grassland, or possibly low shrub savanna or open savanna-parkland environments" (Hunt, 1990, p. 107).
Sheep Creek Chronofauna: Park Savanna
The trend toward more open country habitats continued from the Early into the Middle Miocene at least in the midcontinent. Another substantial wave of immigrants from Asia altered the makeup of the North American mammal fauna and spurred the course of its evolution. It is awkward from a nomenclatural view that this turnover episode falls within the Hemingfordian land mammal age and is not very far removed in time from the previous turnover episode. Tedford et al. (1987) comment as follows:
It is important to note that an important faunal discontinuity exists within the Hemingfordian, signaling an abrupt shift in the Great Plains from the chronofauna characteristic of the late Arikareean and early Hemingfordian to one typifying the late Hemingfordian and early Barstovian . . . . The differences between the faunas of the Box Butte through Sheep Creek interval and the chronofaunally related younger faunas here referred to the Barstovian (Observation Quarry through Lower Snake Creek) can be ascribed mainly to anagenetic change in persistent lineages, making it necessary to distinguish these ages at the specific rather than the generic level.
Major advances within the autochthonous fauna of Hemingfordian age indicate continued evolution toward more open-country adaptations. The best example is the transition from Parahippus, a browsing horse, to Merychippus, an early grazer (Hulbert and MacFadden, 1991). Similar trends are suggested by the increased hypsodonty and quantum increase in diversity of pronghorn antelopes.
The mid-Hemingfordian wave of immigrants from Asia, like the preceding episode, represents a broad ecological spectrum. Among the diverse rodents are flying squirrels of Old World (petauristine) type, eomyid rodents, and the very significant cricetid rodent, Copemys. New carnivores include weasel-like and otter-like mustelids, while the first true cats in the New World are represented by the genus Pseudaelurus (see Table 11.2). Immigration of four very large mammals had a major impact on the Middle Miocene ecosystem: these were two rhinocerotids (short-legged, aquatic Teleoceras and long-legged, terrestrial Aphelops) and also two proboscideans (the browsing mammutid Miomastodon and the probable mixed-feeding gomphotheriid, Gomphotherium). It is now generally supposed that such "megaherbivores" played a formative role in modifying the landscape, as do elephants in modern savanna ecosystems (Owen-Smith, 1988). Detailed evidence on this point, however, has not been developed in the Miocene of North America. The mid-Hemingfordian immigration episode took place about 18 to 17 Ma and was the last major episode until the latest Miocene, a span of about 12 m.y. (Tedford et al., 1989).
Clarendonian Chronofauna: Grassland Savanna
Land mammal diversity in North America reached its zenith during the Barstovian mammal age (Webb, 1989). Savage and Russell (1983) recognized 16 families with 60 genera and 141 nominal species of land mammals in the Barstovian. The next highest numbers occurred in the Clarendonian (the next mammal age), with 55 genera and 117 species. These mammal ages are thought to indicate a savanna optimum in North America, with a rich mosaic of trees, shrubs, and grasses supporting an extraordinary variety of large and small, grazing and browsing, ungulates. It is not uncommon during this savanna acme to collect in a single site 20 genera of ungulates of which half are Equidae (Webb, 1983a; Voorhies, 1990). Savage and Russell (1983, p. 300) noted that during this interval "the mammalian fauna . . . appears singularly homogeneous throughout its geographic range." The Clarendonian chronofauna spanned three mammal ages, an interval of more than 10 m.y. (Tedford et al., 1987).
The array of ungulates in the Late Miocene of North America is comparable in many respects to that living in Africa today. The resemblance is purely convergent: the two faunas have no genera in common, and members of the few shared families play substantially different roles. For example, the body form and paleoecological context of Teleoceras, the most distinctive rhinocerotid perossodactyl in the Clarendonian chronofauna, indicate a niche analogous to that of Hippopotamus (an artiodactyl) in Africa. Voorhies and Thomasson (1979) confirmed by direct evidence from the hyoid (throat) bones that Teleoceras consumed grasses and, from the biocenosis in pond deposits at Poison Ivy Quarry, that herds of young and old frequented water. Likewise in North America the role of the African giraffe is played by the native giraffe-camel, Aepycamelus (Webb, 1983a). The most plausible explanation for these convergences would seem to be that the North American fauna evolved in a broadly comparable manner because of environmental conditions similar to those in Africa, namely, grassland savanna.
Webb (1983a) and Janis (1984) independently attempted comparisons between North American Miocene and African Recent ungulate faunas, with emphases on body size and height (or volume) of cheek teeth. They found similar numbers of ungulate species distributed as browsers, roughage feeders, and mixed feeders. A rough estimate of biomass distribution among these categories, based on quarry censuses from major North American Miocene sites, also gave results that were remarkably similar to actual census data from African game reserves: browsers accounted for less than 10% of all ungulate biomass, whereas roughage feeders (with high hypsodonty indices) made up some 60 to 80% of the total. In a related study, Hulbert (1982) showed that population structure in a Clarendonian species of the grazing horse Neohipparion indicates that it underwent seasonal migrations, an essential feature of the strategy of African grazing ungulates.
African ecological studies show that savanna biotas live where annual rainfall ranges between about 400 and 1000 mm and that, within this range, biomass is positively correlated with rainfall (Coe et al., 1976). Furthermore the number of ungulate species and the relative abundance of larger-bodied species interact with and are roughly correlated with primary productivity (McNaughton et al., 1988). These general observations in Africa shed light on mechanisms governing species richness and size-frequency distribution of ungulates in Miocene local faunas of midcontinental North America before and after the savanna optimum in the Barstovian. In midcontinental North America the Late Miocene ungulate diversity decline presumably tracks a decrease in mean annual rainfall.
Floristic evidence supports the faunal evidence of widespread grassland savanna in the Middle Miocene of North America and increasingly arid climate in the Late Miocene. The Tehachapi flora of Hemingfordian age in California, the Stewart Valley flora of Barstovian age in Nevada, and the Kilgore flora of Barstovian age in Nebraska each has distinctive regional features, yet together they justify the conclusion (Wolfe, 1985, p. 371) that "during the Early to Middle Miocene, savanna developed in low latitude areas that are presently dry." Studies of as many as 18 species of fossil grasses directly associated with mid-Hemphillian ungulates in the Minium Quarry in Kansas also strongly indicate a grassland savanna biome (Thomasson, 1986; Thomasson et al., 1990). In the same floras are leaves with stomata and other detailed features indicative of the subfamily Chloridoideae and of C4 photosynthesis characteristic of tropical floras growing at an optimum temperature of 30 to 32°C (Thomasson et al., 1986). Such floristic studies suggest that the Late Miocene climatic regime in midcontinental North America resembled that of seasonally arid subtropical savannas in South and Central America today.
Early Pliocene: Spread of Steppe
About 5 Ma, at the end of the Miocene, the long-term trend toward increasing aridity in midlatitudes led to the final breakup of the extensive North American savanna biome and its replacement in midcontinental North America by extensive grasslands or true steppe. Ungulates experienced a series of decimations that spanned the Hemphillian; and the first major decline (at about 12 Ma) affected browsers more than grazers (Webb, 1983a, 1984; see also Figure 11.4). By the end of the Hemphillian the number of ungulate genera was cut to less than half of its former peak. No new chronofauna was established, but a major immigration episode coincided with the final late Hemphillian extinctions.
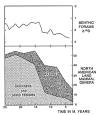
Figure 11.4
Decline of ungulate genera in Miocene of North America (after Webb, 1983a) compared with Miocene record of δ18O based on benthic foraminifera (after Miller et al., 1987). Note that the major Middle Miocene cooling episode coincides with the major (more...)
Floristic changes at the end of the Miocene clearly record the expansion of grasslands in midcontinental and western North America. For example, Wolfe (1985, p. 371) notes that in the Hemphillian of Kansas "the fructifications include abundant grass, and the leaf-assemblages are the low-diversity type that typically occurs along streams in unforested grassland regions." In western Wyoming, Barnosky (1984) recognizes xeric shrub floras during the latest Miocene. The Mt. Eden flora in southern California suggests the beginning of steppe conditions (Wolfe, 1985). Axelrod's (1992) floristic review shows that in the Great Basin and the midcontinent, the most arid interval of the Tertiary occurred at about 6 Ma. Renewed Cordilleran tectonics during the Miocene-Pliocene greatly increased rain shadow effects and provincialism in many regions of temperate North America.
The vast herds of ungulates themselves probably accelerated the decline of savanna and the expansion of steppe in the Pliocene. In Africa during extended droughts, elephants and/or equids devastatingly transform savanna to steppe (Sinclair, 1983; Owen-Smith, 1988). In North America, many late Hemphillian and Blancan fossil quarries (e.g., the Hagerman Horse Quarry in Idaho) yield abundant samples of only one or two grazing equids. These suggest (but do not demonstrate) scenes of massive overgrazing. A positive feedback loop between faunal and floral evolution may have accelerated the process that established steppe conditions in the Early Pliocene.
It should be noted that the Early Pliocene expansion of steppe environments did not encompass all of North America. Provincialism is far more evident than in the Miocene. In the Pacific Northwest, faunal and floral evidence clearly indicates continuation of a mesic forest biome although it interdigitated with semiarid savannas in the Great Basin (Tedford and Gustafson, 1974). The biota around the Gulf of Mexico also indicates the continuation there of savanna conditions (Webb, 1989). The remarkable resemblances between such Hemphillian faunas as the Yepomera local fauna in Chihuahua, Mexico, and the Bone Valley fauna in Florida give evidence of a broad subtropical savanna south of latitude 30°N, which was perpetuated by the persistence of summer monsoons (Webb, 1977). Thus, the Pliocene shift to steppe biomes in the midcontinent produced greater provincialism among diverse regions of North America.
Onto such a scene of regional desiccation and increased provincialism burst a new wave of Asiatic immigrants. As indicated below (Table 11.2), these immigrations appear to be concentrated in the latest Hemphillian, about 5 Ma. Key groups of land mammals that crossed the Bering at this time were vole-like rodents, both terrestrial and aquatic (Repenning, 1987); at least six carnivores, including exotic groups such as hyaenids (Chasmaporthetes) and pandas (Parailurus), as well as more familiar groups such as Lynx and Ursus; and also at least two genera of deer, which gave rise to a substantial radiation in North and South America.
Late Pliocene and Pleistocene: Further Continentality and Provincialism
The secular trend of the Cenozoic toward colder, drier, and in other ways more extreme conditions led to the environments of the later Pliocene and Pleistocene. As the first Laurentide glaciation settled over North America about 2.5 Ma, forest tundra biomes became widespread in the Arctic Circle (Funder et al. 1985; Repenning, 1985). Mountain building in the Cordillera extended tundra corridors along high-elevation routes into temperate latitudes. Rain shadows helped extend cold-steppe habitats into many basins of western North America. In the Snake River Plain (as represented by the Hagerman fauna and flora), there were still broad-leaved deciduous forests. To the north and at higher elevations, however, conifers predominated (Lundelius et al., 1987). The southern plains (as represented by the Mt. Blanco fauna and the Rita Blanca flora) were characterized by a seasonally arid climate that produced grassy scrubland and an impoverished ungulate fauna dominated by grazers. Widespread caliche deposits in Late Pliocene deposits of the Great Plains have long been recognized as indicators of seasonal aridity (Hibbard and Taylor, 1960).
In Florida and presumably the rest of the southeastern United States, vertebrate faunas of late Blancan age carry a number of "holdover taxa"—such as the peccary, Mylohyus; the protoceratid, Kyptoceras; and the three-toed horse, Cormohipparion—which were already extinct in the midcontinent. The ecological balance of these holdovers suggests persistence of seasonal savannas and extensive mesophytic forests in warmer, more productive settings than in the High Plains (Robertson, 1976; Webb, 1978; Hulbert, 1987). In general, the Late Pliocene array of biomes in North America approximated the degree of provincialism seen at present, although the extremes of freezing winters and arid deserts were absent.
In the Late Pliocene (Blancan 2) the first large wave of immigrants from South America appeared, including glyptodonts, chlamytheres, armadillos, ground sloths, capybaras, and porcupines. At the same time, another large wave of immigrants came from Asia; among them were the spectacled bear (Tremarctos) and several rodents (Synaptomys, Pliopotamys, and Mictomys) (Lundelius et al., 1987). This final immigration episode of the Tertiary coincided with expansion of the Bering bridge. It clearly correlates with the onset of Northern Hemisphere glaciation, sea-level lowering, and evidence of pre-Nebraskan till in western Iowa (Lundelius et al., 1987; Repenning, 1987).
The late Hemphillian and early Blancan immigration episodes just considered adumbrate an increasingly active series of Quaternary immigration episodes. High latitude Quaternary studies on both sides of the Beringia land bridge record trends among microtine rodents, as well as many large mammals, such as mammoths and musk oxen, toward steppe-tundra adaptations (Sher, 1974; Repenning, 1985; Herman, 1989). The complex pattern of faunal dynamics among Quaternary mammals is well summarized by Tedford et al. (1987, p. 192): "The accelerating pace of immigration from Hemphillian into Pleistocene time reflects the availability of dispersal routes to North America and increasing environmental instability, particularly at high latitudes, that provided the goad for the movement of mammals."
Results
We analyzed the record of immigrations in successive North American land mammal faunas, as a possible signal of major environmental changes in the history of that continent. We selected this continent because it has the most complete Cenozoic record of terrestrial mammalian history. We tallied the number of land mammal genera that apparently immigrated in each of 52 informal subdivisions of 19 land mammal ages following the biostratigraphic scheme presented in Woodburne (1987). In this review, we have not dealt with other diversity changes due to extinctions and endemic radiations. We found that the two largest episodes (Wasatchian 1 and Hemingfordian 1) involved 15 and 14 genera, respectively; five other episodes also involved nine or more genera (Table 11.1). We arbitrarily designate these seven largest as first-order immigration episodes. We categorize another cluster of seven episodes, consisting of five or seven genera each, as second-order episodes. All smaller counts were considered third-order or "background" immigration patterns. The numbers of genera in first-order and second-order episodes are documented in Table 11.1. The only third-order episodes we were able to tally accurately are the five from the Miocene. The genera counted in the seven first-order immigration episodes are listed in taxonomic order in Table 11.2.
TABLE 11.1
Land Mammal Immigration Episodes.
An unexpected result of this analysis is the paired pattern among six of the seven first-order immigration episodes. Except for the Duchesnean, each first-order episode occurs within about two million years of another first-order episode. The three pairs are Clarkforkian with Wasatchian, Hemingfordian 1 and 2, and Hemphillian 3 and Blancan 2. The first-order status of the Duchesnean immigration episode may be artifactual. This interval, and the preceding Uintan, span about 10 m.y., much of it represented by sparsely fossiliferous sediments. The nine immigrant genera recognized here are thought to be clustered in the late Duchesnean (Emry, 1981; Krishtalka et al., 1987), but this may well be an artifact of a relatively impoverished record. If one examines the distribution of first- and second-order episodes throughout the Tertiary, one notes a tendency for clustering of several episodes, notably in the Early Miocene, when the Arikareean associates with the two Hemingfordian episodes, and in the Late Miocene where a string of three second-order episodes is associated with the final pair of first-order episodes.
Discussion
During the past decade, mammalian paleontologists have discovered, somewhat to their collective surprise, that the pulse of the Cenozoic succession in North America is strongly syncopated. In particular, publication of a detailed mammalian biochronology for North America (Woodburne, 1987) underlined the unevenness of faunal turnover. The rhythm of long-stable chronofaunal intervals punctuated by rapid turnover episodes has emerged as a clear pattern (Vrba, 1985a; Webb, 1989). At the level of a continental ecosystem this pattern may be referred to as "syncopated equilibrium." The need now is for closer analysis of rapid turnover episodes to gather new insights into the mechanisms and modalities that translate environmental change into radical reorganization of terrestrial ecosystems.
In the following discussion we address the question of what "trigger mechanism" might bring about the first-order immigration episodes that we have tallied above. An unexpected result of this analysis is the close association of the six first-order immigration episodes into three pairs. This is not conceivably a random pattern. These three pairs of immigration episodes pack a majority of North American immigrants into a time frame equal to only about one-tenth of the Cenozoic. Perhaps the second episode in each pair represents delayed discovery, due to poor recovery of rare fossils during early phases of colonization. Thus, the second immigration wave could be an "echo" of the first. After careful review of the stratigraphic and geographic details of each instance, we find this hypothesis unlikely, although it is worthy of more rigorous analysis. We are left with the straightforward view that the physical and ecological conditions that triggered the first of each pair (or cluster) of major immigration episodes were reiterated within one or two million years, thus bringing about paired and clustered sets of immigration episodes. If correct, this pattern provides an important clue as to the sustained (or recurrent) force of whatever conditions "triggered" the immigration episodes into North America.
In exploring possible causal mechanisms for immigration and rapid turnover episodes on the North American continent, we turned to two other large bodies of correlative evidence. First, we scanned the record of land mammal faunal turnover on other continents in search of corroborative evidence. Secondly, we looked at the marine record of global climatic change (as represented by oxygen isotope signals) for correlative patterns during the Cenozoic.
Mammal Turnover on Other Continents
As the land mammal successions on other continents become more continuously documented and their chronologies become more fully correlated with the absolute time scale, it is important to determine whether they respond synchronously with the immigration and rapid turnover episodes observed in the North American record. We summarize a few highlights from other continental mammal faunas in Europe, China, the Indian subcontinent, and Africa. South America and Australia were almost completely isolated during most of the Tertiary; therefore, their land mammal faunas need not be involved in this immigration analysis.
European Land Mammal Record
The European record of Cenozoic land mammals closely approaches that of North America both in chronological continuity and in taxonomic diversity. Unfortunately, no complete revision of European mammal invasions is available. Mein (1989) lists many generic first appearances for Neogene mammal ages, but the lists do not distinguish between allochthonous and autochthonous first appearances. Such distinctions are more challenging in western Europe than in North America because it is simply a portion of Eurasia and the proportion of immigrant genera in successive faunas is probably much greater in Europe than in North America. Thus we are unable to derive an authoritative analysis of immigrants in the European record to compare with that for North America.
Opdyke (1990) recognized a strong correlation between Neogene faunal turnover episodes on the two continents, using a somewhat indirect approach. Since the land mammal ages were established independently in Europe and North America, on the basis of empirical biostratigraphy, he compared the biochronological boundaries. Synchronous boundaries indicate synchronous pulses of faunal turnover, which must be governed by global climatic events. If, on the other hand, the mammalian turnover (and immigration) episodes were independent and governed only by local effects, there should be little or no correlation between the two systems. As Opdyke (1990) suggests, there is a remarkable degree of correspondence between the two independently derived continental land mammal chronologies during the Neogene.
The status of land mammal ages in Europe was recently reviewed by Lindsay et al. (1990). Although the methods of mammal age definition differ considerably from those used in North America (Woodburne, 1987), the succession of mammal ages on the two continents appears nearly isochronous from Early Miocene through Late Pliocene. We highlight some of the more favorable comparisons below.
Legendre (1986) indicates three major immigration episodes among Paleogene land mammals of Europe. First is the strong continuity with North America in the earliest Eocene; this has long been recognized as the time of arrival of modern orders such as Primates, Perissodactyls, and Artiodactyls. The second is a small immigration wave in the Lutetian about 45 Ma. The third and most important is the rapid turnover episode long known as the "Grande Coupure," at approximately 32 to 34 Ma. What began mainly as an extinction event, led soon to a modernized fauna, characterized for example by new families of perissodactyls. The landscape in western Europe shifted from predominantly rain forest to savanna during this Early Oligocene transition (Legendre, 1986).
In the European Neogene system, the beginning of the Orleanian (MN 4) is marked by the immigration of the browsing horse, Anchitherium; two genera of proboscidea from Africa; and at least five genera of ruminants, possibly from Africa (Mein, 1989; Ginsburg, 1989; Tassy, 1989).
Although Anchitherium came from North America, it reached Europe by way of Asia. Early Orleanian correlates with lower Hemingfordian and with the first-order immigration episode at about 20 Ma in North America.
The Vallesian-Astaracian boundary has been the subject of controversy, and that controversy is entwined with evidence regarding the first appearance of Hipparion and other hipparionine horses from North America via Asia. The genus Hipparion appears on the margins of the Mediterranean at 11.5 Ma in Chron C5R, which would make the base of the Vallesian at least this old. This date correlates precisely with the base of the Clarendonian assigned by Tedford et al. (1987) on the basis of North American biostratigraphy and many radiometric dates. Few immigrants, however, appear in North America at that time.
The base of the Turolian is marked by many immigrant appearances such as the rabbit, Alilepus, and the proboscidean, Anancus, and is well dated at 9 Ma (Berggren et al., 1985; Mein, 1989). This date coincides with the beginning of the Hemphillian in the North American record and with the second-order immigration episode here recognized as Hemphillian 1 (Table 11.2).
The beginning of the European Ruscinian mammal age correlates with the latest Hemphillian in North America (Tedford et al., 1987). The Ruscinian mammal age, approximating the Miocene-Pliocene boundary, is now well dated in the Mediterranean area, where that sea refilled. Ruscinian is defined by the many new appearances that occurred as a consequence of the Messinian sea-level cycle and the severe climatic deterioration that accompanied it. In North America, we recognize Hemphillian 3 as a first-order immigration episode, correlated with the Messinian sea-level cycle (Webb, 1983b).
The early Villafranchian is marked in Europe by a major turnover episode including the arrival of Equus there (as well as in Asia), accompanied by the appearance of Elephas, various large deer, and also as Azzaroli (1989, p. 341) notes, "the demise of a forest assemblage characterized by Tapirus, Sus, and Ursus." This episode may be correlated with the first-order immigration episode known as Blancan 2 in North America. Presumably these equivalent events were driven by the same climatic mechanisms, namely, the onset of Northern Hemisphere glaciation.
By the Pleistocene, North America and Europe lack any meaningful correspondence between their respective mammal ages. Possibly the frequency of faunal migrations and climatic shifts after the Gauss Chron will require a much more refined system than the present broadly defined mammal ages. Repenning (1987) and others have found much finer-tuned rodent zonations to be quite useful in relating mammal faunal turnover to global climatic changes in the Quaternary.
Chinese Land Mammal Record
Correlations of the rich land mammal succession in China are rapidly improving and already can test, in some instances, the hypothesis of global synchroneity of rapid faunal change episodes among land mammals. Current studies of Oligocene faunas in China suggest that Asia may have been the source of many immigrants in the European Grande Coupure after the marine seaway retreated from the Turgai Straits. In his review of Neogene mammalian biochronology in China, Qiu (1989) recognizes four major immigration episodes that alter the character of the Asian fauna. First, the nearly simultaneous immigration of Anchitherium from North America and Gomphotherium from Africa marks a major change in the Early Miocene fauna. Qiu (1989) also notes the brachyerycine insectivores as another trans-Beringian immigrant group that appears in the Early Miocene of China.
The second major Miocene immigration episode from outside Eurasia was the appearance of Hipparion from North America. The importance of the Hipparion datum is widely recognized throughout Eurasia and may occur at about 12 Ma.
Thirdly, the Gaozhuang fauna of Miocene-Pliocene age in the Yushe Basin is under intensive study and represents one of the best understood and best-dated immigration episodes in Asia. Among key immigrants are an arvicoline rodent Germanomys; the rabbit Hypolagus; the canid Nyctereutes; the pig Sus; and the camelid Paracamelus (Qiu, 1989; Tedford et al., 1989). There is a fairly close correlation between the Gaozhuang fauna in China and the Ruscinian in Europe.
Finally, the Late Pliocene immigration episode in China closely corresponds to that in Europe, key immigrations being those of Elephas from Africa and Equus from North America. As in North America, this episode occurs at about 2.5 Ma near the base of the Matuyama Chron (Qiu,
1989). Ding et al. (1992) show that Hipparion-bearing red clays were transitional to the loess sequence in which these immigrants appear and that these events were coupled with major uplift of the Himalayan Plateau.
Indian Land Mammal Record
One of the best-studied mammal-bearing sequences in the world is that of the Siwalik Hills of Pakistan and India. Long, nearly continuous sections, extensively exposed and richly fossiliferous, yield a virtually complete magnetostratigraphic column for the Miocene. It is quite interesting, therefore, that Barry and Flynn (1989, p. 567) attribute the pulse of faunal turnover in that region primarily to immigration episodes triggered by land bridges; they summarize that relationship as follows:
In the Siwaliks in situ evolution appears to occur in only a few lineages and is therefore thought to be unimportant in most groups. Immigration and extinction events tend to be correlated and together were the principal cause of faunal change. As immigration events often precede extinctions, and in some cases can be inferred to have caused them, immigration and the resulting ecological disruption may have been the primary cause of community change. Many of the immigrant species probably originated in Africa, Europe, or other parts of Asia. Faunal turnovers are thus also intervals of faunal exchange and indicate times when land connections were established. Nearly all of these episodes show approximate correlations to global climatic, oceanographic, and tectonic events, and these through their effects on sea-level, intercontinental connections, and vegetation, may have controlled movement of mammals into the Siwalik province.
The earliest major immigration episode had already occurred before the first Neogene mammalian fauna is recorded in the Siwalik Hills, and led to the establishment of bovids and other ruminants (the dominant large herbivores and muroid rodents). Barry et al. (1985) estimate that this first episode occurred between 18 and 22 Ma, and Barry and Flynn (1989) correlate it approximately with the TB 2.1 sea-level fall of Haq et al. (1988). This episode coincides with similar (first-order) episodes of mammal immigration between Eurasia and North America, and between Europe and Africa.
A second major immigration episode (between 15 and 13 Ma) involving muroid rodents and ruminant artiodactyls was accompanied by an abrupt rise in species numbers (Barry et al., 1991). This coincides approximately with the high diversity "savanna optimum" in North America, but does not correlate with any major immigration episode in North America.
The next major faunal turnover episode observed in the Siwalik mammal succession occurs at 9.5 Ma when hipparionine horses appear and murids become dominant over cricetid rodents. Barry et al. (1985) suggested that this probably represents one of the major Middle Miocene isotopic and sea-level events discussed by Barron (1985).
A major climatic shift toward increasing aridity and seasonality took place between 7.5 and 7.0 Ma, and caused a major turnover in Siwalik faunas, notably the replacement of large hominoids by cercopithecoid monkeys. The pattern of increased aridity, however, was time transgressive, so that contemporaneous faunas 300 miles to the southeast retained a more tropical woodland aspect (Barry and Flynn, 1989). Unfortunately, the Siwalik record does not adequately record the major faunal turnover episodes at the end of the Miocene.
African Land Mammal Record
In Africa, much of the Cenozoic record of mammalian immigrations and faunal turnover episodes is interrupted by too many hiatuses to represent a coherent record of immigration episodes. Nevertheless, during selected intervals, Africa provides an exceedingly valuable sample of a continent notable for being the evolutionary cradle of such important mammalian groups as proboscideans, ruminants, and hominoids. At the beginning of the Neogene, for example, Savage (1989, p. 592) recognizes in the Early Miocene at Gebel Zelten in Libya " . . . a tropical regime with open shrubland faunas . . . communicating across Tethys with Eurasia." Among the mammal groups with shared genera of about 20 Ma (Orleanian age of the European land-mammal scheme) are proboscidea, creodonts, carnviores, rhinoceroses, suids, and bovids (Vrba, 1985b).
Particularly significant immigration episodes occurred in Africa at about 5.0 and 2.5 Ma. At the former time, apparent immigrants are the antelope groups (bovid subfamilies) Ovibovini, Bovini, and probably Reduncini; at the latter time, caprines (goats) and the genus Oryx constitute key immigrant groups (Vrba, 1985b).
Coincidence of first-order immigration and rapid turnover episodes across several continents adds great force to the significance of North American episodes. At present, however, such generalizations can be developed securely only for three intervals in the Neogene. In absolute terms, these times are about 20, 5, and 2.5 Ma. These three well-corroborated rapid turnover episodes correspond to the following first-order immigration episodes in North America: Hemingfordian 1, Hemphillian 3, and Blancan 2.
Oxygen Isotopes and Mammal Immigrations
In an influential paper, Fischer (1983) introduced the concept of two Phanerozoic supercycles in which the Earth's climate has alternated between a greenhouse state and an icehouse state. In the Cenozoic the Earth has experienced its most recent change from greenhouse to icehouse. The broad outlines of this change are well known and have been revealed by shifts in the oxygen isotope ratios extracted from tests of foraminifera in marine sediment cores. The Cenozoic record for benthic foraminifera clearly displays a secular trend to more positive isotopic values, reflecting major cooling of bottom waters in the world ocean. There is general agreement that in the Early Eocene, δ18O values were zero or negative; that these values became more positive throughout the Eocene; and that they declined sharply in latest Eocene, and again in Middle Miocene and latest Miocene.
Another independent approach to the history of Cenozoic climates derives from seismic stratigraphic studies of passive continental margins. Haq et al. (1988) provided a recent summary of the apparent eustatic curve compiled from many well-dated seismic stratigraphic studies. The Cenozoic is represented by parts A and B of the Tejas megasequence and includes seven second-order supercycles. Each of these supercycles spans about 10 Ma and terminates at a sequence boundary of major magnitude. Shackleton et al. (1988), Williams (1988), Christie-Blick et al. (1990), and Miller et al. (1991) have carefully considered the relationships between isotopic and seismic studies as they bear on the history of Cenozoic climate. Potential pitfalls for the seismic method are confounding the effects of local sedimentary load or local tectonism with more global eustatic effects. For these reasons, we focus on the isotopic record as the more reliable signal of global climatic change during the Cenozoic. It should be noted, however, that for many intervals, there is substantial correlation between the seismic and isotopic approaches.
Shackleton and Opdyke (1977) showed that during the late Pleistocene, oxygen isotope ratios covaried between tropical planktic and benthonic foraminifera. They interpreted the simultaneous changes in surface and bottom-dwelling foraminifera as evidence of growth and decay of ice sheets on the continents. Therefore, the δ18O record represents change in both ocean temperature and ice volume. The problem has been to partition these two effects. Miller et al. (1991) recently extended the covarying isotopic studies to sediments of mid-Tertiary age, recognizing key intervals of ice buildup by covariant increases in heavy oxygen ratios in Miocene and even Oligocene cores.
Prentice and Matthews (1988) have attempted to monitor Cenozoic sea-level change by analyzing oxygen isotope ratios in planktic foraminifera from equatorial regions. The efficacy of this approach depends on the assumption that equatorial sea-surface temperatures (away from upwellings) have not changed during the Cenozoic. Thus, they reason that observed isotopic changes wholly reflect waxing and waning of glacial ice. Figure 11.2 juxtaposes North American land mammal immigration episodes with the trace of Cenozoic oxygen isotope ratios based on planktic forams from equatorial regions. We discuss each of the continental first-order immigration episodes in relation to the marine record.

Figure 11.2
First- and second-order land mammal immigration episodes in the Cenozoic of North America juxtaposed with the marine record of δ18O from equatorial planktic foraminifera after Prentice and Matthews (1988). If sea-surface temperatures have not (more...)
The earliest pair of first-order immigration episodes are the Clarkforkian and early Wasatchian, straddling the Paleocene-Eocene boundary. Although they fall generally within the warmest climatic interval of the Cenozoic, they correlate with small cooling events in the isotope curve at about 59 and 56 Ma. The Clarkforkian immigration episode correlates particularly well with the abrupt cooling episode at the end of the Paleocene (59 Ma), demonstrated by Kennett and Stott (Chapter 5, this volume) in high-resolution data based on planktonic forams from the southern ocean. Miller et al. (1987) postulated a sea-level drop at this time, and Haq et al. (1988) recognized a second-order drop in sea-level (TA 222-24) in the Thanetian.
The very large Wasatchian land mammal immigration episode reflects plate tectonic effects in the North Atlantic that established a very broad, low latitude corridor across the Thulean route and thus produced extremely close faunal resemblance between North America and Europe as discussed above.
The next first-order episode occurs in the early Duchesnean (Late Eocene) at about 40 Ma (Emry, 1981; Krishtalka et al., 1987). This episode corresponds well to a number of global Late Eocene events. Miller et al. (1987) show a major oxygen isotope increase at about 40 Ma based on benthic forams, and this event is also seen in planktonic forams from equatorial cores presented by Prentice and Matthews (1988). These isotopic events correlate with the beginning of the major sea-level drop TA4 (Priabonian) of Haq et al. (1988). According to Hallam (1984) and others cited therein, this latest Eocene sea-level drop is greater than that of the Late Oligocene. The profound global climatic shift of the Late Eocene correlates with a strong increase in the Earth's thermal gradient due to cooling in the southern ocean (Kennett and Barker, 1990).
During the Neogene, land mammal immigration episodes increased markedly in North America. Because of their importance and frequency, these Miocene and Pliocene immigrations were subjected to detailed analysis by Tedford et al. (1987). In Figure 11.3, we juxtapose the full array of Neogene land mammal immigration episodes (including third-order episodes) with the δ18O excursions numbered by Miller et al. (1991). As suggested by Opdyke (1990) the episodes correlate remarkably closely with oxygen isotope events in the marine record. Only one isotope event (namely Miocene 3) fails to correlate with an immigration episode in North America.
On the other hand, several immigration episodes in the Miocene of North America fail to correlate with any of the positive isotope excursion numbered by Miller et al. (1991). One first-order immigration episode (Arikareean 2 at 20 Ma) fails this correspondence test; as noted below it does correlate with a small (unnumbered) positive isotope excursion. Blancan 1 (at 4.8 Ma) also appears to be unrequited, but in fact it may correspond with Pliocene 1 of Miller et al. (1991). Other lesser immigration episodes at 14.5, 7.0, and 6.0 Ma do not correspond to numbered isotope excursions.
The Early Miocene records the largest set of generic immigrations in the history of the North American land mammal fauna. Three land mammal dispersal episodes fall near the boundary between Arikareean and Hemingfordian: they begin with a second-order episode at 21 Ma followed by first-order episodes at 20 and 18 Ma. The episodes at 21 and 18 Ma coincide with isotope events Mi1b and Mi1c of Miller et al. (1991). The immigration episode at 20 Ma corresponds to a positive isotope excursion in both benthic and planktonic forams as identified by Prentice and Matthews (1988). According to the latter, sea-level at 20 Ma reached depths not attained again until the Pleistocene. Miller et al. (1987) show a possible erosional interval at 20-21 Ma, and Haq et al. (1988) record a first-order drop in the seismic curve between 20 and 21 Ma (TB2). On the other hand, the episode at 18 Ma does not correspond to any major sea-level drop noted in the seismic curve.
Early Miocene land mammal immigrants, including three groups of ruminants, participated in the transition to savanna biomes in midcontinental North America, accompanied by a shift to seasonally arid climates and loess deposition, as discussed above. Presumably this Early Miocene savanna transformation was driven by a fundamental change of state in the global climatic pattern, most notably intensified deep water flow with opening of the Drake Passage and concomitant development of the Antarctic Circumpolar Current (Kennett and Barker, 1990).
Further major shifts are evident in the Middle Miocene, but in North America, these do not include significant numbers of immigrants. Instead, the acme of land mammal diversity, dominated by horses and other savanna herbivores, is attained in the Barstovian. This savanna optimum on the continent correlates with the largest Middle Miocene shift in the Neogene δ18O record. The strong string of positive isotope shifts in the marine record is widely believed to represent the buildup of permanent glaciers on East Antarctica (Kennett and Barker, 1990). Haq et al. (1988) record a major sea-level drop at 16 Ma with additional drops at 14 and 12 Ma.
In Figure 11.4, we juxtapose the Neogene portion of the benthic foram isotope record after Miller et al. (1987) with the detailed record of large herbivore diversity and feeding modes in North America. The latter record, more fully discussed by Webb (1983a), shows the acme of herbivore diversity in the Barstovian, followed by a steep stepwise decline during the Clarendonian and early Hemphillian. The losses affected primarily browsing ungulates and, secondarily, grazing ungulates. Environmentally, these Middle and Late Miocene faunal changes represent the shift from savanna to steppe biomes, presumably a result of declining rainfall. A remarkably similar transition occurs concurrently in the land mammals of the Indian subcontinent: from the herbivore acme in woodland savanna environments, a stepwise decline takes place that also represents a shift toward more arid environments between 16 and 12 Ma (Barry et al., 1991). The Middle Miocene patterns on land and sea strongly support an interpretation of climatic forcing (driven by attainment of permanent glaciation in East Antarctica) of land mammal faunal evolution in temperate latitudes.
In the Late Miocene of North America, beginning with the Clarendonian/Hemphillian boundary at about 8.5 Ma, three second-order immigration episodes occur. The episode at 8 Ma coincides with isotope event Mi7 of Miller et al. (1991) and roughly approximates the drop in sea-level recognized as TB3.2 of Haq et al. (1988). Of the two further migratory episodes at roughly 7 and 6 Ma, only the latter corresponds to a reported marine event, namely that shown by Miller et al. (1991) at 5.8 Ma. Kennett and Barker (1990) suggest that the later Miocene was the interval during which West Antarctica became permanently glaciated.
The Miocene-Pliocene boundary is associated with a first-order immigration episode in land mammal faunas of both North America and Europe. This episode correlates well with a positive shift in δ18O (Hodell et al., 1986), but does not seem to correlate with any episode of sea-level lowering in the Haq et al. (1988) curve. Possibly sea-level is beginning to fluctuate at high frequency in the Late Miocene and Pliocene, with the consequence that it becomes difficult to define any particular pulse with seismic stratigraphy. On the other hand, the Messinian is well known in the Mediterranean region and in the isotopic record as a major glacioeustatic cycle (Adams et al., 1977; Kennett, 1985; Hodell et al., 1986). As emphasized by Webb (1983b), the Messinian can be tightly correlated with the mid-Hemphillian extinction and faunal turnover episode in temperate North America.
The last second-order episode of the Tertiary sweeps through land mammal faunas on most continents at 2.5 Ma in the Late Pliocene. Such major immigrations are clearly related to the onset of Northern Hemisphere glaciation recognized in the δ18O record and also indicated by glacial sediments (Ruddiman and Raymo, 1988; Shackleton et al., 1988; Raymo and Ruddiman, 1992). All of these features correlate with marked cooling and sea-level lowering indicated by the oxygen isotope record (Shackleton and Opdyke, 1977).
Generally speaking, land mammal immigration episodes in North America and other well-studied continental areas correlate with positive excursion of the oxygen isotope record from tropical marine forams. Other continental regions clearly corroborate the significant turnovers recorded at about 20, 5, and 2.5 Ma.
In some cases, however, the terrestrial turnover pattern gives no evident response to major cooling events. The two most glaring examples are the Early Oligocene (White River chronofauna) and the Middle Miocene (Clarendonian chronofauna) in North America when, despite major global changes, the mammal faunas show remarkable stability and continuity. Possibly no land bridges were available during the Early Oligocene. In the Middle Miocene, this excuse cannot serve, because a few Asiatic immigrants did trickle in and Beringia was emergent. Evidently, during these two stable chronofaunal stages the North American mammalian fauna was resilient and not open to immigrants.
Conclusions
The rich record of Cenozoic land mammals in North America documents a large-scale succession of terrestrial ecosystems. In the midcontinent the predominant biomes changed from subtropical forest in the Eocene through woodland savanna in the Oligocene, to park savanna and then grassland savanna in the Miocene, to steppe in the Pliocene, when increased provincialism became evident. This broad march of faunal change has been inferred not only from dental adaptations and body-size distributions in the mammalian faunas, but also from independent lines of terrestrial evidence including floral succession, sedimentology, and stable isotopes. Clearly the continental biota in North America broadly tracks the global climatic trends of the Cenozoic.
More specific faunal analyses of the well-calibrated North American record reveal a remarkably irregular progression of faunal change. Immigration of land mammals from Europe, Asia, and South America, totaling some 140 genera in the course of the Cenozoic, are concentrated mainly in seven first-order episodes. Pairs of first-order episodes bracketing 57, 19, and 4 Ma suggest that a major interval favoring immigration somehow produced an ''echo" effect. The largest of all immigration sets occurred in the Early Miocene when two first-order episodes and one second-order episode arrived between 21 and 18 Ma.
Land mammal records from other continents tend to replicate strong mammal immigration episodes at 20, 5, and 2.5 Ma. Other resemblances include a Middle Miocene acme in the Siwaliks, possibly correlated with the Barstovian acme in North America, but this interval has a notably low rate of intercontinental immigrations.
Causal considerations lead to two possible hypotheses linking mammal immigrations to patterns of global climatic change. The first hypothesis postulates greater availability of land bridges due to low sea-levels, given the correlation between positive oxygen-isotope excursions and eustatic drops in the marine record. The second, more purely terrestrial hypothesis links each first-order immigration episode to wholesale reorganization of the continental ecosystem triggered by climatic shifts.
Two major contradictions to the first hypothesis appear in the Oligocene (35 to 30 Ma) and the Middle Miocene (16 to 6 Ma). Despite major global cooling events, the North American mammal fauna admitted very few immigrants. Possibly no land bridges were available at those times, although the appearance of a few immigrants in the Middle Miocene suggests that there was some physical access. More probably, there were ecological barriers to immigrants at these times. During these two intervals the land mammal faunas experienced high diversity and long stable community development (chronofaunal evolution). This lends credence to the view that the ecosystem was near capacity during the Barstovian acme and perhaps during other chronofaunal intervals. Other more disturbed intervals, on the other hand, were open to major immigration episodes that coincided with positive isotopic excursions in the marine realm.
Evidently first-order changes in the oxygen isotope record are necessary but not sufficient causes of first-order immigration episodes into the North American land mammal fauna. The record of continental mammal faunas itself offers a strong signal of major global climatic change. The question of why the continental ecosystem was open to immigrations during certain positive isotopic excursions and not others has fundamental significance to our understanding of the stability of present continental ecosystems. It therefore demands further investigation.
Acknowledgments
We have benefited from discussions with Catherine Badgely, John Barry, David Hodell, Everett Lindsay, Bruce MacFadden, Malcolm McKenna, Ken Miller, Nick Shackleton, Richard Tedford, Elizabeth Vrba, and Michael Woodburne. This research was partly supported by grant number BSR 89 18065 to S.D.W. from the National Science Foundation. This is contribution number 431 in Paleobiology from the Florida Museum of Natural History.
References
- Adams, C. G., R. H. Benson, R. B. Kidd, W. B. F. Ryan, and R. C. Wright (1977). The Messinian salinity crisis and evidence of Late Miocene eustatic changes in the world ocean, Nature 269 , 383-386.
- Andrews, P., J. M. Lord, and E. M. Newsbit Evans (1979). Patterns of ecological diversity in fossil and modern mammalian faunas, Biol. Jour. Linnean Soc. 11 , 177-205.
- Axelrod, D. I. (1992). Climatic pulses, A major factor in legume evolution, in Advances in Legume Systematic: The Fossil Record ( part 4 ), P. S. Herendeen, editor; and D. L. Dilcher, editor. , eds., Royal Botanical Gardens, Kew, London, pp.259-279.
- Azzaroli, A. (1989). The genus Equus in Europe, in European Neogene Mammal Chronology , Plenum Press, New York, pp.339-356.
- Barnosky, C. W. (1984). Late Miocene vegetational and climatic variations inferred from a pollen record in northwest Wyoming, Science 223 , 49-51. [PubMed: 17752988]
- Barron, E. J. (1985). Explanations of the Tertiary global cooling trend, Palaeogeography, Palaeoclimatology, Palaeoecology 50 , 45-61.
- Barry, J. C., and L. J. Flynn (1989). Key biostratigraphic events in the Siwalik sequence, in European Neogene Mammal Chronology , E. H. Lindsay, editor; , V. Fahlbusch, editor; , and P. Mein, editor. , eds., Plenum Press, New York, pp.557-572.
- Barry, J. C., N. M. Johnson, S. M. Raza, and L. L. Jacobs (1985). Neogene mammalian faunal change in southern Asia: Correlations with climatic, tectonic, and eustatic events, Geology 13 , 637-640.
- Barry, J. C., L. J. Flynn, and D. R. Pilbeam (1991). Faunal diversity and turnover in a Miocene terrestrial sequence, in Causes of Evolution: A Paleontological Perspective , R. Ross, editor; and W. Allmon, editor. , eds., University of Chicago Press, Chicago.
- Behrensmeyer, A. K., and A. P. Hill (1980). Fossils in the Making: Vertebrate Taphonomy and Paleoecology , University of Chicago Press, Chicago.
- Behrensmeyer, A. K., J. D. Damuth, W. A. DiMichele, R. Potts, H.-D. Sues, and S. L. Wing (1992). Terrestrial Ecosystems Through Time , University of Chicago Press, Chicago, 568 pp.
- Berggren, W. A., D. V. Kent, J. J. Flynn, and J. A. Van Couvering (1985). Cenozoic Geochronology, Geological Society of America Bulletin 96 , 1407-1418.
- Butler, R. F., P. D. Gingerich, and E. H. Lindsay (1991). Magnetic polarity, stratigraphy and biostratigraphy of Paleocene and lower Eocene continental deposits, Clark's Ford Basin, Wyoming, Journal of Geology 89 , 299-316.
- Christie-Blick, N., G. S. Mountain, and K. G. Miller (1990). Seismic stratigraphic record of sea-level change, in Sea-Level Change , Studies in Geophysics, National Research Council, National Academy Press, Washington, D.C., pp.116-140.
- Clark, J., J. R. Beerbower, and K. K. Kietzke (1967). Oligocene sedimentation, stratigraphy, paleoecology and paleoclimatology in the Big Badlands of South Dakota, Fieldiana , Geology Memoirs 5, 1-158.
- Coe, M. J., D. H. Cumming, and J. Phillipson (1976). Biomass and production of large African herbivores in relation to rainfall and primary production, Oecologia 22 , 341-354. [PubMed: 28308896]
- Damuth, J. (1982). Analysis of the preservation of community structure in assemblages of fossil mammals, Paleobiology 8 , 434-446.
- Ding, Z., N. Rutter, H. Jingtai, and L. Tungsheng (1992). A coupled environmental system formed at about 2.5 Ma in East Asia, Palaeogeography, Palaeoclimatology, Palaeoecology 94 , 223-242.
- Emry, R. J. (1981). Additions to the mammalian fauna of the type Duchesnean, with comments on the status of the Duchesnean "age," Journal of Paleontology 55 , 563-570.
- Estes, R. D. (1974). Social organisation of the African Bovidae, in Behavior of Ungulates in Relation to Management , V. Geist, editor; and F. Walther, editor. , eds., I.U.C.N., Morges, Switzerland.
- Fischer, A. G. (1983). The two Phanerozoic supercycles, in Catastrophes and Earth History , W. A. Berggren, editor; and J. A. Van Couvering, editor. , eds., Princeton University Press, Princeton, N.J., pp.129-150.
- Funder, S., N. Abrahamsen, P. Bennike, and R. W. Feyling-Hannsen (1985). Forested Arctic: Evidence from North Greenland, Geology 13 , 542-546.
- Gingerich, P. D. (1984). Pleistocene extinctions in the context of origination-extinction equilibria in Cenozoic mammals, in Quaternary Extinctions , P. S. Martin, editor; and R. G. Klein, editor. , eds., University of Arizona Press, Tucson, pp.211-222.
- Gingerich, P. D. (1989). New earliest Wasatchian mammalian fauna from the Eocene of northwestern Wyoming: Composition and diversity in a rarely sampled high-floodplain assemblage, University of Michigan Papers in Paleontology 28 , 197.
- Ginsburg, L. (1989). The faunas and stratigraphical subdivisions of the Orleanian in the Loire Basin (France), in European Neogene Mammal Chronology , E. H. Lindsay, editor; , V. Fahlbusch, editor; , and P. Mein, editor. , eds., Plenum Press, New York, pp.157-176.
- Hallam, A. (1984). Pre-Quaternary sea-level changes, Annual Reviews of Earth and Planetary Sciences 12 , 205-243.
- Haq, B. U., J. Hardenbol, and P. R. Vail (1988). Mesozoic and Cenozoic chronostratigraphy and eustatic cycles, in Sea-Level Changes: An Integrated Approach , Society of Economic Paleontologists and Mineralogists Special Publication 42, pp.71-108.
- Herman, Y., editor. , ed. (1989). The Arctic Seas: Climatology, Oceanography, Geology and Biology , Van Nostrand Reinhold, New York, 888 pp.
- Hibbard, C. W., and D. W. Taylor (1960). Two late Pleistocene faunas from southwestern Kansas, University of Michigan Museum of Paleontology Contribution 16 , 1-223.
- Hodell, D. A., K. M. Elmstron, and J. P. Kennett (1986). Latest Miocene benthic δ18O changes, global ice volume, sea-level and the "Messinian salinity crisis," Nature 320 , 411-414.
- Huber, O. (1982). Significance of savanna vegetation in the Amazon Territory of Venezuela, in Biological Diversification in the Tropics , G. T. Prance, editor. , ed., Columbia University Press, New York, pp.221-224.
- Hulbert, R. C., Jr. (1982). Population ecology of Neohipparion (Mammalia: Equidae) from the Late Miocene Love Bone Bed of Florida, Paleobiology 8 , 159-167.
- Hulbert, R. C., Jr. (1987). A new Cormohipparion (Mammalia, Equidae) from the Pliocene (latest Hemphillian and Blancan) of Florida, Journal of Vertebrate Paleontology 7 , 451-468.
- Hulbert, R. C., Jr., and B. J. MacFadden (1991). Morphological transformation and cladogenesis at the base of the adaptive radiation of Miocene hypsodont horses, Amer. Mus. Novitates .
- Hunt, R. M., Jr. (1990). Taphonomy and sedimentology of Arikaree (lower Miocene) fluvial, eolian, and lacustrine paleoenvironments, Nebraska and Wyoming: A paleobiota entombed in fine-grained volcaniclastic rocks, in Volcanism and Fossil Biotas , M. G. Lockley, editor; and A. Rice, editor. , eds., Geological Society of America Special Paper 244, pp.69-111.
- Hutchison, J. H. (1982). Turtle, crocodilian, and champsosaur diversity changes in the Cenozoic of the north-central region of western United States, Palaeogeography, Palaeoclimatology, Palaeoecology 37 , 149-164.
- Janis, C. (1982). Evolution of horns in ungulates: Ecology and paleoecology, Biol. Rev. 57 , 261-318.
- Janis, C. (1984). The use of fossil ungulate communities as indicators of climate and environment, in Fossils and Climate , P. Brenchley, editor. , ed., John Wiley & Sons, New York, pp.85-104.
- Jarman, P. J. (1974). The social organisation of antelope in relation to their ecology, Behavior 48 , 215-267.
- Jenkins, F. A., Jr., and D. W. Krause (1983). Adaptations for climbing in North American Multituberculates (Mammalia), Science 220 , 712-715. [PubMed: 17813876]
- Kennett, J. P., editor. , ed. (1985). The Miocene Ocean: Paleoceanography and Biogeography , Geological Society of America Memoir 163.
- Kennett, J. P., and P. F. Barker (1990). Latest Cretaceous to Cenozoic climate and oceanographic developments in the Weddell Sea, Antarctica: An ocean-drilling perspective, in Proceedings of the Ocean Drilling Program 113 , P. F. Barker, editor; , J. P. Kennett, editor. et al., eds., College Station, Texas, pp.937-960.
- Kowalevsky, W. (1873). Sur l'Anchitherium aurelianense Cuvier et sur l'Histoire paleontologique des Chevaux, Mem. de l'Acad. Imperiale des Sciences St. Petersburg, 7e ser. 20(5) , 1-73 .
- Krause, D. W., and M. C. Maas (1990). The biogeographic origins of Late Paleocene-Early Eocene mammalian immigrants to the Western Interior of North America, in Dawn of the Age of Mammals in the Northern Part of the Rocky Mountain Interior , T. M. Brown, editor; and K. D. Rose, editor. , eds., Geological Society of America Special Paper 243, pp.71-105.
- Krishtalka, L., R. M. West, C. C. Black, M. R. Dawson, J. J. Flynn, W. D. Turnbull, R. K. Studky, M. C. McKenna, T. M. Bown, D. J. Golz, and J. A. Lillegraven (1987). Eocene (Wasatchian through Duchesnean) biochronology of North America, in Cenozoic Mammals of North America: Geochronology and Biostratigraphy , M. O. Woodburne, editor. , ed., University of California Press, Berkeley and Los Angeles.
- Legendre, S. (1986). Analysis of mammalian communities from the Late Eocene and Oligocene of southern France, Palaeovertebrata 16 , 191-212.
- Lindsay, E. H., N. D. Opdyke, and N. M. Johnson. (1984). Blancan-Hemphillian land mammal ages and late Cenozoic mammal dispersal events, Annual Review Earth and Planetary Science 12 , 445-488.
- Lindsay, E. H., editor; , V. Fahlbusch, editor; , and P. Mein, editor. , eds. (1990). European Neogene Mammal Chronology , Plenum Press, New York, 658 pp.
- Litke, R. (1968). Uber den nachweis tertiarer Gramineen, Monatsber. Deut. Akad. Wiss. Berlin 19 , 462-471.
- Lundelius, E. L., Jr., T. Downs, E. H. Lindsay, H. A. Semken, R. J. Zakrzewski, C. S. Churcher, C. R. Harington, G. E. Schultz, and S, D. Webb (1987). The North American Quaternary sequence, in Cenozoic Mammals of North America: Geochronology and Biostratigraphy , M. O. Woodburne, editor. , ed., University of California Press, Berkeley and Los Angeles.
- MacGinitie, H. D. (1962). The Kilgore flora: A Late Miocene flora from northern Nebraska, University of California Publications in Geological Sciences 35 , 67-158.
- McNaughton, S. J., and N. J. Georgiadis. (1986). Ecology of African grazing and browsing mammals, Annual Review of Ecological Systems 17 , 39-65.
- McNaughton, S. J., R. W. Ruess, and S. W. Seagle (1988). Large mammals and process dynamics in African ecosystems, Bioscience 38 , 794-800.
- Mein, P. (1989). Updating of MN zones, in European Neogene Mammal Chronology , E. H. Lindsay, editor; , V. Fahlbusch, editor; , and P. Mein, editor. , eds., Plenum Press, New York, pp.73-90.
- Miller, K. G., R. G. Fairbanks, and G. S. Mountain. (1987). Tertiary oxygen isotope synthesis, sea-level history, and continental margin erosion, Paleoceanography 2 , 1-19.
- Miller, K. G., J. D. Wright, and R. G. Fairbanks (1991). Unlocking the ice house: Oligocene-Miocene oxygen isotopes, eustasy, and margin erosion, Journal of Geophysical Research 96(B4), 6829-6848.
- Olson, E. C. (1952). The evolution of a Permian vertebrate chronofauna, Evolution 6, 181-196.
- Olson, E. C. (1983). Coevolution or coadaptation? Permo-Carboniferous vertebrate chronofauna, in Coevolution , M. H. Nitecki, editor. , ed., University of Chicago Press, Chicago, pp.307-338.
- Opdyke, N. D. (1990). Magnetic stratigraphy of Cenozoic terrestrial sediments and mammalian dispersal, Journal of Geology 98 , 621-637.
- Owen-Smith, R. N. (1988). Megaherbivores: The Influence of Very Large Body Size on Ecology , Cambridge University Press, Cambridge, 369 pp.
- Prentice, M. L., and R. K. Matthews. (1988). Cenozoic ice-volume history: Development of a composite oxygen isotope record, Geology 16 , 963-66.
- Prothero, D. R. (1986). A new Oromerycid (Mammalia, Artiodactyla) from the Early Oligocene of Montana, Journal of Paleontology 60 , 458-465.
- Qiu, Z. (1989). The Chinese Neogene mammalian biochronology: Its correlation with the European Neogene mammalian zonation, in European Neogene Mammal Chronology , E. H. Lindsay, editor; , V. Fahlbusch, editor; , and P. Mein, editor. , eds., Plenum Press, New York, pp.527-556.
- Raymo, M. E., and W. F. Ruddiman (1992). Tectonic forcing of late Cenozoic climate, Nature 359 , 117-122.
- Repenning, C. A. (1985). Pleistocene mammalian faunas: Climate and evolution, Acta Zool. Fennica 170 , 173-176.
- Repenning, C. A. (1987). Biochronology of the microtine rodents of the United States, in Cenozoic Mammals of North America: Geochronology and Biostratigraphy , M. O. Woodburne, editor. , ed., University of California Press, Berkeley and Los Angeles.
- Retallack, G. J. (1983). A paleopedological approach to the interpretation of terrestrial sedimentary rocks: The mid-Tertiary fossil soils of Badlands National Park, South Dakota, Geological Society of America Bulletin 94 , 823-840.
- Robertson, J. R. (1976). Latest Pliocene mammals from Haile XV A, Alachua Co., Florida, Bulletin of the Florida State Museum, Biological Sciences 20 , 111-186.
- Rose, K. D. (1981). The Clarkforkian land mammal age and mammalian faunal composition across the Paleocene-Eocene boundary, University of Michigan Papers in Paleontology 26 , 1-196.
- Rowley, D. B., and A. L. Lottes (1988). Plate-kinematic reconstructions of the North Atlantic and Arctic Late Jurassic to Present, Tectonophysics 155 , 73-120.
- Ruddiman, W. F., and M. E. Raymo (1988). Northern Hemisphere climate regimes during the last three million years: Possible tectonic connections, Philosophical Transactions of the Royal Society of London B318 , 411-430.
- Savage, D. E., and D. E. Russell. (1983). Mammalian Paleofaunas of the World , Addison-Wesley Publishing Co, Reading, Mass.
- Savage, R. J. G. (1989). The African dimension in European Early Miocene faunas, in European Neogene Mammal Chronology , E. H. Lindsay, editor; , V. Fahlbusch, editor; , and P. Mein, editor. , eds., Plenum Press, New York, pp.587-599.
- Scott, W. B. (1937). A History of Land Mammals in the Western Hemisphere , Macmillan, New York.
- Shackleton, N. J., and N. D. Opdyke. (1977). Oxygen isotope and paleomagnetic stratigraphy of equatorial Pacific core V28-239; Oxygen isotope temperatures and ice volumes on a 105-106 year scale, Quaternary Research 3 , 39-55.
- Shackleton, N. J., R. G. West, and D. Q. Bowen (1988). The past three million years: Evolution of climatic variability in the North Atlantic region, Philosophical Transactions of the Royal Society of London B318 , 409-688.
- Sher, A. V. (1974). Pleistocene mammals of the far northeast USSR and North America, International Geology Review 16 , 1-89.
- Sinclair, A. R. E. (1983). The adaptation of African ungulates and their effects on community function, in Tropical Savannas , F. Bourliere, editor. , ed., Elsevier, Amsterdam.
- Sinclair, A. R. E., and M. Norton-Griffiths (1979). Serengeti: Dynamics of an Ecosystem , University of Chicago Press, Chicago and London.
- Stehli, F. G., editor; , and S. W. Webb, editor. , eds. (1985). The Great American Biotic Interchange , Topics in Geobiology, Plenum Press, New York.
- Stucky, R. (1990). Evolution of land mammal diversity in North America during the Cenozoic, Current Mammalogy 2 , 375-432.
- Tassy, P. (1989). The "Proboscidean Datum Event": How many proboscideans and how many events, in European Neogene Mammal Chronology , E. H. Lindsay, editor; , V. Fahlbusch, editor; , and P. Mein, editor. , eds., Plenum Press, New York, pp.237-252.
- Tedford, R. H., and E. Gustafson (1974). First North American record of the extinct Pand, Parailurus, Nature 265 , 621-623.
- Tedford, R. H., M. F. Morris, R. W. Fields, J. M. Rensberger, D. P. Whistler, T. Galusha, B. E. Taylor, J.R. Macdonald, and S. D. Webb (1987). Faunal succession and biochronology of the Arikareean through Hemphillian interval (Late Oligocene through earliest Pliocene Epochs) in North America, in Cenozoic Mammals of North America: Geochronology and Biostratigraphy , M. O. Woodburne, editor. , ed., University of California Press, Berkeley and Los Angeles.
- Tedford, R. H., L. J. Flynn, and Z. Qiu (1989). Neogene faunal succession, Yushe Basin, Shanxi Province, PRC, Journal of Vertebrate Paleontology 9 , 41A.
- Thomasson, J. R. (1986). Tertiary fossil plants found in Nebraska, National Geographic Society Research Report 19 , 553-564.
- Thomasson, J. R., M. E. Nelson, and R. J. Zakrzewski (1986). A fossil grass (Gramineae: Chloridoideae) from the Miocene with Kranz anatomy, Science 233 , 876-878. [PubMed: 17752216]
- Thomasson, J. R., R. J. Zakrsewski, H. E. Lagarry, and D. E. Mergen (1990). A Late Miocene (late early Hemphillian) biota from northwestern Kansas, National Geographic Society Research 6 , 231-244.
- Truswell, E. M., and W. K. Harris (1982). The Cainozoic palaeobotanical record in arid Australia: Fossil evidence for the origins of an arid-adapted flora, in Evolution of the Flora and Fauna of Arid Australia , W. R. Barker, editor; and P. J. M. Greenslade, editor. , eds., Peacock Publications, Frewville, Australia, pp.57-76.
- Van Valen, L. M. (1978). The beginning of the age of mammals, Evolution Theory 4 , 45-80.
- Voorhies, M. R. (1990). Vertebrate Paleontology of the Proposed Norden Reservoir Area, Brown, Cherry and KeyaPaha Counties, Nebraska , Bureau of Reclamation, Denver, Colo., 944 pp.
- Voorhies, M. R., and J. R. Thomasson (1979). Fossil grass anthoecia within Miocene rhinoceros skeletons: Diet in an extinct species, Science 206 , 331-333. [PubMed: 17733681]
- Vrba, E. S. (1985. a). Environment and evolution: Alternative causes of the temporal distribution of evolutionary events, South African Journal of Science 81 , 229-236.
- Vrba, E. S. (1985. b). African bovidae: Evolutionary events since the Miocene, Suid-Arikaanse Tydskrif vir Wetenskap 81 , 263-266.
- Wall, W. P. (1982). Evolution and biogeography of the Amynodontidae (Perissodactyla, Rhinocerotoidea), Third North American Paleontological Convention, Proceedings 2 , 563-567.
- Webb, S. D. (1977). A history of savanna vertebrates in the New World. Part I: North America, Annual Reviews of Ecological Systems 8 , 355-380.
- Webb, S. D. (1983. a). The rise and fall of the Late Miocene ungulate fauna in North America, in Coevolution , M. H. Nitecki, editor. , ed., University of Chicago Press, Chicago, pp.267-306.
- Webb, S. D. (1983. b). On two kinds of rapid faunal turnover, in Catastrophes and Earth History: The New Uniformitarianism , W. A. Berggren, editor; and J. A. Van Couvering, editor. , eds., Princeton University Press, Princeton, N.J., pp.417-436.
- Webb, S. D. (1984). Ten million years of mammal extinctions in North America, in Quaternary Extinctions: A Prehistoric Revolution , P. S. Martin, editor; and R. G. Klein, editor. , eds., University of Arizona Press, Tucson, pp.189-210.
- Webb, S. D. (1989). The fourth dimension in North American terrestrial mammal communities, in Patterns in the Structure of Mammalian Communities , D. W. Morris, editor. et al., eds., Special Publications, Museum of Texas Technical University, pp.181-203.
- Webb, S. D., and B. E. Taylor (1980). The phylogeny of hornless ruminants and a description of the cranium of Archaeomeryx , Bulletin of the American Museum of Natural History 167 , 117-158.
- West, R. M., and M. R. Dawson (1978). Vertebrate paelontology and the Cenozoic history of the North Atlantic region, Polarforschung 48 , 103-119.
- Williams, D. F. (1988). Evidence for and against sea-level changes from the stable isotopic record of the Cenozoic, in Sea-Level Changes: An Integrated Approach , Society of Economic Paleontologists and Mineralogists Special Publication 42, pp.31-38.
- Wing, S. L., and B. H. Tiffney (1987). The reciprocal interaction of angiosperm evolution and tetrapod herbivory, Reviews of Palaeobotany and Palynology 50 , 179-210.
- Wolfe, J. A. (1985). Distribution of major vegetational types during the Tertiary, in The Carbon Cycle and Atmospheric CO 2 Natural Variations Archean to Present , E. T. Sundquist, editor; and W. S. Broecker, editor. , eds., Geophysical Monograph 32, American Geophysical Union, Washington, D.C., pp.357-374.
- Wood, A. E. (1980). The Oligocene rodents of North America, Transactions of the American Philosophical Society 70 , 1-68.
- Woodburne, M. O., editor. , ed. (1987). Cenozoic Mammals of North America: Geochronology and Biostratigraphy , University of California Press, Berkeley and Los Angeles, 336 pp.
Publication Details
Copyright
Publisher
National Academies Press (US), Washington (DC)
NLM Citation
National Research Council (US) Panel on Effects of Past Global Change on Life. Effects of Past Global Change on Life. Washington (DC): National Academies Press (US); 1995. 11, Global Climatic Influence on Cenozoic Land Mammal Faunas.