NCBI Bookshelf. A service of the National Library of Medicine, National Institutes of Health.
Institute of Medicine (US) Committee on Nutrition, Trauma, and the Brain; Erdman J, Oria M, Pillsbury L, editors. Nutrition and Traumatic Brain Injury: Improving Acute and Subacute Health Outcomes in Military Personnel. Washington (DC): National Academies Press (US); 2011.
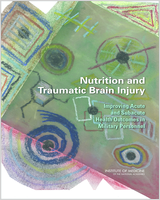
Nutrition and Traumatic Brain Injury: Improving Acute and Subacute Health Outcomes in Military Personnel.
Show detailsThe relationship between nutrition and brain function continues to be investigated, and there is still much to be discovered about brain function, brain disease processes, and interventions to improve or restore brain function following injury. There are likewise numerous challenges in identifying pharmaceutical interventions to improve resilience or treat traumatic brain injury (TBI). Despite years of pharmaceutical research, there is still no effective drug treatment for TBI (see Hall in Appendix C); since the 1970s, not a single phase III human clinical trial has shown a significant benefit (Maas et al., 2010).
In this second part of the report, the committee presents its core recommendations. In particular, this chapter describes the committee’s rationale for selecting specific nutritional interventions for review. Briefly, the committee based its selection on the current understanding of the injury process and of mechanisms of action of specific nutritional interventions. When the function and biochemistry associated with a particular nutritional approach appeared promising, a literature search was initiated. Based on the experience with pharmaceuticals mentioned above, the committee understands that nutrition will not be the sole treatment for TBI, but it is a potentially powerful adjunct to promote metabolic support during wound healing, and may even exert neuroprotection in some instances if provided prior to injury.
GENERAL APPROACH FOR SELECTING NUTRITIONAL INTERVENTIONS
The committee’s approach to identifying nutritional interventions of potential use in the treatment of TBI consisted of expert discussions, literature reviews on the pathophysiology of TBI, initial identification of promising nutrients, and reviews of their effectiveness.
Expert Discussions
A public workshop was held June 23–24, 2010, in Washington, DC, where invited civilian and military subject-matter experts (e.g., medical experts, nutritionists, and neu roscientists) presented their findings and experiences on various TBI-related topics, with a focus on potential nutrient interactions with the pathogenic sequelae of TBI. The committee also reviewed the existing literature examining the pathology and metabolic alterations of the insult with the goal of identifying nutrients that had the potential to favorably alter metabolism, oxidative stress, and tissue structure and repair, following and sometimes in advance of TBI.
Review of the Literature on Pathophysiology and Identification of Targets for Nutritional Interventions
As a background to elucidate the committee’s rationale, a summary of current knowledge about the pathophysiology of TBI was presented in Chapter 3. Briefly, brain injuries differ dramatically from patient to patient depending upon the location, type, intensity, and duration of the initiating concussion force. One common feature of severe brain injury is reduced blood flow and oxygen deficiency. Neuronal membranes become damaged and ion channels malfunction, leaking proteins and neurotransmitters. Apoptotic genes are activated, brain cells begin to swell, and mitochondrial function diminishes as the brain cells enter a “death spiral” (Scudellari, 2010). Production of free radicals increases and calcium ions are released, resulting in tissue damage and cell death (Mattson and Chan, 2003). For the purposes of this report, TBI pathology has been divided into primary, secondary, and long-term injury mechanisms to permit more specific focus on the timing and locus of the nutrient intervention. Box 4-1 shows a summary listing of adverse physiological responses as well as select potential neuroprotective targets (e.g., control of inflammation, angiogenic and neurogenic repair and recovery) discussed during the June public workshop and committee meetings that might respond to nutritional interventions. Box entries with an asterisk indicate especially promising potential targets for nutritional intervention. These potential nutritional targets and the mechanism or metabolic bridge linking them to TBI treatment or prevention have been grouped into three topic areas and will be discussed briefly in this chapter. Table 4-1 matches these potential nutritional targets with nutrients having relevant mechanisms of action; however, the reader should make no conclusions about the clinical efficacy of any of these nutrients based on this table. The information in this table is not meant to imply that there is any evidence from animal models or human trials showing benefits in ameliorating TBI. The table was constructed to illustrate that those nutrients and food components act on multiple and diverse processes that are common to brain injury, and therefore their effects (beneficial and adverse) should be reviewed.
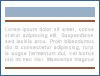
BOX 4-1
Physiologic Responses to TBI and Protective/Reparative Targets. Physiologic responses Intracranial hemorrhage
TABLE 4-1
Plausible Mechanisms of Action by Selected Nutrients and Food Components That Might Affect TBI Outcomes.
For most nutrients, more than one mechanism is proposed. The scientific community believes that, based on the variations in injuries, responses, and outcomes after TBI, no single nutritional mechanism will serve as the magic bullet for TBI. The evidence on the ability of these putative nutrients to improve resilience or ameliorate the effects of TBI will be discussed in detail in subsequent chapters (Chapters 6–16).
Review of the Literature on Effectiveness of Nutrients
From its discussions, the committee identified an initial list of search criteria and promising pre- and postinjury nutritional interventions that might be useful adjuncts in the overall medical treatment and prevention of TBI. The committee decided on the search criteria and vocabulary included in Box 4-2.
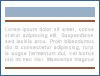
BOX 4-2
Literature Search Criteria and Vocabulary. Inclusion Criteria Health condition: patients or animal models of TBI (including in the developing brain), subarachnoid hemorrhage, intracranial aneurysm, stroke, anoxic or hypoxic ischemia, epilepsy
NUTRITIONAL MODIFIERS OF TBI: PROMISING TARGETS
The treatment and recovery of TBI typically involves one or more of the following interventions: fueling hypermetabolism, reestablishment of vascularity, supporting brain plasticity, prevention of posttrauma protein tangle development, and controlling glutamate excitotoxicity. For the purposes of this report, the potential nutritional approaches targeting these interventions and the mechanism or metabolic connection linking them to TBI have been grouped into three target areas: (1) restoration of cellular energetics, (2) reduction of oxidative stress and inflammation and repair of cellular damage, and (3) repair and recovery. Each of these approaches is discussed briefly below, with example(s) of potentially useful nutrients that may have implications across several of these target areas. It is important to note that nutrients typically have multiple modes of action, interacting with various aspects of the potential target areas, so they may be considered in more than one target area.
Restoration of Cellular Energetics
Metabolic characteristics of TBI include cerebral acidosis, hypoxia, decreased adenosine triphosphate (ATP) production, and resultant mitochondrial malfunction followed by apoptotic cell death. Apoptosis can be triggered by many different stimuli such as certain cytokines, toxins, oxidative stress, and calcium influx through damaged plasma membrane channels or release from the endoplasmic reticulum. Mitochondrial calcium dynamics are involved in the regulation of cellular energy metabolism and neurotransmitter release (Mattson and Chan, 2003). Although it is a nutrient, calcium acts in this case as a messenger to coordinate cellular reactions that drive apoptosis. Calcium release is normally under tight physiological control, but this normally close regulation is altered by the events following a traumatic brain injury. Calcium released from the endoplasmic reticulum triggers a mass exodus of cytochrome C from mitochondria in the cell, activating caspases and other nuclease enzymes that finalize cell death (Mattson and Chan, 2003). Many of the hypothetical mechanisms of nutrients included in this report may have the effect of preventing apoptosis. Neuronal apoptosis can be induced by activation of glutamate receptors confined to the postsynaptic membrane of a dendrite, permitting a local influx of calcium that triggers cytochrome C release from mitochondria throughout the dendrites and cell body (Boehning et al., 2003). The series of events that triggers the malfunctioning of mitochondria severely disrupts the energetics of the cell. Thus, restoration of mitochondrial function or providing sources of energy other than glucose is a key objective after a TBI event. In addition, nutrients or food components that counteract or prevent ionic disturbances and excitotoxicity have the potential to improve outcomes of TBI. One such nutrient is magnesium, which plays a role in the pathobiology leading to TBI (Sen and Gulati, 2010). The level of magnesium in the brain has been found to affect the influx of calcium and glutamate to the postsynaptic neuron, one potential mechanism by which magnesium exerts its effects.
Several other food components could potentially ameliorate the described effects on mitochondrial malfunction by augmenting energy metabolism. Creatine, a nitrogenous guanidine compound found naturally in vertebrates, accesses the brain via a creatine transporter. Creatine and the creatine kinase/phosphocreatine system serve to buffer energy in tissues with high and fluctuating energy requirements (e.g., muscle and brain). Since the brain requires significant ATP turnover to maintain membrane potentials and its signaling capacity, creatine metabolism and the creatine kinase/phosphocreatine system are important for normal brain functioning; these may be compromised in diseases of the central nervous system. Studies also suggest that creatine’s neuroprotective effects are at least partly due to increased cerebral blood flow and reduction in the processes associated with secondary brain damage, such as an increase in free fatty acid and lactate (Sullivan et al., 2000).
Acetyl-L-carnitine (ALC), another food component, has been shown to confer neuro-protection in models of both global and focal ischemia (see review, Zanelli et al., 2005). It is postulated that ALC serves as an alternative source of acetyl coA, and as such promotes aerobic energy metabolism and reduces tissue acidosis. With reduced tissue acidosis, oxidative stress may likewise be ameliorated. Data further suggest that ALC modulates excitotoxicity, apoptosis, and inflammation. The beneficial effects of ALC may reflect the targeting of multiple pathogenic pathways. However, a review of the literature did not deliver enough evidence for the committee to write a chapter and make recommendations. Only a few studies have been done in animal models of ischemia. In addition, improvements in neurological outcomes were only seen when ALC was administered within 30 minutes after the injury, which might not be feasible in the field. The acetate precursor glyceryl triacetate, a food additive and dietary supplement, has also been found to effectively supply acetyl coA as an alternative energy source in an animal model of TBI (Arun et al., 2010), which can contribute to more immediate energy production for mitochondria than glucose.
Essential nutrients might also be promising in the treatment of TBI. Dietary branched-chain amino acids (BCCAs) are important for de novo synthesis of glutamate, which plays an important role in maintaining synaptic neurotransmitter pools. In an experimental model of TBI, BCAAs (i.e., valine, isoleucine, leucine) were found to be reduced (Cole et al., 2010), and dietary delivery resulted in improved cognitive outcomes that correlated with restored net synaptic efficacy. Although these findings are quite encouraging, further studies, as recommended in the next chapter, are needed to determine if this benefit is sustained over time. It is postulated that BCAAs are beneficial as precursors of neurotransmitters as well as providing an alternative energy source. It is unclear to what extent the synthesis neurotransmitters might contribute to the potential effects of BCAAs in TBI, because BCAAs influence the synthesis of both excitatory and inhibitory neurotransmitters.
Other promising interventions include specific diets rather than a single food component or nutrient. The ketogenic diet is a high-fat, low-carbohydrate diet used as a therapy for epilepsy, especially in children (Beniczky et al., 2010). This diet provides an alternative source of fuel to the brain and enhances energy production in the brain when glucose utilization is impaired. Although the mechanisms of protection in epilepsy are not completely understood, it appears that following a ketogenic diet provides resistance to metabolic stress and may thereby provide protection to the injured brain. Animal studies have shown that ketogenic diets improve mitochondrial antioxidant status and protect the DNA (deoxyribonucleic acid) from oxidative damage by increasing glutathione, a major mitochondrial antioxidant, and by reducing both acetyl coA, an indicator of mitochondrial redox status, and lipoic acid, a thiol antioxidant. One drawback of ketogenic diets is that patient compliance is difficult to maintain. Alternative diets could be developed that are more organoleptically acceptable and have similar effects in the brain.
Reduction of Oxidative Stress and Inflammation and Repair of Cellular Damage
Oxidative stress and chronic inflammation are among the root causes of many diseases, such as cardiovascular disease, insulin resistance, autoimmune diseases like rheumatoid arthritis, and Alzheimer’s disease. Brain injuries such as ischemic brain injury and TBI have also implicated oxidative stress as a key pathological factor in secondary injury progression. Mitochondria, the main source of cellular ATP production under normal aerobic conditions, are susceptible to reactive oxygen species (ROS), which generate localized oxidative stress. Injury to the brain also promotes inflammation, which can eventually lead to further tissue damage if not well regulated and resolved promptly. These observations suggest that dietary antioxidant and anti-inflammatory therapy may be useful approaches to promote protective mechanisms in the injured brain (Wu et al., 2006).
One class of nutrients that could be important to improve TBI outcomes is niacin (i.e., vitamin B3, nicotinamide adenine dinucleotide [NAD+]) and its derivatives, since it plays an important role in maintaining and regulating cellular redox states, calcium ion stores, DNA damage and repair, response to cellular stress, and energy metabolism (Xu and Sauve, 2010). Enhanced oxidative stress can induce DNA strand breaks, which in turn are repaired by activation of poly(ADP-ribose) polymerase (PARP). Under certain pathological conditions such as trauma and resulting sepsis, this process of DNA damage and repair can consume large amounts of NAD+, leading to its cellular depletion, which in turn promotes inflammation (Wendel and Heller, 2010). The nuclear protein poly(ADP-ribose) polymerase-1 (PARP-1) functions as a molecular stress sensor and DNA repair mechanism. Overactivation of PARP-1 followed by NAD+ depletion is a major contributor to ischemic brain damage (Love, 1999). PARP-1 is believed to be a key mediator of cell death in excitotoxicity, ischemia, and oxidative stress (Alano et al., 2010). Reactive oxygen species strongly activate the enzymatic activity of PARP-1 (Altmeyer and Hottiger, 2009). Excessive activation of PARP-1 is believed to be a key link between oxidative stress, glutamate excitotoxicity, and cell death (Ying and Xiong, 2010; Yu et al., 2009). NAD+ links PARP-1 to energy metabolism (Altmeyer and Hottiger, 2009), suggesting that NAD+ depletion, such as that observed in TBI, is a causal event in PARP-1 mediated cell death. Preventing the depletion of cytosolic NAD+ that characterizes the massive disruption of energy metabolism following TBI could therefore ameliorate the chain of events that lead to cell death. Despite this role in regulating redox states, a literature search on niacin did not reveal any study to explore its effects in outcomes of TBI. For this reason, niacin was excluded from the list of promising nutritional approaches.
There are numerous other nutrients and food components that have either antioxidant or anti-inflammatory effects and that could potentially be beneficial in TBI pathology. The committee initially selected some because they have been studied extensively in connection with cardiovascular disease and other chronic diseases having inflammation as one of their etiologies. Vitamin E (alpha-tocopherol) is a potent reactive species scavenger that acts as an inhibitor of lipid peroxidation (Marklund et al., 2006). In a 2010 experimental model of TBI, dietary supplementation with alpha-tocopherol has been shown to reduce protein oxidation, increase levels of the antioxidant superoxide dismutase, and improve cognitive outcome (Wu et al., 2010). Identifying compounds such as alpha-tocopherol or alpha-eleostearic acid that mitigate oxidative stress or regulate the release of apoptosis-releasing factors from the mitochondria may yield important therapeutic approaches for the nutritional treatment of neuronal injury (Kondo et al., 2010).
Brain development and function are reliant upon n-3 fatty acids (Bazan, 2007). This group of fatty acids is of particular interest in the setting of TBI because of its potential role as a modulator of inflammation. Two fatty acids, the n-3s docosahexaenoic acid (DHA— C22:6) and eicosapentaenoic acid (EPA—C20:5), support resolution of inflammation (see review, Kidd, 2007). While their mechanisms of action are currently being elucidated, Serhan and colleagues in Appendix J describe a number of studies that led to this conclusion. A hallmark finding of this research is that DHA is metabolized to resolvins and protectins, which are hypothesized to resolve inflammation and protect the brain from further damage. Endogenous neuroprotectin D1, while elevated in a model of brain ischemia, may not be sufficient to thwart the pro-inflammatory response; exogenous administration (intraventricular infusion), however, may reduce inflammation and result in neuroprotection (Bazan, 2007). Taken together, it appears that the mechanistic effects of diets high in n-3 fatty acids, such as DHA or its analogues, warrant further investigation for ameliorating the adverse effects of TBI.
Many phytochemicals, such as flavonoids, may also exert benefits because of their antioxidant or anti-inflammatory actions. Flavonoids consist of two aromatic rings bound together by three carbons that form an oxygenated heterocycle. Flavonoids can be classified into seven subclasses: flavonols (e.g., quercetin), flavones (e.g., apigenin), flavanones (e.g., hesperetin), flavan-3-ols (e.g., epicatechin), anthocyanins (e.g., cyanidin), polymers (e.g., proanthocyanidins), and isoflavones (e.g., genistein). The flavanol epicatechin was shown to be neuroprotective in a stroke model of brain injury (Shah et al., 2010). It is thought that this flavanol supports antioxidant defense mechanisms in the brain by activating the transcription factor Nrf2. This results in an increase in the enzyme heme oxygenase-1, which confers neuroprotection in part by degrading the prooxidant heme to the antioxidants biliverdin and bilirubin (Chang et al., 2005). In the setting of TBI, where heme arises from the breakdown of hemoglobin as well as the release of heme from dying cells, epicatechin may prove to be beneficial (Doré, 2010).
One food component with anti-inflammatory properties is resveratrol (3,4′,5-trihydroxystilbene). Resveratrol belongs to a class of polyphenolic compounds called stilbenes. Some types of plants produce resveratrol and other stilbenes in response to stress and injury, presumably to aid in their recovery process. Resveratrol has been shown to be neuroprotective in animal models of cerebral ischemia. Its exact mechanism of action is not known; there are several hypotheses, one of which is that it acts indirectly by increasing sirtuin-1 (SIRT1) activity during recovery or by sparing cells via 5′ adenosine monophosphate-activated protein kinase’s (AMPK’s) elevation of NAD+ levels. The NAD-dependent deacetylase, SIRT1, is an enzyme that deacetylates proteins involved in cellular regulation, including reaction to stressors. Recent evidence suggests that SIRT1 inhibitors may be neuroprotective. Based on in vitro studies, resveratrol is also hypothesized to inhibit the activity of several inflammatory enzymes, including cyclooxygenase and lipoxygenase, resulting in a suppressive effect on inflammatory and oxidative stress (Ghanim et al., 2010). Yet another hypothesis states that resveratrol may also inhibit proinflammatory transcription factors, such as NFκB or AP-1. This is supported by a human randomized controlled trial in which resveratrol was shown to exert anti-inflammatory and antioxidative stress effects in normal humans when measured by blood biomarkers (Ghanim et al., 2010). The suppressive effects of this extract on ROS generation and a range of inflammatory mediators suggest that resveratrol may induce its effects through increased expression of anti-inflammatory cytokines and a reduction in proinflammatory molecules, all potentially beneficial implications for TBI.
Curcumin, a phenolic yellow pigment from the rhizome Curcuma longa used as a flavoring and coloring agent, especially in curries, is an antioxidant and an anti-inflammatory agent. Curcumin is a potent inhibitor of the proinflammatory cytokine IL-1β and the transcription factor NFκB that are involved in cellular responses to stimuli such as stress, cytokines, and free radicals (Laird et al., 2010).
One compound that deserves attention because of its potential synergism with progesterone therapy is vitamin D. The hormone progesterone and its metabolites are considered pleiotropic drugs that confer neuroprotection by preventing the expression of inflammatory cytokines in the brain while reducing apoptosis, lipid peroxidation, and cerebral edema (Beauchamp et al., 2008) and stimulating growth-promoting factors that may play a significant role in remyelination of damaged neurons. The dosage of progesterone effective for treatment is too high to be feasibly achieved with current commercial dietary supplements. The committee therefore did not consider it as a potential stand-alone nutritional approach to the treatment of TBI; rather, it considered vitamin D3 as an example of a nutrient that may act synergistically with a pharmaceutical product, progesterone. Cekic and Stein (2010) suggest that vitamin D, a potent modulator of cell cycle, immune function, and calcium homeostasis, can work synergistically with progesterone therapy to reduce post-TBI inflammation.
Although microbial proteases have been used as anti-inflammatory agents (e.g., in wounds and hematomas), a review of their effects using the criteria in Box 4-2 did not identify any active research with proteases and TBI or associated conditions; therefore, they were neither considered as promising interventions nor reviewed in this report.
Repair and Recovery
Studies in the late 1990s showed that the brain has the capacity to generate new cells and that these cells can, under defined conditions, become functional. These findings, together with key studies showing the plasticity of the somatosensory circuits, serve as a foundation for understanding mechanisms of adaptability after a brain injury (see review, Kidd, 2009). Repair and recovery mechanisms likely involve revascularization of damaged tissue, neurogenesis, axonal/dendritic plasticity, and changes in neuronal networks. These events may be susceptible to nutritional augmentation approaches. For example, neurotrophins regulate neuronal survival, growth, and synaptic plasticity and neurotransmission, and protect against neuronal injury. One neurotrophin that has received particular attention is brain-derived neurotrophic factor (BDNF), modulated by diet and exercise. Brain-derived neurotrophic factor has been shown to regulate survival, growth, and differentiation of neurons during development, and is associated with learning and memory skills.
The nutrient vitamin E may act to improve neuroplasticity. Utilizing a fluid percussion model in rats, Wu et al. (2010) found that rats receiving dietary supplementation with vitamin E prior to TBI suffered less loss of BDNF and showed better-preserved brain cognition and synaptic plasticity than rats receiving no vitamin E supplementation. Thus, in addition to its antioxidant activity, vitamin E may exert its action via other targets, such as those supporting neuroplasticity.
Dietary curcumin has also been investigated for its potential to counteract the outcome of TBI on synaptic plasticity and cognition, as well as for its antioxidant properties (Wu et al., 2006). Dietary supplementation reduced oxidative damage and normalized levels of BDNF and cyclic AMP-response element-binding protein. It was therefore suggested that oxidative stress may influence synaptic plasticity and cognitive recovery through its actions on BDNF.
Cytidine 5′-diphosphocholine (citicoline or CDP-choline) is an essential intermediate in the biosynthetic pathway of cell membrane phospholipids. It is naturally present in eggs and liver, and also sold as a dietary supplement. It is readily absorbed by the oral route, with no reported serious side effects, and is subsequently widely distributed throughout the body where it is incorporated into membrane and microsomal phospholipids. The two principal components of CDP-choline, cytidine and choline, can cross the blood-brain barrier where they then become incorporated into lipid membranes (Jain, 2008). CDP-choline has been shown to inhibit apoptosis associated with cerebral ischemia and edema and to potentiate neuroplasticity in experimental models. It exerts neuroprotection in hypoxic and ischemic conditions (Secades and Lorenzo, 2006) as well as in rodent models of TBI (Kokiko and Hamm, 2007; Marklund et al., 2006), and is currently in phase III clinical trials for TBI, sponsored by the National Institute of Child Health and Human Development (NICHD) (Jain, 2008; Zafonte et al., 2009).
The antioxidant effects of flavonoids, polyphenolic compounds found in fruits and vegetables as well as wine, tea, and other beverages, are well known. They also exert a multiplicity of neuroprotective actions within the brain, including a potential to protect neurons against injury induced by neurotoxins; an ability to suppress neuroinflammation; and the potential to promote memory, learning, and cognitive function (Spencer, 2009). Flavonoids are believed to interact with critical protein and lipid kinase signaling cascades in the brain that lead to an inhibition of apoptosis and to a promotion of neuronal survival and synaptic plasticity (Spencer, 2009). Based on their actions, it is possible that flavonoids also hold potential to aid in recovery from brain trauma, although this particular aspect of flavonoid therapy has not been the subject of human clinical trials. Spencer (2009) suggests that flavonoids increase peripheral and cerebral blood flow, which in turn support angiogenesis and neurogenesis. Flavonoids likely have other effects on the injured brain, targeting post-transcriptional factors and gene expression, and scavenging neurotoxic and proinflammatory species. Yao and colleagues (2010) also observed that Epimedium (a genus of more than 60 species of herbaceous flowering plants in the family Berberidaceae) flavonoids can have a direct stimulatory effect on neuronal stem cell proliferation and differentiation in a rat cell culture model. Altogether, these observations hold promise for the application of flavonoids to neuroprotection.
SUMMARY
The heterogeneity of TBI, together with prolonged secondary pathogenesis and long-lasting adverse neurological sequelae, makes this disease a challenge to manage. In fact, there has never been a single pharmaceutical that has been successful as a therapeutic agent. Interventions targeting features of the acutely injured brain such as hypoxia and excitotoxicity will differ from those that are directed toward the more chronically injured brain that is characterized in part by the emergence of proteinopathies. Alterations in metabolism early after the injury can result in disorders in the areas of motor function, cognition, and neuropsychiatry. Based on the multifaceted nature of the disease, scientists and clinicians recommend a combinatorial approach to its management. Nutritional interventions should not be viewed as stand-alone therapies but rather as support for other therapies, and can range from the clinical support provision of essential nutrients and energy for recovery from major trauma, to individual nutrients or phytochemicals that may uniquely affect the neuronal repair process.
A number of nutrients, dietary supplements, or specific diets act in biological systems in ways that suggests administration to TBI patients before or after injury could improve patient outcomes. For example, restoring energetics, acting as anti-inflammatory or anti-oxidant agents, or delaying apoptosis could all contribute to faster recovery from TBI. The mechanisms of action of most nutrients are currently not well understood. The committee centered its attention on those compounds shown to act in relevant physiological processes. As an illustration, based upon the clinical success of progesterone and its mechanism of action, it seems logical to focus upon the same clinical goals, that is, to prevent or modulate the expression of inflammatory cytokines (particularly interleukin-6) in the brain while blocking the mechanisms that trigger apoptosis, such as oxidative stress, excess cellular calcium release, and activation of apoptotic genes. The fact that nutrients can affect various pathological responses presents a real advantage in potential interventions. However, when selecting nutritional interventions and conducting reseach, it is essential to consider that the target population is critically ill (see Chapter 17 for other considerations in study designs). Therefore, it is extremely important that adverse events associated with the nutrients be investigated not only in the healthy population, but also in the critically ill. As an example, although probiotics have shown some benefits for TBI, care should be taken when considering administering these to critically ill persons because of potential adverse effects (Besselink et al., 2008).
Based on the expert opinion of the committee and the target areas identified as relevant for nutrition, the following nutritional interventions were identified for review: energy needs for severe cases of TBI, acetyl-CoA, antioxidants, branched-chain amino acids, choline, creatine, ketogenic diets, magnesium, nicotinamide adenine dinucleotide (NAD+), n-3 fatty acids, polyphenols, probiotics, vitamin D, and zinc. The committee searched the literature using the database Medline and employing the parameters shown in Box 4-2. Three of the nutritional interventions (i.e., acetyl-L-carnitine, niacin, and probiotics) initially selected were not included in the report because of insufficient evidence from animal and human studies to reach conclusions about their effectiveness, or concerns for harm. Based on the existence of promising data from animal or human studies on TBI (or the associated conditions listed in Box 4-2), the committee selected the following ten nutritional interventions to review in greater detail: energy needs (Chapter 6), antioxidants (i.e., vitamins E and C) (Chapter 7), branched-chain amino acids (Chapter 8), choline (Chapter 9), creatine (Chapter 10), ketogenic and modified diets (Chapter 11), magnesium (Chapter 12), omega-3 fatty acids (Chapter 13), polyphenols (i.e., flavonoids, resveratrol, and curcumin) (Chapter 14), vitamin D (Chapter 15), and zinc (Chapter 16).
REFERENCES
- Alano, C. C., P. Garnier, W. Ying, Y. Higashi, T. M. Kauppinen, and R. A. Swanson. 2010. NAD+ depletion is necessary and sufficient for poly(ADP-ribose) polymerase-1-mediated neuronal death. Journal of Neuroscience 30(8):2967–2978. [PMC free article: PMC2864043] [PubMed: 20181594]
- Altmeyer, M., and M. O. Hottiger. 2009. Poly(ADP-ribose) polymerase 1 at the crossroad of metabolic stress and inflammation in aging. Aging 1(5):458–469. [PMC free article: PMC2806023] [PubMed: 20157531]
- Arun, P., P. S. Ariyannur, J. R. Moffet, G. Xing, K. Hamilton, N. E. Grunberg, J. A. Ives, and A. M. Namboodiri. 2010. Metabolic actetate therapy for the treatment of traumatic brain injury. Journal of Neurotrauma 27(1):293–298. [PMC free article: PMC2824219] [PubMed: 19803785]
- Bales, J. W., A. K. Wagner, A. E. Kline, and C. E. Dixon. 2009. Persistent cognitive dysfunction after traumatic brain injury: A dopamine hypothesis. Neuroscience and Biobehavioral Reviews 33(7):981–1003. [PMC free article: PMC2806224] [PubMed: 19580914]
- Bazan, N. G. 2006. The onset of brain injury and neurodegeneration triggers the synthesis of docosanoid neuroprotective signaling. Cellular and Molecular Neurobiology 26(4–6):901–913. [PubMed: 16897369]
- Bazan, N. G. 2007. Omega-3 fatty acids, pro-inflammatory signaling and neuroprotection. Current Opinion in Clinical Nutrition and Metabolic Care 10(2):136–141. [PubMed: 17285000]
- Bazan, N. G., V. L. Marcheselli, and K. Cole-Edwards. 2005. Brain response to injury and neurodegeneration— Endogenous neuroprotective signaling. Annals of the New York Academy of Sciences 1053:137–147. [PubMed: 16179516]
- Beauchamp, K., H. Mutlak, W. R. Smith, E. Shohami, and P. F. Stahel. 2008. Pharmacology of traumatic brain injury: Where is the “golden bullet”? Molecular Medicine 14(11–12):731–740. [PMC free article: PMC2527342] [PubMed: 18769636]
- Beniczky, S., M. Jose Miranda, J. Alving, J. Heber Povlsen, and P. Wolf. 2010. Effectiveness of the ketogenic diet in a broad range of seizure types and EEG features for severe childhood epilepsies. Acta Neurologica Scandinavica 121(1):58–62. [PubMed: 19951269]
- Besselink, M. G., H. C. van Santvoort, E. Buskens, M. A. Boermeester, H. van Goor, H. M. Timmerman, V. B. Nieuwenhuijs, T. L. Bollen, B. van Ramshorst, B. J. Witteman, C. Rosman, R. J. Ploeg, M. A. Brink, A. F. Schaapherder, C. H. Dejong, P. J. Wahab, C. J. van Laarhoven, E. van der Harst, C. H. van Eijck, M. A. Cuesta, L. M. Akkermans, and H. G. Gooszen. 2008. Probiotic prophylaxis in predicted severe acute pancreatitis: A randomized, double-blind, placebo-controlled trial. The Lancet 371(9613):651–659. [PubMed: 18279948]
- Boehning, D., R. L. Patterson, L. Sedaghat, N. O. Glebova, T. Kurosaki, and S. H. Snyder. 2003. Cytochrome c binds to inositol (1,4,5) trisphosphate receptors, amplifying calcium-dependent apoptosis. Nature Cell Biology 5(12):1051–1061. [PubMed: 14608362]
- Bramlett, H. M., and W. D. Dietrich. 2004. Pathophysiology of cerebral ischemia and brain trauma: Similarities and differences. Journal of Cerebral Blood Flow and Metabolism 24(2):133–150. [PubMed: 14747740]
- Cekic, M., and D. G. Stein. 2010. Traumatic brain injury and aging: Is a combination of progesterone and vitamin D hormone a simple solution to a complex problem? Neurotherapeutics 7(1):81–90. [PMC free article: PMC2834197] [PubMed: 20129500]
- Chang, E. F., C. P. Claus, H. J. Vreman, R. J. Wong, and L. J. Noble-Haeusslein. 2005. Heme regulation in traumatic brain injury: Relevance to the adult and developing brain. Journal of Cerebral Blood Flow and Metabolism 25(11):1401–1417. [PubMed: 15917748]
- Cole, J. T., C. M. Mitala, S. Kundu, A. Verma, J. A. Elkind, I. Nissim, and A. S. Cohen. 2010. Dietary branched chain amino acids ameliorate injury-induced cognitive impairment. Proceedings of the National Academy of Sciences of the United States of America 107(1):366–371. [PMC free article: PMC2806733] [PubMed: 19995960]
- Dietrich, W. D., and H. M. Bramlett. 2010. The evidence for hypothermia as a neuroprotectant in traumatic brain injury. Neurotherapeutics 7(1):43–50. [PMC free article: PMC2819078] [PubMed: 20129496]
- Doré, S. 2010. Mechanisms of Nutritional Neuroprotection: Neuroprotective action of the flavanol (–)-epicatechin. Presented at the IOM Nutrition and Neuroprotection in Military Personnel Workshop, Washington, DC.
- Dubreuil, C. I., N. Marklund, K. Deschamps, T. K. McIntosh, and L. McKerracher. 2006. Activation of Rho after traumatic brain injury and seizure in rats. Experimental Neurology 198(2):361–369. [PubMed: 16448651]
- Faden, A. I. 2002. Neuroprotection and traumatic brain injury: Theoretical option or realistic proposition. Current Opinion in Neurology 15(6):707–712. [PubMed: 12447109]
- Ghanim, H., C. L. Sia, S. Abuaysheh, K. Korzeniewski, P. Patnaik, A. Marumganti, A. Chaudhuri, and P. Dandona. 2010. An antiinflammatory and reactive oxygen species suppressive effects of an extract of polygonum cuspidatum containing resveratrol. The Journal of Clinical Endocrinology and Metabolism 95(9):E1–E8. [PMC free article: PMC2936054] [PubMed: 20534755]
- Graham, D. I., T. K. McIntosh, W. L. Maxwell, and J. A. Nicoll. 2000. Recent advances in neurotrauma. Journal of Neuropathology and Experimental Neurology 59(8):641–651. [PubMed: 10952055]
- Jain, K. K. 2008. Neuroprotection in traumatic brain injury. Drug Discovery Today 13(23–24):1082–1089. [PubMed: 18848641]
- Kidd, P. M. 2007. Omega-3 DHA and EPA for cognition, behavior, and mood: Clinical findings and structural-functional synergies with cell membrane phospholipids. Alternative Medicine Review 12(3):207–227. [PubMed: 18072818]
- Kidd, P. M. 2009. Integrated brain restoration after ischemic stroke—medical management, risk factors, nutrients, and other interventions for managing inflammation and enhancing brain plasticity. Alternative Medicine Review 14(1):14–35. [PubMed: 19364191]
- Kokiko, O. N., and R. J. Hamm. 2007. A review of pharmacological treatments used in experimental models of traumatic brain injury. Brain Injury 21(3):259–274. [PubMed: 17453754]
- Kondo, K., S. Obitsu, S. Ohta, K. Matsunami, H. Otsuka, and R. Teshima. 2010. Poly(ADP-ribose) polymerase (PARP)-1-independent apoptosis-inducing factor (AIF) release and cell death are induced by eleostearic acid and blocked by alpha-tocopherol and MEK inhibition. Journal of Biological Chemistry 285(17):13079–13091. [PMC free article: PMC2857103] [PubMed: 20177052]
- Laird, M. D., S. Sukumari-Ramesh, A. E. Swift, S. E. Meiler, J. R. Vender, and K. M. Dhandapani. 2010. Curcumin attenuates cerebral edema following traumatic brain injury in mice: A possible role for aquaporin-4? Journal of Neurochemistry 113(3):637–648. [PMC free article: PMC2911034] [PubMed: 20132469]
- Longhi, L., K. E. Saatman, R. Raghupathi, H. L. Laurer, P. M. Lenzlinger, P. Riess, E. Neugebauer, J. Q. Trojanowski, V. M. Y. Lee, M. S. Grady, D. I. Graham, and T. K. McIntosh. 2001. A review and rationale for the use of genetically engineered animals in the study of traumatic brain injury. Journal of Cerebral Blood Flow and Metabolism 21(11):1241–1258. [PubMed: 11702040]
- Love, S. 1999. Oxidative stress in brain ischemia. Brain Pathology 9(1):119–131. [PMC free article: PMC8098220] [PubMed: 9989455]
- Maas, A. I. R., B. Roozenbeek, and G. T. Manley. 2010. Clinical trials in traumatic brain injury: Past experience and current developments. Neurotherapeutics 7(1):115–126. [PMC free article: PMC5084118] [PubMed: 20129503]
- Mammis, A., T. K. McLntosh, and A. H. Maniker. 2009. Erythropoietin as a neuroprotective agent in traumatic brain injury review. Surgical Neurology 71(5):527–531. [PubMed: 18789503]
- Margulies, S., R. Hicks, and B. Combination Therapies Traumatic. 2009. Combination therapies for traumatic brain injury: Prospective considerations. Journal of Neurotrauma 26(6):925–939. [PMC free article: PMC2857809] [PubMed: 19331514]
- Marklund, N., A. Bakshi, D. J. Castelbuono, V. Conte, and T. K. McIntosh. 2006. Evaluation of pharmacological treatment strategies in traumatic brain injury. Current Pharmaceutical Design 12(13):1645–1680. [PubMed: 16729876]
- Marklund, N., K. Salci, A. Lewen, and L. Hillered. 1997. Glycerol as a marker for post-traumatic membrane phospholipid degradation in rat brain. Neuroreport 8(6):1457–1461. [PubMed: 9172153]
- Mattson, M. P., and S. L. Chan. 2003. Calcium orchestrates apoptosis. Nature Cell Biology 5(12):1041–1043. [PubMed: 14647298]
- Mattson, M. P., W. Z. Duan, S. L. Chan, A. W. Cheng, N. Haughey, D. S. Gary, Z. H. Guo, J. W. Lee, and K. Furukawa. 2002. Neuroprotective and neurorestorative signal transduction mechanisms in brain aging: Modification by genes, diet and behavior. Neurobiology of Aging 23(5):695–705. [PubMed: 12392775]
- Moppett, I. K. 2007. Traumatic brain injury: Assessment, resuscitation and early management. British Journal of Anaesthesia 99(1):18–31. [PubMed: 17545555]
- Morales, D. M., N. Marklund, D. Lebold, H. J. Thompson, A. Pitkanen, W. L. Maxwell, L. Longhi, H. Laurer, M. Maegele, E. Neugebauer, D. I. Graham, N. Stocchetti, and T. K. McIntosh. 2005. Experimental models of traumatic brain injury: Do we really need to build a better mousetrap? Neuroscience 136(4):971–989. [PubMed: 16242846]
- Morganti-Kossmann, M. C., L. Satgunaseelan, N. Bye, and T. Kossmann. 2007. Modulation of immune response by head injury. Injury 38(12):1392–1400. [PubMed: 18048036]
- Povlishock, J. T., and D. I. Katz. 2005. Update of neuropathology and neurological recovery after traumatic brain injury. Journal of Head Trauma Rehabilitation 20(1):76–94. [PubMed: 15668572]
- Raghupathi, R. 2004. Cell death mechanisms following traumatic brain injury. Brain Pathology 14(2):215–222. [PMC free article: PMC8096005] [PubMed: 15193035]
- Royo, N. C., S. Shimizu, J. W. Schouten, J. F. Stover, and T. K. McIntosh. 2003. Pharmacology of traumatic brain injury. Current Opinion in Pharmacology 3(1):27–32. [PubMed: 12550738]
- Schouten, J. W. 2007. Neuroprotection in traumatic brain injury: A complex struggle against the biology of nature. Current Opinion in Critical Care 13(2):134–142. [PubMed: 17327733]
- Scudellari, M. 2010. Brain, interrupted. The Scientist 24(7):37, http://www
.the-scientist .com/2010/7/1/37/1/ (accessed July 19, 2010). - Secades, J. J., and J. L. Lorenzo. 2006. Citicoline: Pharmacological and clinical review, 2006 update. Methods and Findings in Experimental and Clinical Pharmacology 28:1–56. [PubMed: 17171187]
- Sen, A. P., and A. Gulati. 2010. Use of magnesium in traumatic brain injury. Neurotherapeutics 7(1):91–99. [PMC free article: PMC5084116] [PubMed: 20129501]
- Shah, Z. A., R. C. Li, A. S. Ahmad, T. W. Kensler, M. Yamamoto, S. Biswal, and S. Dore. 2010. The flavanol (–)-epicatechin prevents stroke damage through the Nrf2/HO1 pathway. Journal of Cerebral Blood Flow Metabolism 30(12):1951–61. [PMC free article: PMC3002885] [PubMed: 20442725]
- Spencer, J. P. E. 2009. Flavonoids and brain health: Multiple effects underpinned by common mechanisms. Genes and Nutrition 4(4):243–250. [PMC free article: PMC2775888] [PubMed: 19685255]
- Stein, D. G., D. W. Wright, and A. L. Kellermann. 2008. Does progesterone have neuroprotective properties? Annals of Emergency Medicine 51(2):164–172. [PubMed: 17588708]
- Stetler, R. A., Y. Gan, W. Zhang, A. K. Liou, Y. Gao, G. Cao, and J. Chen. 2010. Heat shock proteins: Cellular and molecular mechanisms in the CNS. Progress in Neurobiology 92(2):184–211. [PMC free article: PMC2939168] [PubMed: 20685377]
- Sullivan, P. G., J. D. Geiger, M. P. Mattson, and S. W. Scheff. 2000. Dietary supplement creatine protects against traumatic brain injury. Annals of Neurology 48(5):723–729. [PubMed: 11079535]
- Thompson, H. J., J. Lifshitz, N. Marklund, M. S. Grady, D. I. Graham, D. A. Hovda, and T. K. McIntosh. 2005. Lateral fluid percussion brain injury: A 15-year review and evaluation. Journal of Neurotrauma 22(1):42–75. [PubMed: 15665602]
- Thompson, H. J., N. Marklund, D. G. LeBold, D. M. Morales, C. A. Keck, M. Vinson, N. C. Royo, R. Grundy, and T. K. McIntosh. 2006. Tissue sparing and functional recovery following experimental traumatic brain injury is provided by treatment with an anti-myelin-associated glycoprotein antibody. European Journal of Neuroscience 24(11):3063–3072. [PMC free article: PMC2377452] [PubMed: 17156367]
- Unterberg, A. W., J. Stover, B. Kress, and K. L. Kiening. 2004. Edema and brain trauma. Neuroscience 129(4): 1021–1029. [PubMed: 15561417]
- Wendel, M., and A. R. Heller. 2010. Mitochondrial function and dysfunction in sepsis. Wiener Medizinische Wochenschrift 160(5–6):118–123. [PubMed: 20364414]
- Werner, C., and K. Engelhard. 2007. Pathophysiology of traumatic brain injury. British Journal of Anaesthesia 99(1):4–9. [PubMed: 17573392]
- Wu, A., Z. Ying, and F. Gomez-Pinilla. 2006. Dietary curcumin counteracts the outcome of traumatic brain injury on oxidative stress, synaptic plasticity, and cognition. Experimental Neurology 197(2):309–317. [PubMed: 16364299]
- Wu, A. G., Z. Ying, and F. Gomez-Pinilla. 2010. Vitamin E protects against oxidative damage and learning disability after mild traumatic brain injury in rats. Neurorehabilitation and Neural Repair 24(3):290–298. [PMC free article: PMC2824788] [PubMed: 19841436]
- Xu, P., and A. A. Sauve. 2010. Vitamin B3, the nicotinamide adenine dinucleotides and aging. Mechanisms of Ageing and Development 131(4):287–298. [PubMed: 20307564]
- Yao, R. Q., L. Zhang, X. L. Li, and L. Li. 2010. Effects of epimedium flavonoids on proliferation and differentiation of neural stem cells in vitro. Neurological Research 32(7):736–742. [PubMed: 19703337]
- Yi, J. H., and A. S. Hazell. 2006. Excitotoxic mechanisms and the role of astrocytic glutamate transporters in traumatic brain injury. Neurochemistry International 48(5):394–403. [PubMed: 16473439]
- Ying, W., and Z. G. Xiong. 2010. Oxidative stress and NAD(+) in ischemic brain injury: Current advances and future perspectives. Current Medicinal Chemistry 17(20):2152–2158. [PMC free article: PMC3395209] [PubMed: 20423305]
- Yu, S.-W., Y. Wang, D. S. Frydenlund, O. P. Ottersen, V. L. Dawson, and T. M. Dawson. 2009. Outer mitochondrial membrane localization of apoptosis-inducing factor: Mechanistic implications for release. ASN Neuro 1(5). [PMC free article: PMC2784601] [PubMed: 19863494]
- Zafonte, R., W. T. Friedewald, S. M. Lee, B. Levin, R. Diaz-Arrastia, B. Ansel, H. Eisenberg, S. D. Timmons, N. Temkin, T. Novack, J. Ricker, R. Merchant, and J. Jallo. 2009. The citicoline brain injury treatment (COBRIT) trial: Design and methods. Journal of Neurotrauma 26(12):2207–2216. [PMC free article: PMC2824223] [PubMed: 19803786]
- Zanelli, S. A., N. J. Solenski, R. E. Rosenthal, and G. Fiskum. 2005. Mechanisms of ischemic neuroprotection by acetyl-l-carnitine. Annals of the New York Academy of Sciences 1053:153–161. [PMC free article: PMC4454400] [PubMed: 16179519]
- Approach for Selecting Nutritional Interventions: Mechanistic Targets - Nutritio...Approach for Selecting Nutritional Interventions: Mechanistic Targets - Nutrition and Traumatic Brain Injury
- Profile neighbors for GEO Profiles (Select 117857949) (20)GEO Profiles
- PPIB peptidylprolyl isomerase B [Homo sapiens]PPIB peptidylprolyl isomerase B [Homo sapiens]Gene ID:5479Gene
- Gene Links for GEO Profiles (Select 117857017) (1)Gene
Your browsing activity is empty.
Activity recording is turned off.
See more...