NCBI Bookshelf. A service of the National Library of Medicine, National Institutes of Health.
National Research Council (US) Panel on Anticholinesterase Chemicals; National Research Council (US) Panel on Anticholinergic Chemicals. Possible Long-Term Health Effects of Short-Term Exposure to Chemical Agents: Volume 1 Anticholinesterases and Anticholinergics. Washington (DC): National Academies Press (US); 1982.
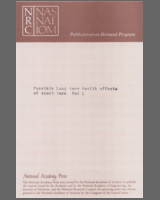
Possible Long-Term Health Effects of Short-Term Exposure to Chemical Agents: Volume 1 Anticholinesterases and Anticholinergics.
Show detailsATROPINE, SCOPOLAMINE, AND RELATED THERAPEUTIC ANTICHOLINERGIC CHEMICALS*
SITES OF ACTION
Anticholinergic drugs inhibit the actions of acetylcholine (ACh) on autonomic effectors that are innervated by postganglionic cholinergic nerves and on smooth muscles that lack cholinergic innervation; that is, they antagonize the muscarinic actions of ACh. They are therefore known as antimuscarinic agents or muscarinic cholinergic blocking agents. Because the main actions of all members of this class of drugs are qualitatively similar to those of the best-known member, atropine, the terms “atropinic” and “atropine-like” are also appropriately used.
In general, antimuscarinic agents have little effect on the actions of ACh at nicotinic receptor sites. Thus, at autonomic ganglia, where transmission normally involves an action of ACh on nicotinic receptors, atropine produces partial block only at relatively high doses. At neuromuscular junctions, where the receptors are nicotinic, extremely high doses of atropine or related drugs are required to cause any degree of blockade. However, quaternary ammonium analogues of atropine and related drugs generally have some degree of nicotinic blocking activity and consequently are more likely to interfere with ganglionic or neuromuscular transmission in doses only slightly greater than those that produce muscarinic block.
In the central nervous system (CNS), cholinergic transmission appears to be predominantly nicotinic in the spinal cord and both muscarinic and nicotinic at subcortical and cortical levels in the brain (2). Accordingly, many or most of the CNS effects of atropine-like drugs at ordinary doses are probably attributable to their central anticholinergic actions. At high or toxic doses, the central effects of atropine and related drugs consist, in general, of stimulation followed by depression; these are probably due to a combination of antimuscarinic and other actions. There is an increased release and turnover of ACh in the CNS associated with the administration of antimuscarinic drugs; this may result in the activation of nicotinic receptors in the brain and contribute to the central effects of this class of drugs (3). Because quaternary compounds penetrate the blood-brain barrier only poorly, antimuscarinic drugs of this type show little in the way of central effects.
PERIPHERAL EFFECTS
Parasympathetic neuroeffector junctions in different organs are not equally sensitive to antimuscarinic agents. However, the relative sensitivity of various parasympathetically innervated organs to blockade by atropinic agents varies little among the drugs. Small doses inhibit salivary and bronchial secretion and sweating. Larger doses cause the pupils to dilate, inhibit accommodation of the eyes, and block vagal effects on the heart, so that the heart rate is increased. Still larger doses inhibit the parasympathetic control of the urinary bladder and gastrointestinal tract, thus inhibiting micturition and decreasing intestinal tone and motility. Even larger doses are required to inhibit gastric secretion and motility. Because only the primary phase of gastric secretion is controlled by the vagus, the remaining hormonally controlled secretion is unaffected. Thus, doses of any antimuscarinic drug that reduce the tone and motility of the stomach and the duodenum and inhibit gastric secretion also invariably affect salivary secretion, ocular accommodation, and micturition.
The drugs produce reversibly the functional equivalent of resection or paralysis of postganglionic cholinergic nerves. The actions and effects of particular antimuscarinic agents usually differ only quantitatively from those of atropine, a belladonna alkaloid, which is considered in detail as the prototype of the group.
CENTRAL EFFECTS
Atropine stimulates the medulla and higher cerebral centers. In doses used clinically (0.5–1.0 mg), this effect is usually confined to mild vagal excitation. The rate and occasionally the depth of breathing are increased, but this effect is probably the result of bronchiolar dilatation and the consequent increase in physiologic “dead space.” With toxic doses of atropine, central excitation becomes more prominent, leading to restlessness, irritability, disorientation, hallucinations, or delirium. With still larger doses, stimulation is followed by depression, coma, and medullary paralysis. The latter may be primarily responsible for a fatal outcome. Even moderate doses of atropine may depress some central motor mechanisms that control muscle tone and movement. This effect has been used to advantage in the management of the tremor and rigidity of Parkinsonism.
Scopolamine in therapeutic doses normally causes drowsiness, euphoria, amnesia, fatigue, and dreamless sleep with a reduction in rapid-eye-movement (REM) sleep. However, the same doses of scopolamine occasionally cause excitement, restlessness, hallucinations, or delirium, especially in the presence of severe pain.
Doses of atropine required to inhibit peripheral responses to choline esters or anticholinesterase (anti-ChE) agents produce almost no detectable central effects. This may reflect difficulty of penetration of the drug into the CNS. In animals, atropine antagonizes the action of ACh applied locally to the cerebral cortex and spinal cord. However, atropine also depresses the effects of noncholinergic stimuli, indicating that the drug has central actions other than blocking cholinergic synapses.
The rise in body temperature due to the belladonna alkaloids is usually significant only after large doses. Nevertheless, in infants and small children, moderate doses induce “atropine fever.” In atropine poisoning in infants, the temperature may reach 43°C or higher. Suppression of sweating is doubtless an important factor in the production of the fever, especially when the environmental temperature is high, but other mechanisms also may be important when large doses are taken. It has been suggested that atropine may exert a central effect on temperature regulation; however, animals that do not sweat, such as dogs, do not exhibit fever after atropine.
POISONING BY BELLADONNA ALKALOIDS
The deliberate, or accidental ingestion of belladonna alkaloids or other drugs with atropinic properties is a major cause of poisonings. Infants and young children are especially susceptible to the toxic effects of atropinic drugs (4). Delirium and toxic psychoses, without undue peripheral manifestations, have been reported in adults after instillation of atropine eyedrops.
Fatalities from intoxication with atropine and scopolamine are rare, but they sometimes occur in children, in whom 10 mg or less may be lethal. Idiosyncratic reactions are more common with scopolamine than with atropine, and ordinary therapeutic doses sometimes cause alarming effects. Table 1 lists undesirable responses or symptoms of overdose associated with various doses of atropine.
TABLE 1
EFFECTS OF ATROPINE IN RELATION TO DOSE.
SYMPTOMS AND SIGNS OF ATROPINE POISONING
Symptoms and signs of atropine poisoning develop promptly after ingestion. The mouth becomes dry and the person experiences a burning sensation; swallowing and talking are difficult or impossible; and there is marked thirst. The vision is blurred, and photophobia is prominent. The skin is hot, dry, and flushed. A rash may appear (more likely in children), especially over the face, neck, and upper part of the trunk; desquamation may follow. Body temperature rises, especially in infants. The pulse is weak and very rapid although tachycardia may be less pronounced in infants and old people. Palpitation is prominent, and blood pressure may be increased. Urinary urgency and difficulty in micturition are sometimes noted. Abdominal distention may develop, especially in infants.
The patient is restless, excited, and confused and exhibits weakness, giddiness, and muscular incoordination. Gait and speech are disturbed. Nausea and vomiting sometimes occur. The behavior and mental signs may suggest an acute organic psychosis. Memory is disturbed, orientation is faulty, hallucinations (especially visual) are common, the sensorium is clouded, and mania and delirium are not unusual (5). The diagnosis of an acute schizophrenic episode or alcoholic delirium has been mistakenly made, and some patients have been committed to psychiatric institutions for observation and diagnosis. The syndrome often lasts 48 h or longer and may be punctuated by convulsions. Depression and circulatory collapse occur only in cases of severe intoxication; blood pressure decreases, respiration becomes inadequate, and death due to respiratory failure follows after a period of paralysis and coma (6).
TREATMENT
When intoxicating doses of atropine have been taken orally, gastric lavage and other measures to limit intestinal absorption should be initiated without delay. Physostigmine, long overlooked as a possible antidote to atropine poisoning, is the rational therapy. For example, the slow intravenous injection of 1–4 mg of physostigmine (0.5–1.0 mg in children) rapidly abolishes the delirium and coma caused by large doses of atropine. Because physostigmine is metabolized rapidly, the patient may again lapse into coma within 1–2 h, and repeated doses may be needed (4,5,7).
MECHANISM OF ACTION
The major action of the antimuscarinic agents is a competitive antagonism of the actions of ACh and other muscarinic agonists. The antagonism can be overcome by increasing sufficiently the concentration of ACh at receptor sites of the effector organ. The receptors affected are those of peripheral structures that are either stimulated or inhibited by muscarine—that is, exocrine glands and smooth and cardiac muscle. Much evidence supports the notion that atropine and related compounds compete with muscarinic agonists for identical binding sites on muscarinic receptors (2,8,9).
ABSORPTION, FATE, AND EXCRETION
The belladonna alkaloids are absorbed rapidly from the gastrointestinal tract. They also enter the circulation when applied locally to mucosal surfaces. Only limited absorption occurs from the intact skin. Atropine disappears rapidly from the blood and is distributed throughout the entire body. Most is excreted in the urine within the first 12 h, in part unchanged. Only about 1% of an oral dose of scopolamine is eliminated as such in the urine, traces of atropine are found in various secretions, including milk. The total absorption of quaternary ammonium derivatives of the alkaloids after an oral dose is only about 10–25 percent (10,11); nevertheless, some of these compounds can cause mydriasis and cycloplegia if applied to the eye.
PHARMACOLOGICAL PROPERTIES OF SYNTHETIC ATROPINE DERIVATIVES
After reports in the late 1950s of the psychotomimetic effects of a number of basic esters of substituted glycolic acids related to scopolamine and atropine, several hundred compounds were synthesized at Edgewood and by its contractors and by academic and industrial researchers. These compounds were intended to be more active centrally than peripherally. Because quaternary salts of these anticholinergic compounds usually penetrate the CNS only with great difficulty, such derivatives were of little interest in the testing program.
The compounds prepared and tested in the Edgewood program are listed in Table 2, and their structures are shown in the master file (Appendix B). The basic structures of these compounds, which are substituted glycolates, are as follows:
Table 2
Summary of Exposures to Anticholinergics and Records Selected for Review of Acute Effects of Anticholinergic Chemicals.

where X=-OH, -CH2OH, -OCH3, -Cl, -Br, -I, -O2CCH3,
R1 and R2=hydrogen, phenyl, substituted phenyl, alkyl, cycloalkyl, ethylenic, olefinic, thienyl, and
R3=acyclic aminoalkyl, cyclic aminoalkyl, bicyclic amino alkyl (e.g., tropine or scopine for atropine and scopolamine, respectively).
Table 3 lists the relative potencies (comparing central with peripheral effects) of many of these derivatives. Note that BZ and EA 3443 exhibit extensive central effects for a long period; the studies at Edgewood emphasized these chemicals, as does this report.
Table 3
Human Pharmacologic Characteristics of 14 Glycolic Acid Esters (Based on Edgewood Arsenal Studies, 1960–1971).
In a comparison of a series of structurally related anticholinergic compounds, there was less discrepancy in potency with respect to peripheral than central actions. Because data on their effects on man are limited, most of the information was derived from animal behavioral studies, which in general predict psychotomimetic actions in man.
BZ and other quinuclidinyl glycolates containing at least one phenyl and a cycloalkyl group in the acid moiety were the most potent and the longest-acting both in animals and in man. The corresponding piperidyl glycolates were one-fifth to one-half as active as the quinuclidinyl esters. Atropine is equipotent (although of shorter duration) to BZ in producing peripheral anticholinergic effects, but its central actions are much less pronounced.
ROLE OF DRUG DISPOSITION IN EXPLAINING DIFFERENCES AMONG ANTICHOLINERGIC COMPOUNDS
ATROPINE
Numerous reports dealing with the disposition of labeled atropine in animals and are reviewed by Wills in Appendix I. Investigators have been only partially successful in their efforts to compare the pharmacologic actions and relative potencies of the various anticholinergic compounds in the CNS with their distribution in brain or their binding to brain components. For instance, BZ has been reported to adsorb to isolated brain mitochondria about 3 times as avidly as atropine (12). The affinity of BZ for peripheral muscarinic receptors was also greater than that of atropine (8), and atropine competed with BZ for specific binding sites in the brain. Because BZ is known to be more potent than atropine and its duration of action is appreciably longer (13), these drug effects could be due to differences in the affinities of the two drugs for specific receptor sites.
BZ
Snyder's group (14,15) used autoradiography to determine precisely the location of binding of [3H]BZ in rat brain. In the cerebral cortex, the greatest binding of the benzilate was in the occipital cortex and the cortex of the cingulate gyrus, with decreasing binding in the cortex in the piriform area and the frontal pole. In the hippocampus, three strata of the archipallium were found to have approximately equal abilities to bind BZ, whereas the white matter of the alveus bound less. The high binding of BZ to the striate body was particularly striking in autoradiographs because of the comparatively low binding activity of the adjoining globus pallidus. Thalamic and hypothalamic nuclei exhibited less binding of BZ, as did the inferior and superior colliculi. Still lower in the binding of this ligand were cerebellar cortex, medulla oblongata-pons, and the cerebral peduncles. Nerve tracts—e.g., the optic chiasma, the cervical spinal cord, and the corona radiata—exhibited the lowest degree of binding.
The areas of the brain that retained the greatest concentrations of the label after intravenous injection of [3H]BZ into cats (16) were motor cortex, sensory cortex, caudate nucleus, lateral geniculate, and medial geniculate. Smaller concentrations were retained in thalamus, hippocampus, hypothalamus, medulla oblongata, colliculi, cerebellar cortex, the pyramids of the medulla, cerebral white matter, and cerebellar white matter.
Both cholinomimetic and anticholinergic compounds reduced the retention of the label after [3H]BZ treatment in some areas of the brain. The cholinomimetic compounds used were 1,2,3,4-tetrahydro-5-aminoacridine (THA) and S-diethylaminoethyl-diethylphosphorothionate, both inhibitors of cholinesterases. For the anticholinergic compounds, displacement of the label probably was related to the substitution of one drug by another.
Zvirblis and Kondritzer (12) found that a mitochondrial fraction prepared from rat brain adsorbed 3–4 times as much BZ as atropine in the physiologic range of pH. The optimal pH for adsorption of BZ was about 8.0, whereas that for atropine was about 9.7; however, at their own optimal pH values, the adsorptions of the two anticholinergic compounds were about the same. THA, Ca2+ and PO4 2− decreased the adsorption of the benzilate by mitochondria.
Yamamura et al. (17) studied the adsorption of [3H]BZ on the particulate fraction of homogenates of various regions of the brain of monkey after incubation with [3H]BZ in phosphate buffer at a pH of 7.4. The regions that were the most avid adsorbers of the benzilate, in order of decreasing uptake, were putamen, caudate nucleus, occipital cortex, cingulate gyrus, postcentral gyrus, pyriform cortex, frontal cortex, superior colliculi, thalamus, and inferior colliculi. This distribution of adsorptive sites among various parts of the monkey brain incubated in vitro differs from that for intravenously injected [3H]BZ in the cat (16). The two studies do agree that the caudate nucleus is one of the highest areas of localization of [3H]BZ. Yamamura et al. (17) found that uptake of the label by various areas of brain was correlated with choline uptake and with choline acetyltransferase activity.
Interruption of the septal hippocampal tract and elimination thereby of cholinergic afferents to the hippocampus reduced by about 70% the activity of choline acetyltransferase in homogenates of the hippocampus, but did not alter the binding of [3H]BZ by particles of such homogenates (8). The investigators proposed that presynaptic muscarinic sites that are innervated by the septal hippocampal nerve fibers do not bind the labeled benzilate, whereas postsynaptic muscarinic sites do bind it.
Ketchum (18) provided information that suggests that the brain is the only mouse organ that contains 3H 48 h after intraperitoneal injection of [3H]BZ. Perfusion of [3H]BZ through rat liver had indicated that this organ destroyed the compound quickly; 90% of the compound present originally in the perfusing fluid disappeared from the perfusate within 30 min, and less than 0.2% of the unaltered compound was found in the bile. The identity of the products was not determined.
The effects of BZ and Ditran, compared with atropine and scopolamine, have been examined to elucidate the mechanisms by which these compounds produce their effects. All these compounds have an extremely high affinity for muscarinic receptor sites, so the higher potency and longer duration of action of BZ must be related to other properties.
The long-lasting effects (up to 7 d) of a single injection of BZ in cats (19) raised the issue of possible irreversible interaction of BZ with brain sites. With another anticholinergic drug, [3H]pyrrolidylmethylphenylcyclopentylglycolate (20), a small amount of radioactivity was still measurable 3 d after a single injection. Considering the high affinity of BZ for muscarinic receptors (Kd=10−11 M), it would not be surprising to find BZ still attached to brain receptors 5–7 d later, especially because pharmacologic effects of BZ are evident in man after 7–8 d. The prolonged action of BZ, compared with its somewhat shorter duration of binding to CNS structures, is not yet readily explainable.
ANIMAL TOXICOLOGY OF ANTICHOLINERGIC COMPOUNDS
IMMEDIATE EFFECTS
BZ
BZ has been chosen as the paradigm of the various anticholinergic test compounds used at Edgewood. It was used in a large number of volunteers and is probably one of the best documented. Because it is also one of the longest-acting of all the drugs, it is also more likely to cause persistent adverse effects. It was one of the first glycolates used in relatively high doses in man, so more years have elapsed in which long-range consequences might have occurred.
The pharmacology and toxicology of BZ have been studied extensively in animals. Much of the information was derived through a contract with Hazleton Laboratories, Falls Church. A summary of the preclinical pharmacology and toxicology in rats, dogs and monkeys is presented here; a list of other toxicity studies is given in Appendix F. Additional background information is contained in Appendixes G, H, I, J, K and L.
Rats. Repeated intravenous administration of BZ to male albino rats was carried out for 20 daily injections during a 4-wk period. The doses included 0.01, 0.1, and 1.0 mg/kg.
The most significant pharmacologic sign noted was mydriasis. The daily pattern of mydriasis noted after each injection suggested metabolic inactivation of BZ by rats within 24 h. No tolerance to the mydriatic response was, however, apparent during the course of the study. At the high dose there appeared to be a progressive diminution in the intensity of pharmacotoxic signs (other than mydriasis); that suggested development of tolerance or decreased responsiveness to BZ.
Other measured indicators of pharmacologic effect included body weight, food consumption, hematology, gross and microscopic pathologic signs and organ weights. No significant effect attributable to the repeated administration of BZ was found.
There were no indications of liver disease or malfunction directly attributable to BZ in chronic-toxicity studies. In some rats, there were a few small foci of periportal lymphocytic infiltration; but these occurred as often in saline-treated animals.
Dogs. Repeated intravenous administration of BZ to male and female mongrel dogs was carried out for 20 d over a 4-wk period. The dosages were 0.01, 0.1, and 1.0 mg/kg-d. The indicators of effect were gross pharmacotoxic signs, daily body weight, hematology, biochemistry, urinalysis, organ weights, organ-to-body weight ratios, and gross and microscopic pathology.
A minimal number of effects was produced by low dosages between the eleventh and thirteenth injection days. Both the intermediate and high dosages produced mydriasis, ptosis, decreased activity, ataxia, and weakness of limbs. Other signs were less frequently observed. An increase in sedimentation rate occurred at 4 wk in one low- and one high-dosage animal. Liver weight was high in one male from each test group. Testicular atrophy was observed in one male each from the intermediate- and high-dosage groups. A dose of 10 mg/kg induced bradycardia in all of five dogs and resulted in death due to cardiac arrest in two of the animals (21). Dogs given daily intravenous doses of 100 μg/kg for 14 consecutive days had the same LD50 as dogs that had not been so exposed, but the interval between injection of the daily dose and the appearance of ataxia increased from 4 min to 14 min during the period of pretreatment. In dogs trained in a conditioned-escape routine and then given graded intravenous doses of BZ, doses up to and including 12.5 μg/kg had no effect on performance. However, a dose of 25 μg/kg resulted in failure to escape by four of four dogs tested (22). None of the experiments summarized in this report demonstrated any persistent effect of exposure to BZ in surviving dogs.
Monkeys. Repeated intravenous administration of BZ at 0.01, 0.1, and 1.0 mg/kg-d to male and female monkeys was carried out for 20 d over a 4-wk period. The indicators of effect were gross pharmacotoxic signs, daily body weight, hematology, biochemistry, organ weights, organ-to-body weight ratios, and gross and microscopic pathology.
The most frequently observed signs were mydriasis at all dosages and decreased activity and ataxia at the two highest dosages. Blood sugar of one monkey given an intermediate dosage and serum transaminase of one monkey given a high dosage were increased at 4 and 2 wk, respectively. The testicular weight and organ-to-body weight ratio were high for one intermediate-dosage animal. All other findings were within control limits.
Cycloplegia persisted in monkeys for more than 7 d after exposure to BZ, whereas most other effects had disappeared after 2 d (18).
EA 3443
Hazleton Laboratories conducted preclinical toxicity studies with EA 3580, EA 3392, and EA 3443. The following information was abstracted for EA 3443.
A total of 20 daily intravenous injections of EA 3443 in male and female monkeys at 0.01, 0.1, and 1.0 mg/kg produced no hematologic or biochemical abnormalities. Organs examined microscopically presented no significant alterations (23).
A total of 20 daily intravenous injections of EA 3443 in mongrel dogs (two dogs per dose) at 0.01, 0.1, and 1.0 mg/kg produced no hematologic, urinary, or biochemical changes. Histopathologic findings were essentially normal (24).
EA 3834
Prompted by a report of hematuria in a subject receiving EA 3834, research was begun on the effects of these drugs on ureteral and bladder motility (25). Microscopic hematuria was shown to occur within several minutes after intravenous administration of EA 3834 and usually cleared within 1 h. The dogs had ureteral or bladder catheters in place and often had a few red blood cells in the urine before administration of the agent. However, the red cells became much more numerous after administration. No additional information is available.
The hematuria was most likely the result of a reduction in renal blood flow. It is not clear that the hematuria was produced by EA 3834.
LONG-TERM AND DELAYED EFFECTS
Atropine
Atropine sulfate was administered intramuscularly to rabbits daily for 100 d. At a dose estimated to be 5% of the LD50, signs of toxicity were seen. Pathologic signs included weight loss, edema of most organs, hepatitis, pulmonary thrombosis, inhibition of spermatogenesis, thymic atrophy, and toxic changes in the gall bladder, spleen, and pancreas. The rabbits' survival of daily administration of atropine beyond the LD50 was related to their ability to maintain food intake (26).
Clidinium (Quartzan)
Clidinium was administered intragastrically to dogs at 1,5 and 25 mg/kg, 5 d/wk for 52 wk (27). The animals were observed for survival, behavior, and general physical condition. Blood, liver and kidney function were evaluated. Organs were examined microscopically at the conclusion of tests. Toxic signs of anticholinergic exposure were not seen. Hematologic characteristics were not significantly altered by these prolonged exposures. Clinical-chemistry measures and results of gross and microscopic evaluations of internal organs were within normal ranges.
Administration of clidinium for 1 yr at 5.0, 25, and 50 mg/kg in the diet of rats did not result in drug-related toxicity. Blood counts, clinical-chemistry measures, and results of gross and microscopic studies remained within normal limits (28).
Other Data
No study of the long-term administration of BZ to animals has been uncovered. The only long-term toxicity study with an ester of benzylic acid was with the ester with diethylaminoethanol; no significant pathemas were found in rats during this lifetime feeding study, but the lifetimes of the rats fed the benzilate may have been shortened somewhat. Feeding of the quaternized ester of 3-quinuclidinol to rats for a year (27) and gavaging of dogs with this compound 5 d/wk for a year (29) produced no significant pathemas.
With respect to genotoxicity, BZ has been found to have weak mutagenic activity in yeast cells in culture (30) and to produce gaps and breaks in chromosomes of bone marrow cells; it has not been proved to produce a heritable change in mammalian species. Diethylaminoethylbenzilate was more toxic cytogenetically than BZ, despite its lower anticholinergic activity. Unfortunately, there is too little information on these compounds to assume or exclude genotoxic effects. Although various test systems have been used (point mutations, chromosomal observations, and dominant-lethal effects), most observed effects probably resulted from general drug toxicity, rather than genotoxicity. The drugs have been tested at only a few concentrations, and interpretation of existing data does not permit estimation of the genotoxicity of BZ or even of atropine or scopolamine.
HUMAN TOXICOLOGY OF ANTICHOLINERGIC COMPOUNDS
DOSING CONSIDERATIONS
Atropine
Textbooks generally suggest that death from atropine poisoning may be expected after ingestion of doses above 100 mg. However, a review of the literature for the last 100 yr yielded a probit estimate of about 450 mg/person, based on several hundred cases of accidental poisoning, including some 40 fatalities. Because the oral route is somewhat less effective than the intravenous or intramuscular (and probably the inhalation) route, a conservative figure of 75 mg/person (approximately 900 μg/kg) is a working estimate for the human LD50.
Other Anticholinergic Compounds
Acute toxicity data on the 21 glycolates studied in man in a cool climate show a fairly consistent ratio between the lethal dose in animals and the heart-rate-increasing dose in man. Extrapolation of the animal data therefore allows an estimate of the risk associated with human exposure. Deaths have occurred in seemingly healthy people when atropine has been given at doses only 50 times greater than the heart-rate-increasing dose. Thus, the estimated LD50 in man for BZ is about 200 μg/kg, whereas that for EA 3834 is about 60 μg/kg. This represents safety margins of about 35 for BZ and 100 for EA 3834, where safety margin is the ratio between and ID50
In a warm climate, these numbers need correction, because the heat loss from sweating is diminished. It is speculated that fatal hyperthermia or heatstroke could occur at doses very close to the ID50 for BZ and not more than 2 or 3 times the ID50 for EA 3834.
In the Edgewood tests, no volunteer received BZ at more than 10% of the LD50. Probably no more than 30 or 40 subjects received more than 3% of the LD50.
Few subjects received more than one dose of a glycolate. In the case of BZ, seven subjects were given the ID50 on two occasions, 2–3 wk apart. Two subjects received 8.0 μg/kg twice each, in a crossover double-blind study of the efficacy of physostigmine as an antidote. Eight subjects were given 1 μg/kg daily for 7 d (with no appreciable effects), and four received 2 μg/kg on three consecutive days (with cumulative effects approaching incapacitation on the third day.) Thus, fewer than 10% of all subjects received more than a single dose. The figures for other glycolates are lower. The total exposure of any person to glycolate never exceeded 3 times the ID50 dose in the entire series of tests.
USE OF ANTIDOTES
Anticholinergic agents are typically used as treatment for anticholinesterase poisoning and vice versa. Physostigmine, tetrahydroaminoacridine, and other cholinesterase inhibitors were used successfully as antidotes in several dozen subjects. The demonstration of physostigmine's effectiveness led to the first controlled study of its ability to reverse delirium due to scopolamine (31). Physostigmine has been used to overcome anticholinergic toxicity.
IMMEDIATE EFFECTS
Acute clinical findings were recorded by involved physicians and nurses when Edgewood volunteers underwent tests with 21 anticholinergic chemicals shown in Table 2–2. About 1,750 subjects were tested with agents in this pharmacologic class, 10% of which were summarized for review. Selection for inclusion in this summary was based on high dose, high frequency of administration, or additional physiologic stress; it was the intent to focus on the extreme cases. The summaries identify the compound administered, dose, route of exposure, and significant acute reactions or clinical findings.
Peripheral and Behavioral Effects
In the brain, the arousal system that maintains alertness during waking hours is depressed by the action of BZ; this leads to drowsiness, which may progress to stupor or even coma at higher doses. Simultaneously, the coordinating motor systems of the cerebellum seem to be affected, and that causes an unsteady gait and pronounced clumsiness. There is a subjective sensation of dizziness which may arise in part from disturbances of the vestibular systems in the brainstem. The modulation of muscle tone is affected, possibly owing to an action on the Renshaw interneurons in the spinal cord, and the results are an abnormal increase in tendon reflexes and a tendency toward stiffness and jerkiness in muscle movement. As the drug penetrates higher in the brainstem, hypothalamic systems show signs of disruption. There is a decrease in drinking and eating. Sleep may be inhibited. A tendency toward increased body temperature appears in a warm environment. Finally, the highest integrative systems of the brain are affected. Memory, attention, problem-solving, and judgment are all seriously impaired. The ability to distinguish reality from imagination is lost, and thoughts and words flow in an uncontrolled manner. For a moment, the subject may seem to be in contact with his surroundings, but within seconds he drifts rapidly into incoherent speech and disconnected action. He is, at this stage, truly incapacitated and cannot function sufficiently even to preserve his life. If not brought under control, the delirious subject can inadvertently become a casualty and may fall prey to a fatal accident.
During the early stage of intoxication, activity is reduced, movements are slow and ineffective, and the subject is generally unable to offer effective resistance and tends to be relatively tractable. Once this period (a few hours) has passed, his behavior becomes increasingly unpredictable, energetic, and dangerous.
In the features thus far described, BZ is a typical glycolate, indistinguishable qualitatively from atropine, scopolamine, EA 3834, EA 3443, EA 3580, EA 302,196, or any other compounds of the 21 in this family studied in volunteers.
EEG Effects
There is a close relationship between the changes in EEG patterns and the behavioral effects of drug administration. With high doses of atropine (10–30 mg), for example, or repeated administration, the EEG shows an increase in slow waves, a decrease in mean frequency, a decrease in the percent time and amplitude of alpha activity, and an increase in the fast frequencies, which can be seen to be ‘riding' on the slow waves. There is a direct association between the EEG fast waves with behavioral restlessness and the EEG slow waves with stupor and cognitive defects. At (‘toxic') doses, patients are in stupor or coma, with high heart rate and lowered blood pressure. The EEG demonstrates persistent high-voltage slow waves, with a minimum of alpha and fast frequencies.
EEG and behavioral effects were modified in a parallel manner by the concurrent administration of antagonistic drugs. For instance, when patients who exhibited a toxic delirium to Ditran or atropine were given tetrahydroaminoacridine, a cholinesterase inhibitor, the stupor was relieved and the EEG showed a decrease in both slow and fast frequencies.
During acute administration of various compounds, the time for recovery varied with dose with low doses, the peak effects of parenteral administration were seen in 0.5 h and lasted up to 6 h; with high doses, the effects persisted for up to 24 h. With toxic doses, a return to baseline occurred in the second day after administration. Followup EEG data are limited, the principal data being reports of atropine toxicity. In these studies, the few statements referring to EEG changes suggest that the effects disappear within a few days of the last exposure.
For the test compounds used at Edgewood, EEG data are available only for BZ, EA 3580, and EA 3834 on five, two, and one patients,respectively. The pretreatment and posttreatment EEG records appeared to be within normal limits and reflected effects observed for other CNS-active anticholinergic drugs. Neuropsychologic effects of the exposures to the agents were transient, and test values reverted to baseline. The EEG changes and neuropsychologic effects were temporally related, and a similar reversion to normal EEG patterns was anticipated. No evidence of persistence of behavioral or EEG effects after these experimental trials was reported by Klapper et al. (32).
BZ Case Reports
Data on the 36 subjects whose records were selected for evaluation from the records of 354 subjects treated with BZ indicate that BZ is active when it is given by the intravenous, intramuscular, aerosol inhalation, or oral route. The fragmentary data available indicate that the higher the dose the greater the effects and the longer their duration. Understanding of the timecourse of effects was confounded by erratic written documentation, which at best was rather sparse, and by the introduction, at various times after exposure to the drug, of treatment with cholinomimetics, such as physostigmine and tetrahydroaminoacridine. Both these treatments ameliorated, at least temporarily and partially, some of the effects of BZ.
Intravenous Injection . BZ doses of 4.8 and 8 μg/kg were used in four subjects. The time course was approximately as follows:
- 1 h: Dry mouth, flushed face, numbness in extremities, and sleepiness.
- 4 h: Above, plus twitching, yawning, clonus and abnormal reflexes, and ataxia.
- 24 h: Above, plus disorientation, hallucinations, and poor performance on psychometric tests.
- 36 h: Hallucinations persisting without treatment.
- 48 h: Diminished nonverbal and motor skills.
Single Intramuscular Doses. BZ doses of 5.0–6.4 μg/kg were used in 15 subjects. These subjects were treated at various times with the antidotes mentioned above. The time course of action of the drug by this route was similar to that by intravenous injection; in fact, more symptoms were reported with this route, but this may have been due to differences in the completeness of reporting. The time course was approximately as follows:
- 10 min: Light-headedness and giggling.
- 30 min: Dry mouth, blurred vision, nausea, chilly sensations, and twitching.
- 1 h: Flushed skin, incoordination, fatigue, unsteadiness, sleepiness, and quivering legs.
- 2 h: Many of the above, plus poor concentration, restlessness, hallucinations, slurred speech, and muscle fasciculations.
- 3 h: Above, plus tremors.
- 4 h: Above, plus difficulty in handling subject and belligerence; pulse, 136.
- 8 h: Above, plus delirium and hallucinations.
- 24 h: Persistent delirium, hallucinations, restlessness, unsteadiness, and increased pulse in some but not all subjects.
- 48 h: Persistent impairment of function in some and return to apparent normal state in others, depending on vigor of antidotal treatment.
Repeated Intramuscular Injection Six Times Over Same Number of Days. This program was used in four subjects. Reporting was extremely fragmentary, but perhaps not much happened, inasmuch as the doses used were quite small—0.5 μg/kg. A maculopapular rash that cleared when treatment was stopped was noted twice, and dysphagia was noted in another subject. No mydriasis was seen. No tolerance could be demonstrated, probably because the dose was below threshold. No cumulative effect was noted.
Aerosol. Doses used ranged from 5.3 to 17.1 μg/kg in eight subjects. Understanding of the clinical manifestations was confounded by the use of antidotes at various times after exposure to the drug. The time course was as follows:
- 30 min: Restlessness, sleepiness, nausea, dry mouth, weakness, and coldness of extremities.
- 1 h: Above, plus dizziness.
- 2 h: Above, plus flushed skin.
- 4 h: Above, plus unsteadiness and disorientation.
- 8 h: Above, plus hallucinations.
- 24 h: Residual confusion, disorientation, hallucinations, and delirium in some, but not all, subjects.
- 48 h: Residual mental impairment, jumpiness, and shakes in some and improvement in others, depending on amount of antidote used.
In one subject, fever and spastic movements of the head were noted; he was treated vigorously with antidotes. At 3 h, he became unresponsive, showed decerebrate rigidity, had a high heart rate, and had urinary retention; he was treated vigorously with antidotes. Five years later (1968), he was hospitalized for microscopic hematuria and was shown by renal biopsy to have focal glomerulitis.
Oral. Doses ranged from 4.5 to 5 μg/kg and were given to five subjects. The major focus seemed to be on following heart rates and the EEG. Clinical manifestations noted with the other routes of administration probably also occurred here. Some heart rates became fairly high (130/min). One subject had an EEG tracing that showed episodes of light sleep with spindling.
Summary. In spite of the sketchy records, BZ appears to be an anticholinergic hallucinogen quite comparable with JB-329 (Ditran) or others of the JB series or with scopolamine. The onset of effects is usually rapid, regardless of the route of administration, and the duration is often a function of the dose. Although pharmacokinetic data on these compounds in man are unavailable, there is some evidence of a dose-response relationship. Without treatment to counter the effects of these strong centralanticholinergic agents, some effects may persist for several days. For instance, one subject who had received BZ displayed hyperthermia, tachycardia, and spastic movements for a few hours, and required vigorous treatment. He was discharged 6 d after exposure, well oriented and with normal appearance. No further information is available on him; the Veteran's Administration did not find his military records. Another subject developed signs of decerebrate rigidity with limb twitching. Two separate medical opinions were recorded: toxic encephalopathy and BZ delirium. The subject was discharged 6 d after exposure. EEG tracing 20 d after exposure was normal. His VA hospital record provided no useful information.
EA 3834
EA 3834 is somewhat distinctive from the other test compounds. The intravenous ID50 is approximately 5.7 μg/kg base equivalent, about the same as that for BZ. Although the time of onset of severe effects of EA 3834 is inversely related to dose (about 35 min for the ID50 and about 10 min for 3 times the ID50), the duration of severe effects is roughly constant within this dose range, namely 6–9 h. The constancy of recovery time from EA 3834, which is rather unusual for these drugs, reduces the period of medical care required for higher-dose casualties. In contrast, doubling the ID50 prolongs BZ incapacitation about 40 h, as it does for EA 3443 and EA 3580.
Physostigmine has proved effective as an antidote to EA 3834, as it has with many other glycolates. Within 20 min of a single intramuscular injection of 1 mg of physostigmine salicylate per 18 g of body weight, the stuporous subject becomes alert and able to perform at close to his normal level.
Possible renal toxicity has been reported in two men after the use of this compound. One volunteer who had received the agent intravenously had red blood cells in his urine shortly after the completion of his test. Extensive workup failed to uncover any definite kidney disease or lesion to account for the bleeding, which persisted intermittently for a year after exposure. Such a phenomenon had not been noted in any previous glycolate study. It may be related to a pre-existing abnormality that did not show up in routine screening. No additional information is available on the second patient. Animal studies had suggested that such bleeding could indeed result from EA 3834, from BZ, and even from atropine. On careful review of the experiment, it was concluded that the bleeding could be ascribed to techniques used and animal selection. In man, the effect of atropine on renal function was investigated, and no abnormalities were observed. Testing of EA 3834 was cautiously resumed in human subjects, and renal function was monitored with frequent pretest and posttest urine examinations. In a total of 69 subjects, including those who participated in field tests, no additional cases of bleeding were encountered, even though the subjects received up to 3 times the dose administered to subjects with hematuria. A decrease in glomerular filtration was observed in some subjects, but this also occurred in a control population. Nevertheless, continued vigilance concerning possible renal effects seems warranted.
Overall Evaluation of Immediate Adverse Effects
In general, in all the testing programs there were few violent responses or injuries, largely because of surveillance and safety precautions taken at Edgewood. The only other laboratory abnormalities encountered in the Edgewood records involved an increase in liver enzyme values of two volunteers within about 2 wk of exposure to CAR 302,368. These abnormalities reversed after a few weeks, and it was unclear whether the changes resulted from the drug administration. Several cases of pyuria after exposure to EA 3580, or related drugs, and running of an obstacle course, were reported, but the drug etiology is unclear.
LONG-TERM AND DELAYED EFFECTS
Delayed effects of the acute cholinergic drugs were extremely rare. One person reported by Klapper et al. (32) experienced “flashbacks” while still at Edgewood Arsenal. Edgewood records showed that he received EA 3834 intravenously. Medical records on this subject obtained from the VA were voluminous, but did not disclose any significant findings. Six-month followup studies performed by Hart and Balter (33) on related glycolates failed to demonstrate significant cognitive changes. Volunteers in a prospective psychometric study who had received EA 3167 showed no evidence of residual drug effects.
Two nonvolunteers—one military (a chemist) and one civilian (a pharmacologist)—were accidentally exposed to unknown doses of EA 3167, the most persistent of the glycolates studied. In each case, subjective and objective observations of performance over a period of 6–12 mo indicated that mild, but nontrivial, impairment of cognitive function could be discerned for approximately 6 mo, after which seemingly full recovery ensued. These patients were of superior intellect and had occupations that required optimal cognitive function for them to be successful. It is possible that in less demanding assignments they might not have been aware of residual deficits. Similar persistent decrements were not reported by BZ volunteers.
An additional ancedotal report (L.G.Abood, personal communication, 1982) concerns an evaluation of the psychologic state and well-being of three subjects who had been exposed to BZ during the preceeding 10 yr. Subject A had an oral dose of approximately 5 mg during the summer of 1969; subject B, an oral dose of approximately 10 mg in June 1972; and subject C, an oral dose of 5–10 mg in October 1976. All three were graduate students who had surreptitiously undertaken self-experimentation and who had been engaged in laboratory experiments with the drug. All experienced hallucinations, delirium, confusion, amnesia, and the full spectrum of psychologic, neurologic, and autonomic responses associated with the drug. Within 2–4 h after ingestion, all three were hospitalized in the psychiatric ward at the University of Rochester, Strong Memorial Hospital and retained for various periods (3–6 d).
Regarding the possible long-range effects of the drug experience, all three subjects were having difficulties both adjusting to graduate education and in their personal lives immediately before taking BZ. Subjects A and C had entertained the possibility of either dropping out or transferring to another university, largely because of inadequate academic performance. Within a few months after the drug experience, all three demonstrated definite improvement in both academic performance and psychologic well-being. They all appeared to be more out-going, communicative, and enthusiastic about graduate school. This assessment was shared by a number of faculty members and fellow students. They all eventually received Ph.D. degrees with distinction. They are now pursuing an active, productive research and postgraduate education. They all appear well-adjusted and well-motivated and seem to be leading productive, self-fulfilling careers. None of the subjects has experienced any adverse sequelae that might be attributable to the drug experience.
In the absence of additional reports of long-range consequences in subjects who had received the anticholinergic test compounds, data have been sought on delayed adverse reactions to other anticholinergic drugs used therapeutically, such as Ditran and atropine.
Since the introduction in 1958 of Ditran for the treatment of depressed patients (34), the number of persons receiving the drug for depression and other psychiatric disorders has exceeded 2,000 (34–37). During 1957–1963, Ditran was used in at least 200 people, many of whom were private patients. The treatment involved the intramuscular administration of 10–30 mg of Ditran to a depressed patient—a dose that generally produces the full spectrum of behavioral and neurologic effects attributable to the centrally active anticholinergics. The patient's psychiatric condition was closely observed and evaluated for the next few months. The progress of some of the private patients was followed for years after treatment, in some cases for 5 yr or more. In no instance was there any complaint of sequelae or delayed psychopathologic symptoms. The drug ameliorated depression for a period of a few months in a number of patients.
Hundreds of patients have received huge doses of atropine and scopolamine (up to 250 mg), sometimes given three times a week for up to 4 mo, and this form of therapy continues in Eastern Europe today. A chronic behavioral syndrome of toxicity appears unlikely, and single or even multiple exposures to the anticholinergic drugs used in the volunteers, frequently at low doses, are deemed insufficient to stimulate a persistent toxic syndrome. Of course, individual susceptibility to acute effects, which may trigger a long-term effect, cannot be excluded.
The question of occasional long-lasting or late-appearing residual effects is not easily answered. There is very little evidence that these might occur in man, in that so many persons have been exposed to these agents. The new case of glomerulitis several years after exposure in all likelihood was coincidental. Animal studies have been equivocal, but they have suggested the possibility of acute effects on the kidney and liver in some instances.
“Flashbacks” are difficult to explain, but they may result from a heightened sensory awareness after exposure to a drug. They have been of little clinical consequence, even though reported to be associated with a number of mind-altering drugs, including marijuana.
It should be emphasized that careful screening of volunteers for testing with the anticholinergic agents was probably essential, to avoid psychiatric complications after the hallucinatory episode. If a subject has latent psychopathology, the drug experience may precipitate the latent tendencies and create problems later. In one such instance, a subject receiving Ditran at a university medical school required psychotherapy for 2 mo after the drug episode. Psychiatric interviews and psychologic tests before the drug session indicated that this subject had borderline paranoid and homosexual tendencies; otherwise, he appeared normal. The subject was amnesic during the manifestation of these symptoms; but the complications ensued after other divinity students in his dormitory reminded him of his aberrant behavior under the influence of Ditran. The severe embarrassment resulting from this confrontation may have precipitated the anxiety and loss of self-confidence that required psychotherapy.
In summary, one cannot be certain that long-term adverse effects never follow exposure to anticholinergic agents, but it seems most unlikely.
MORTALITY
The deaths of 222 among some 6,620 soldiers have been analyzed. Table 2–4 shows the standardized mortality ratios (SMRs) comparing the observed numbers of deaths and the numbers expected for the U.S. population categorized by age and calendar year. Most of the SMRs for the various test groups are somewhat less than unity, although many of the groups are relatively small. The values for BZ, scopolamine, atropine, and “anticholinergic only” are 0.87, 2.50, 1.76, and 1.06, respectively. It is interesting that atropine and scopolamine, drugs with a long history of therapeutic use, had SMRs exceeding unity, whereas the four candidate chemical warfare agents (BZ, EA 3834, EA 3443, and EA 3580) had SMRs less than unity. This suggests that exposure to the test anticholinergic chemicals may not be related to a subsequent increase in mortality. For anticholinergic with or without other drugs, an SMR of 0.73 is statistically significant and probably represents a selection artifact, inasmuch as volunteers for these studies were especially screened for good health and thus would be expected to have lower than average mortality.
The mortality data do not reveal a cause for concern that significant numbers of the men exposed to the anticholinergics suffered an untimely death. Because the participants were all especially selected for these studies on the basis of their health records, there were relatively few deaths in all groups. It is impossible to determine how much less than 1.0 the SMR values should have been. Comparison of the values of the various groups would not be expected to reveal treatment effects unless these were sizable, which was not the case. However, a small drug effect might be masked by this type of data analysis. For the same reasons, further subdivision of the deaths by cause does not provide useful data. Mortality is discussed in greater detail in Chapter 4.
SUMMARY
The Panel has examined the assembled data on the possibility of long-term adverse effects associated with the testing of various anticholinergic compounds in human volunteers at Edgewood Arsenal.Although there are extensive descriptions of the acute CNS effects of the compounds, there is a paucity of information on delayed adverse effects. Animal experiments have not demonstrated significant long-term effects; and chronic administration of other anticholinergic drugs, such as atropine and scopolamine, which have been used therapeutically in man in high doses for years, has not been associated with chronic adverse effects. Indeed, there are anecdotal reports of improvement in behavioral patterns after such treatment. Because the CNS effects of the anticholinergic test compounds appear to be qualitively similar to those of atropine or scopolamine (although they may differ quantitatively in potency and duration of CNS effects), no additional long-term toxicity is anticipated. The apparent lack of an effect on mortality of the test subjects also suggests that such an effect is unlikely. Morbidity data are required to determine whether the subjects have had more health problems than those anticipated in a corresponding control group. This information is not yet available.
In a few persons, some relatively short-term adverse effects were reported after conclusion of the chemical testing (hematuria, hepatitis, and hallucinogenic flashbacks). It is unclear whether these effects resulted from the drug administration or occurred randomly and coincidentally. The extremely low incidence of such reports makes it doubtful that the anticholinergic test substances played a role in inducing these effects. However, the data are not sufficient to answer this question with certainty.
CONCLUSIONS
No firm evidence has been seen that any of the anticholinergic test compounds surveyed produced long-range adverse human health effects in the doses used at Edgewood Arsenal. More intensive study is required to confirm this conclusion. The high frequency of uncontrolled variables makes evaluation of behavioral effects difficult.
On the basis of available data, in the judgment of the panel, it is unlikely that administration of these anticholinergic compounds will have long-term toxicity effects or delayed sequellae. An ongoing morbidity study should provide more definitive information once it is completed.
Observed and Expected Deaths Among Test Subjects by Classification of Chemical Administered and Underlying Cause of Death
Anticholinergic a | BZ only a (N=136) | Scopolamine only a (N=85) | Atropine only a (N=62) | ||||||||||||
---|---|---|---|---|---|---|---|---|---|---|---|---|---|---|---|
Only (N=570) | Not only (N=1,179) | ||||||||||||||
No. deaths | Obs. | No. deaths | Obs. | No. deaths | Obs. | No. deaths | Obs. | No. deaths | Obs. | ||||||
Underlying cause of death | Obs. | Exp. | Exp. | Obs. | Exp. | Exp. | Obs. | Exp. | Exp. | Obs. | Exp. | Exp. | Obs. | Exp. | Exp. |
All trauma | 10 | 10.0 | 1.00 | 11 | 20.6 | 0.53 | 2 | 3.0 | 0.67 | 2 | 1.3 | 1.54 | 1 | 1.0 | 1.00 |
All diseases | 5 | 8.1 | 0.62 | 5 | 14.9 | 0.34 | 3 | 3.9 | 0.77 | 0 | 0.7 | - | 1 | 0.7 | 1.43 |
Malignant neoplasms | 1 | 1.8 | 0.56 | 3 | 3.3 | 0.91 | 0 | 0.9 | - | 0 | 0.2 | - | 1 | 0.2 | 5.00 |
Cardiovascular disease | 3 | 2.8 | 1.07 | 2 | 4.8 | 0.42 | 2 | 1.6 | 1.25 | 0 | 0.2 | - | 0 | 0.2 | - |
Cirrhosis of the liver | 1 | 0.6 | 1.67 | 0 | 1.2 | - | 1 | 0.3 | 3.33 | 0 | 0.1 | - | 0 | 0.1 | - |
Total deaths, all causes b | 19 | 18.0 | 1.06 | 20 | 35.5 | 0.56 | 6 | 6.9 | 0.87 | 5 | 2.0 | 2.50 | 3 | 1.7 | 1.76 |
EA 3443 only a (N=28) | EA 3580 only a (N=45) | EA 3834 only a (N=64) | Total (N=6,620) | ||||||||||||
No. deaths | Obs. | No. deaths | Obs. | No. deaths | Obs. | No. deaths | Obs. | ||||||||
Underlying cause of death | Obs. | Exp. | Exp. | Obs. | Exp. | Exp. | Obs | Exp. | Exp. | Obs. | Exp. | Exp. | |||
All trauma | 0 | 0.6 | - | 0 | 0.8 | - | 1 | 0.9 | 1.11 | 86 | 128.2 | 0.67 | |||
All diseases | 1 | 0.5 | 2.00 | 0 | 0.6 | - | 0 | 0.4 | - | 105 | 146.9 | 0.71 | |||
Malignant neoplasms | 0 | 0.1 | - | 0 | 0.1 | - | 0 | 0.1 | - | 30 | 32.9 | 0.91 | |||
Cardiovascular disease | 1 | 0.2 | 5.00 | 0 | 0.2 | - | 0 | 0.1 | - | 41 | 58.0 | 0.71 | |||
Cirrhosis of the liver | 0 | 0.0 | - | 0 | 0.0 | - | 0 | 0.0 | - | 7 | 10.5 | 0.67 | |||
Total deaths, all causes b | 1 | 1.1 | 0.91 | 0 | 1.4 | - | 1 | 1.2 | 0.83 | 222 | 275.1 | 0.81 |
- a
Only: This chemical and none other, except for innocuous and control substances. Not only: This and one or more other test chemicals.
- b
Includes causes of death listed here and all other causes, including those not yet determined.
REFERENCES
- 1.
- Goodman, L. and Gilman, A. 1980: Pharmacologic Basis of Therapeutics, editors of Sixth Edition; Macmillan Pub. Co., U.S.A.
- 2.
- Brimblecombe, R.W. 1974: Drug Actions on Cholinergic Systems. University Park Press, Baltimore.
- 3.
- Weiner, N. Neurotransmitter systems in the central nervous system. In Drugs and the Developing Brain. (Vernadakis, A., editor; , and Weiner, N., editor. , eds.) Plenum Press, New York, 1974, pp.105–131.
- 4.
- Rumack, B.H. 1973: Anticholinergic poisoning: treatment with physostigmine. Pediatrics, 52, 449–451. [PubMed: 4147165]
- 5.
- Ketchum, J.S.; Sidell, F.R.; Crowell, E.G., Jr.; Aghjanian, G.K.; and Hayes, A.H., Jr. 1973: Atropine, Scopolamine, and Ditran: Comparative pharmacology and antagonists in man. Psychopharmacologia, 28, 121–145. [PubMed: 4694622]
- 6.
- Shader, R.I., and Greenblatt, D.J. 1972: Belladonna alkaloids and synthetic anticholinergics. Uses and toxicity. In, Psychiatric Complications of Medical Drugs. (Shader, R.I., editor. , ed.) Raven Press, New York, 103–147.
- 7.
- Forrer, G.R. and Miller, J.J. 1958: Atropine coma: a somatic therapy in psychiatry, Am. J. Psychiatry, 115, 455–458. [PubMed: 13583249]
- 8.
- Yamamura, H.I. and Snyder, S.H. 1974: Muscarinic Cholinergic Receptor Binding in the Longitudinal Muscle of the Guinea Pig Ilium with [3H] Quinuclidinyl Benzilate. Mol. Pharmacol. 10:861–867.
- 9.
- Hulme, E.C.: Birdsall, N.J.M.; Burgen, A.S.V.; and Mehta, P. 1978: The binding of antagonists to brain muscarinic receptors. Mol. Pharmacol., 14, 737–750. [PubMed: 714022]
- 10.
- Levine, R.M. 1959: The intestinal absorption of the quaternary derivatives of atropine and scopolamine. Arch. Int. Pharmacodyn. Ther., 121, 146–149. [PubMed: 14416279]
- 11.
- Jonkman, J.H.G.; Van Bork, L.E.; Wijsbeek, J.; De Zeeuw, R. A.; and Orie, N.G.M. 1977: Variations in the bioavailability of thiazinamium methylsulfate. Clin. Pharmacol. Ther., 21, 457–463. [PubMed: 849677]
- 12.
- Zvirblis, P. and Kondritzer, A. 1966: Adsorption of H3BZ and C14-atropine to the mitochondrial fraction of the rat brain. Edgewood Arsenal Tech. Rept. 4042.
- 13.
- Sidell, F.R. undated: A summary of the investigations in man with BZ conducted by the U.S. Army, 1960–1969. CSL 000–137.
- 14.
- Snyder, S.H., Chang, K.J., Kuhar, M.J., Yamamura, H.I. 1975: Biochemical Identification of Mammalian Muscarinic Cholinergics Receptor. Fed. Proc. 34:1915–1921. [PubMed: 808430]
- 15.
- Yamamura, H.I., Chang, K.J., Kuhar, M.J., Snyder, S.H. 1975: Cholinergic Muscarinia Receptor: Biochemical and Light Autoradiographic Localization in the Brain. Croat. Chem. Acta. 47:475–488.
- 16.
- Becker, M.J. 1964: Biochemical Studies of BZ and EA 3443. Final Report, Contract No. DA-18–104-CML-6631.
- 17.
- Yamamura, H.I., Kuhar, M.J., Greenberg, D., Snyder, S.H. 1974: Muscarinic Cholinergic Receptor Binding: Regional Distribution in Monkey Brain. Brain Res. 66:541–546.
- 18.
- Ketchum, J. 1963: The human assessment of BZ. Chemical Research and Development Laboratories Tech. Memo 20–29.
- 19.
- Lowy, K., Abood, L.G. and Raines, H.: 1976. Behavioral effects and binding affinities of two stereoisometric psychotomimetic glycolates. J. Neurosci, Res. 2, 157–165. [PubMed: 950679]
- 20.
- Abood, L.G. and Rinaldi, F.: 1959. Studies with a tritium labeled psychotomimetic agent. Psychopharmacol. 1:119–123. [PubMed: 13791503]
- 21.
- Shallek, W. and Smith, T.H.F. 1952: Electroencephalographic analysis of side effects of spasmolytic drugs. J. Pharmacol. Exp. therap. 104:291–298. [PubMed: 14908898]
- 22.
- McNamara, B.P. 1960: Research in Toxicology Division on Effects of CS4030 in animals. Chemical Warfare Laboratories Special Pub. 2–28.
- 23.
- Mennear, J., Samuel, G., Jennings, H, McCarthy D., OH, J., Marshall, E. and Smith, B. Preclinical Pharmacology Study— Monkeys (EA3443). Contract DA 18–108-AMC-78[A] (C73, Index 105).
- 24.
- Mennear, J., Jennings, H., Kuldell, K., Hall, D., Poland, R. and Lamb, R. Preclinical Pharmacology Study—Dogs. Contract DA-18–108–405-CML-826.
- 25.
- Shiner, P.T., Maj., M.C., Agent EA3834-renal evaluation as of Jan. 1970, Clinic Investigation Branch, Clinical Medical Sciences Dept., Med. Res. Labs.
- 26.
- Boyd, C.E. and Boyd, E.M. 1962. The chronic toxicity of atropine administered intramuscularly to rabbits. Tox. Appl. Pharm. 4:457–467. [PubMed: 13872141]
- 27.
- Bagdon, R.E., 1965: One year chronic toxicity studies of quartzan in dogs, Dec. 13, 1965. Hoffmann-LaRoche, Inc. (Prepared by Food and Drug Res. Labs. Inc. and submitted to Hoffmann LaRoche Dec. 8, 1965 for use in connection with new drug application to FDA for human use).
- 28.
- Bagdon, R.E. and Garner, E., 1966: One year chronic toxicity studies of clidinium bromide in rats. April 20. Hoffmann-LaRoche, Inc.
- 29.
- Carson, S. 1965: Chronic (1-year) oral dosage studies with Quartzan in dogs. Report from Food and Drug Research Labs., Inc. to Hoffmann LaRoche, Inc.
- 30.
- Sram, R.J., Kucerova, M. and Bardode, Z. 1975: Mutagenic activity of 3-chinuclidylbenzilate. [PubMed: 769479]
- 31.
- Crowell, E.B., Jr., and Ketchum J.S. 1967: The treatment of scopolamine-induced delirium with physostigmine. Edgewood Arsenal Tech. Rept. 4115.
- 32.
- Klapper, A.J., McCarroll, J.E. and McColloch, M. 1972: Long-term followup of medical volunteers. Edgewood Arsenal Tech. Rept. 4611.
- 33.
- Hart, J.J. and Balter, L.: 1966. A study of possible residual EFFECTS of EA 3443 and EA 3580 on COG Nitive Ability [U]. Aberdeen Proving Ground, Maryland.
- 34.
- Abood, L.G. and Meduna, L.J.: 1958. Some effects of a new psychotogen in depressive states. J. Nerv. Ment. Dis 127, 546–554. [PubMed: 13621223]
- 35.
- Meduna, L.J. and Abood, L.G.J. Neuropsych. 1:20–22,1959. “Studies of a New Drug (Ditran) in Depressive States.” .
- 36.
- Finkelstein, B.A.: 1961. Ditran, a psychotherapeutic advance: A review of 103 cases. J. Neuropsychiat, 2, 144–148. [PubMed: 13699642]
- 37.
- English, D.C.: 1962. Reintegration of affect and psychic emergence with Ditran. J. Neuropsychiat. 3, 304–310. [PubMed: 13890286]
Footnotes
- *
Most of the information in this section is from Chapter 7 of Goodman and Gilman (1).
- ATROPINE, SCOPOLAMINE, AND RELATED THERAPEUTIC ANTICHOLINERGIC CHEMICALS
- PHARMACOLOGICAL PROPERTIES OF SYNTHETIC ATROPINE DERIVATIVES
- ROLE OF DRUG DISPOSITION IN EXPLAINING DIFFERENCES AMONG ANTICHOLINERGIC COMPOUNDS
- ANIMAL TOXICOLOGY OF ANTICHOLINERGIC COMPOUNDS
- HUMAN TOXICOLOGY OF ANTICHOLINERGIC COMPOUNDS
- SUMMARY
- CONCLUSIONS
- REFERENCES
- PubMedLinks to PubMed
- ANTICHOLINERGICS - Possible Long-Term Health Effects of Short-Term Exposure to C...ANTICHOLINERGICS - Possible Long-Term Health Effects of Short-Term Exposure to Chemical Agents
- rab7a [Acanthochromis polyacanthus]rab7a [Acanthochromis polyacanthus]Gene ID:110955289Gene
- mtif2 [Acanthochromis polyacanthus]mtif2 [Acanthochromis polyacanthus]Gene ID:110970649Gene
- gtpbp6 [Acanthochromis polyacanthus]gtpbp6 [Acanthochromis polyacanthus]Gene ID:110968971Gene
- SRPRB SRP receptor beta subunit [Gallus gallus]SRPRB SRP receptor beta subunit [Gallus gallus]Gene ID:101750392Gene
Your browsing activity is empty.
Activity recording is turned off.
See more...