NCBI Bookshelf. A service of the National Library of Medicine, National Institutes of Health.
Roundtable on Environmental Health Sciences, Research, and Medicine; Board on Population Health and Public Health Practice; Institute of Medicine. Health Impact Assessment of Shale Gas Extraction: Workshop Summary. Washington (DC): National Academies Press (US); 2014 Dec 30.
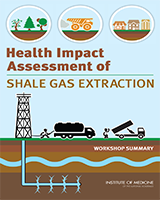
Health Impact Assessment of Shale Gas Extraction: Workshop Summary.
Show detailsThis chapter provides a summary of presentations related to shale gas extraction and implications for air quality. The presentations address how emissions are released during the process, the sources of emissions, the major pollutants that are released, and monitoring efforts. Two presentations discussed specific geographic locations—Texas and Colorado—and their efforts to monitor emissions and assess the potential for health effects. The Colorado presentation describes a health impact assessment (HIA) performed to assess potential risks of unconventional natural gas drilling near a retirement community. The presentations are followed by a summary of the discussion that ensued.
AIR POLLUTION EMISSIONS FROM SHALE GAS DEVELOPMENT AND PRODUCTION
Allen L. Robinson, Ph.D.
Professor, Departments of Mechanical Engineering and Engineering and Public Policy Carnegie Mellon University
Allen Robinson began the presentation by stating that the processes utilized during shale gas development release emissions that have the potential to affect air quality. In general, when emissions are released into the atmosphere, the concentrations of these emissions are highest in the immediate vicinity of the source and decrease as distance from the source increases. In addition, processes can occur in the atmosphere to chemically transform the pollutants or produce new ones. These processes affect exposure, and the dose and response characteristics lead to the health effect ultimately observed in the population. Limiting the focus solely to the upstream part of this paradigm—the emissions—still necessitates consideration of a plethora of sources when assessing the impacts of a process such as shale gas development. There is an entire production chain to examine, and the release of emissions can occur at every point in the production chain, Dr. Robinson said.
There are many sources of air pollutants along the shale gas development chain. Hydraulic fracturing is a single part of the shale gas development operation that occurs for a short period of time. Other activities associated with shale gas development are sources of air pollutants as well. Example of other important sources include
- site preparation, including building roads and clearing pads,
- drilling the well,
- truck traffic to deliver and remove materials and wastes to and from the site,
- separation and treatment operations (remove acid gases, remove water from natural gas and separation of natural gas from other hydrocarbons),
- compressor stations that pressurize natural gas in gathering and transport pipelines,
- flaring that burns off contaminated, noncommercial gas,
- fugitive emissions that escape unintentionally from cracks or leaks, and
- blowdown and venting operations.
The combination of all these activities creates air pollution, and the question that needs to be addressed is what the net effects are of these aggregate emissions from all of the activities associated with natural gas development and production on air quality.
Types of Air Pollutants Associated with Hydraulic Fracturing
Natural gas development and production emits criteria pollutants as defined by the Clean Air Act (EPA, 2012). Nitrogen oxides (NOx) and volatile organic compounds (VOCs) are associated with oil and gas development; in the presence of sunlight, these may react to form ozone and contribute to regional air problems. Regional chemical transport modeling has predicted that ozone may be of particular concern. Nitrogen dioxide and particulate matter (PM2.5) emissions are also worrisome, but may be more of a local than a regional issue.
Hazardous air pollutants or air toxics are another category of pollutant that is emitted with shale gas development and production. Many operations necessary for oil and gas development use diesel-powered engines, which emit diesel particulate matter. Furthermore, natural gasfired engines can be significant sources of formaldehyde, which is also a secondary pollutant. Aromatics (e.g., benzene and toluene) and other VOCs can be released during shale gas production.
Fugitive emissions released during shale gas extraction are also composed of greenhouse gases such as methane. Black carbon from diesel fuel combustion impacts climate. How the benefits of natural gas shift when emissions that directly impact climate change are factored in is an issue to be examined.
Dr. Robinson discussed Figure 5-1, which lists the sources of emissions described above, categorizes them as major or minor sources of pollution, describes the pollutants they emit, and assesses the quality of data. (The values should be considered the “potential to emit” because particulate filters and other technologies, if implemented effectively, can reduce the contributions of these sources.) He noted that there is reasonable understanding of the emissions from diesel-powered engines (e.g., drill rigs, fracturing pumps, and truck traffic). There is less knowledge about emissions from other sources, for example, completion venting, blowdown venting, and fracturing ponds. There may be an increase in knowledge as the field moves to more controlled “green completions” that significantly reduce fugitive emissions of methane and other gases. During the gas production phase, sources such as compressor stations, heaters and dehydrators, condensate tanks, fugitive emissions, and pneumatics emit air pollutants. Some sources have well-defined emission points, and others do not; for most, more data are needed to accurately assess their impacts. Dr. Robinson noted that the main concern is what the net effect is when these pollutants mix in the atmosphere.

FIGURE 5-1
Sources of emissions. NOTE: NOx = nitrogen oxide, PM = particulate matter, VOC = volatile organic compound. SOURCE: Robinson, 2012.
Spatial Scale of Air Pollution
Dr. Robinson commented on the need to consider the spatial scale of air pollution. The air acts as an integrating medium—the emissions from many sources mix to impact air quality and produce exposures. There is much interest in local exposure because there are usually homes near gas wells, and emissions from drilling activities that have the potential to affect local residents. But, from an air quality perspective, he stressed, it is imperative to also think at the level of field exposure. As well pads become concentrated in a small area, the emissions from the individual activities of each are integrated and can alter air quality. Finally, the regional scale needs to be considered. Individual wells may cover a large spatial distribution, and as their numbers increase, they can have a regional impact. This means that NOx and ozone may not only affect local homes, but may also impact cities that are farther away. For example, Marcellus Shale activities have the potential to affect ozone concentrations in Philadelphia. One way of thinking about regional impacts, Dr. Robinson suggested, is as a massive refinery distributed hundreds or thousands of square miles. From a regional perspective, it is very challenging to fully comprehend the impacts on air quality due to shale gas development.
Another point to consider is where air quality monitoring currently occurs. There is a large network of state and local air monitoring stations that are deployed by state and local agencies to monitor air quality. Selection of sites for stations is population-based, with many stations being located in the Northeast corridor; there is reasonable justification for sitting stations in this fashion. However, there are not many sites in more rural, less populated areas, where much of the gas development is occurring. A monitoring strategy to understand the air quality impacts of shale gas developments in less populated areas, such as in the Marcellus Shale region, is needed. It is possible to make measurements for shortterm studies by setting up temporary monitoring stations downwind of well sites. This will indicate very coarsely what the air quality impacts might be. But these types of assessments are sensitive to meteorological and other factors, making it difficult to quantify the true air quality impacts. Furthermore, because of the dearth of permanent monitoring stations in rural areas, there is limited background baseline data, so it is impossible to track the changes in air quality over time. In addition, there is pressure to reduce monitoring stations—not to establish new ones—and this will not aid in ameliorating the spotty nature of the data concerning the impacts of rural shale gas production on air quality.
Predicting Future Trends in Emissions
Dr. Robinson underscored the need to better predict the impact of emissions associated with shale gas production. Carnegie Mellon is developing an emission inventory for chemical transport modeling to predict the regional impacts of Marcellus Shale activities on air quality. The emission inventory covers the states of New York, Pennsylvania, and West Virginia, but the modeling of impacts considers the entire Eastern United States.
In the Marcellus region, new natural gas development has rapidly expanded. There were no Marcellus wells in the mid-2000s. Currently, approximately 1,000 wells are being drilled per year. The future projections are imprecise, but substantial development will certainly continue into the future—perhaps 3,000 wells drilled per year by 2020 (Considine et al., 2010). The associated activities (e.g., drill rigs, hydraulic fracturing pumps) are going to be contributing significant emissions to the area for the foreseeable future. The exact amount depends strongly on the price of natural gas.
Emissions from compressor stations and processing facilities are tied to the amount of gas produced (not necessarily the number of wells drilled). There is a range of estimates of future gas production, which is also expected to increase. By 2020, there could be 15 billion cubic feet per day (bcfd) produced, where currently there is less than 5 bcfd (Considine et al., 2010; U.S. Energy Information Administration, 2013). These activity predictions are important because they factor into the analysis of future emissions.
Dr. Robinson stated that there is also uncertainty in the underlying emissions data. For the diesel-powered sources, there is literature on emissions data and on duty cycles. These data can be used to build reasonably robust estimates. For process-level data or activities such as pneumatics, there is more uncertainty. To estimate these emissions, there are a number of parameters to use. There is the emission factor (which is the rate of production of the pollutant), operations characteristics (such as power), the size of the source, and the amount of time the source is functioning. These values can vary from basin to basin and operator to operator, so they carry some degree of uncertainty, but, despite that challenge, it is possible to account explicitly for that uncertainty in the estimation of emissions.
In a Monte Carlo simulation, probability distributions for factors that have a high degree of uncertainty are used to calculate a range of possible emission levels. For example, ranges of emission factors for drill rigs, hydraulic fracturing pumps, and other sources can be obtained from the literature. There is also increasing amounts of data on how devices are operated in the field. These distributions have a level of uncertainty that can be incorporated into the analysis. Dr. Robinson noted that this type of analysis explicitly acknowledges uncertainty and accounts for it formally, instead of hiding behind it and using it as an excuse for inaction.
These analyses have revealed that aggregate emissions are important. The amount of NOx emissions due to Marcellus Shale is estimated to be substantial: in 2009, 58 tons per day of NOx were emitted, and by 2020, this is expected to increase to 129 tons per day. Currently Marcellus sources are responsible for a few percent of the regional NOx emissions, but, by 2020, this is expected to increase to 12 percent (Roy et al., 2013). Existing regulations are expected to reduce NOx emissions from power plants and diesel engines. However, as the activities associated with development and production grow; the associated emissions may undermine the gains from regulations and have impacts in the region.
It is possible to do an analogous calculation for VOC emissions. A similar trend is observed—the tonnage of emissions is significant and will increase over time. For VOCs, local effects must be examined as well because the type of gas—wet or dry—varies with location. Wet gas has a higher fraction of condensates and its production releases more VOCs. In the Marcellus region, wet gas is found in West Virginia and southwestern Pennsylvania. The properties of the gas alter its emissions and thus affect the exposure of the surrounding community to air toxics (The Nature Conservancy, 2010).
The analysis also shows that diesel particulate matter and other air toxics may be important components of air pollutants, which again, is predicted to increase. In 2009, approximately 2 tons per day of diesel particulate matter were released, and this is predicted to double by 2020 (Kemball-Cook et al., 2010). Emissions from on-road diesel activity are expected to decrease because diesel particulate filters are now required (EPA, 2006). Combining this regulation with the new, sizeable activity from gas development means that the fraction of emissions due to Marcellus activities is expected to increase.
Regarding ozone, model simulations for the Haynesville Shale in Louisiana and Texas have shown a perturbations as large as 10 parts-perbillion (ppb) in the maximum-daily 8-hour average ozone level for high emission scenarios (Kemball-Cook et al., 2010). Considering that the current air quality standard for ground-level ozone is 75 ppb, this disturbance is a significant increase and an important regional impact.
Dr. Robinson emphasized that effective control measures do exist for emissions from shale gas activities. For example, for compressor stations, technology such as oxidation catalysts, selective catalytic reduction, and stoichiometric combustion engines with three-way catalysts can reduce the emissions of NOx, VOCs, and formaldehyde. For drill rigs and hydraulic fracturing pumps, it is possible to use selective catalytic reduction and diesel particulate filters to reduce emissions. Fuel switching from diesel to natural gas is also an option, and will reduce diesel particulate matter (but not necessarily NOx emissions). Finally, removing waste water from drill sites with pipe networks instead of with trucks can reduce emissions. A new oil and gas regulation is going to require green completion, which is predicted to reduce VOCs by 95 percent (EPA, 2006). Flaring also will substantially reduce emissions (by 51 to 84 percent) (EPA, 2006). Vapor recovery units on condensate tanks can reduce emissions by 91 to 95 percent (EPA, 2006). Dr. Robinson noted that society needs to decide what level of emissions is acceptable, and then implement various rules to achieve the necessary reductions. All of the technology currently exists.
When discussing air pollution and natural gas, it cannot be overlooked that natural gas development provides some air quality benefits to end users, Dr. Robinson stated. Natural gas is a cleaner burning fuel and emits less NOx, sulfur dioxide, PM, and carbon monoxide (Lash and Engelder, 2009). For example, switching from coal to natural gas can improve air quality around power plants. Therefore there are many potential air quality benefits associated with greater use of natural gas. However, one cannot ignore the challenges on the development and production side; it is a matter of deciding what the appropriate level of control is, and then implementing strategies.
AIR QUALITY IMPACTS OF NATURAL GAS OPERATIONS IN TEXAS
Michael Honeycutt, Ph.D.
Director, Toxicology Division Texas Commission on Environmental Quality
Michael Honeycutt began his presentation by describing several shale formations in Texas, three of which are actively being drilled. The Haynesville Shale is in east Texas; the Barnett Shale is in north Texas; and the Eagle Ford Shale is in south Texas. Because shale gas development can result in air emissions, the Texas Commission on Environmental Quality (TCEQ) has been monitoring these areas for the past 6 years.
Under the Clean Air Act, regulators have categorized air pollutants as either criteria air pollutants or noncriteria air pollutants (EPA, 2012). The criteria pollutants are chemicals with national ambient air quality standards—ozone, particulate matter, lead, SOx, NOx, and carbon monoxide. The noncriteria air pollutants are those that fall outside this category.
Dr. Honeycutt noted that there has been exponential growth in gas development within the Barnett Shale over the past decade, but it is now starting to level off. Ozone is the only criteria pollutant associated with oil and gas activities for which the Dallas–Fort Worth (DFW) region is in violation. Despite the exponential growth in the number of wells (from almost zero in 1993 to more than 14,000 in 2011), the 8-hour ozone design value is trending down, from 106 ppb in 1995 to 86 ppb in 2010 (see Figure 5-2) (TCEQ, 2013). (The 8-hour ozone design value for the DFW nonattainment area is 85 ppb.) There was a slight increase in 2011, but that was most likely due to a drought, he suggested.

FIGURE 5-2
Dallas–Fort Worth area ozone design values and Barnett Shale production. SOURCE: Honeycutt, 2012.
The Monitoring Process in Practice
Dr. Honeycutt described the types of monitoring that are used to monitor emissions. Observational data on levels of criteria and noncriteria pollutants (such as the instance described above) are collected using fixed-site and short-term monitoring methods. TCEQ carries out two types of long-term fixed-site monitoring. One type uses a fixed-site canister to capture air samples. A stainless steel, clean canister is put under vacuum and attached to a timer and flow controller. On the day that samples will be taken, a valve opens at midnight, and air is pulled through the sampler and into the canister. This continues for a 24-hour period. At midnight the next night, the sampler shuts off. A technician travels to the site the following day, takes out the old canister, and puts in a new one. The sampled canister is shipped to Austin to be analyzed by the toxicology lab. Six days later, the monitor takes another 24-hour sample. This type of monitoring station is permanent and provides a 24-hour sample every sixth day.
The other type of long-term sampling is fixed-site auto gas chromatography (AutoGC). This sampler is housed in a trailer. AutoGC takes an hourly sample; of every hour, 40 minutes are used to collect a continuous air sample, 10 minutes are needed to run the GC analysis, and 10 minutes are required to prepare for the next air sample. This machine operates 24 hours a day and 365 days per year; it collects samples 22 hours per day, 1 hour is devoted to a calibration curve, and the other hour is for a quality assurance/quality control sample. The two control steps are alternated so that the same 2 hours are not omitted each day. Because it provides data continuously, AutoGC provides a wealth of information.
AutoGC can be a fixed-site monitor, but, when necessary, mobile laboratories can be equipped with this technology. These laboratories can be placed right on the property line of oil and gas facilities. Investigators can be out in the field for several weeks at a time collecting samples on many types of chemicals with these monitors.
Dr. Honeycutt reported that a monitor in Longview, Texas, in the Haynesville Shale, detected elevated concentrations of benzene. Benzene is an air toxic that TCEQ is monitoring closely. Benzene is the chemical whose measured concentration is closest to what is considered an acceptable level. Every other chemical that is currently being monitored is further below its acceptable level compared to benzene.
The monitor for this site had been functioning since 1998. Dr. Honeycutt noted that in 2005 there were some slight increases in the annual average benzene concentration, and in 2007 it really climbed. It was above the TCEQ long-term screening value of 1.4 ppb.
This monitor is located 1,100 feet to the northeast of a new oil and gas facility. The facility had only been constructed the year before the monitor started detecting elevated levels of benzene. TCEQ explored the facility and collected air samples. They found that some of the storage tanks were not designed properly. There were significant emissions from those tanks (benzene levels of 1,100 ppb were measured in their vicinity). These benzene leaks were being picked up farther away by the permanent monitoring station. TCEQ worked with the responsible operator to figure out what was going on and to fix it. The next year, benzene levels dropped below 1.4 ppb, and they are still trending downward.
This case underscores a concern of TCEQ about air quality and gas developments. There are many gas developments in Longview, Texas—about one every 0.25 mile. The well immediately south of a monitor was the one to which a problem was traced. TCEQ may have been fortunate to have had a monitor in exactly the right location to pick up on the sole source of benzene emissions in the town. But there was also the possibility that these issues were widespread, and there were no long-term monitors near the rest of the wells to alert TCEQ to problems.
To determine how widespread problems were, TCEQ needed to quickly evaluate many shale gas development wells. To carry out this short-term monitoring and evaluation, TCEQ mounted the GasFindIR™ camera (especially developed for detection of gas leaks) on a helicopter. GasFindIR™ reveals VOC emissions such as methane—they cannot be seen by the naked eye, but, on camera, they appear as dark, billowy smoke. TCEQ has conducted flyovers of the Barnett Shale and Eagle Ford Shale. It costs about $100,000 to conduct a flyover, but it is the best money spent in terms of reducing emissions. Using this camera from a helicopter allows thousands of wells to be surveyed at a time. After the footage is examined, TCEQ can approach the well operators and inform them of a problem. But, finding a problem is atypical. Of the 5,000 storage tanks surveyed in the Barnett Shale, only 88 had significant hydrocarbon emissions.
There are also handheld GasFindIR™ cameras that make it possible to screen many facilities quickly. They are slightly bigger than home camcorders, and field investigators can walk around a facility with them. Since August 2009, TCEQ has surveyed 2,122 gas development sites using GasFindIR™ cameras, and, at 2,078 of these sites, handheld VOC samplers were also used. Whenever there is something of interest, an instantaneous or short-term (30 minutes to 1 hour) canister sample can be taken. On the basis of observations with these instruments, 1,126 canister samples have been collected and analyzed for VOCs. There are other VOCs that TCEQ assesses as well, and this is possible with other instruments that detect nonmethane hydrocarbons. Short-term samples also have been collected and analyzed for carbonyls, NOx, and sulfur compounds.
Indications from Short-Term Monitoring
Dr. Honeycutt provided an overview of findings from short-term monitoring. He said that less than 5 percent of the more than 1,100 VOC canister samples mentioned above exceeded a short-term, health- or odor-based air monitoring comparison value (AMCV).1 Texas regulates chemicals for odor, and some VOCs, such as isobutane and isopentane, have been detected above an odor-based AMCV. Some samples (e.g., benzene and carbon disulfide) have been elevated above a short-term health-based AMCV. Most of these were discovered when TCEQ initiated its monitoring program. The actions taken by TCEQ since then have decreased the number of residents’ complaints of odor.
TCEQ continues to receive some citizen complaints of odor and irritation, and investigators are experiencing similar symptoms in the field. These complaints are thought to be related to a glycol degradation product. TCEQ researchers are trying to figure out what could possibly be emitted with the aim of designing sampling and analysis techniques to monitor these emissions in the future, Dr. Honeycutt said.
Indications from Long-Term Monitoring
Dr. Honeycutt also provided an overview of results from long-term monitoring. Six million to 7 million people live in the DFW metro area (U.S. Census Bureau, 2013). There are about 15,000 wells in the Barnett Shale geologic formation. There are seven fixed-site canister samplers, five fixed-site AutoGC monitors, and two sites where they are co-located (for the purpose of comparing the data between methodologies). There are pros and cons to both types of samplers. The target analyte list for the canister sampler includes about 85 chemicals. The target analyte list for the AutoGC sampler includes only about 55 chemicals. But the hourly aspect of the outputs from the AutoGCs provides more data with which TCEQ can work. The state is prepared to set up five more AutoGC monitors, but it takes time to select an appropriate site for the monitoring station. It is expected that the amount of data available is going to grow exponentially in the near future.
Despite the massive growth in the number of wells, there is no indication from the long-term monitoring of benzene that there is a health risk. (Recall that benzene is the chemical closest to exceeding its acceptable level and is therefore considered the risk driver.) The newer stations are aptly situated both to capture community exposure and to monitor sources. Based on the monitoring data, there is nothing that concerns TCEQ on an airshed level; all levels of benzene are below the 10-5 risk level2 used by the U.S. Environmental Protection Agency (EPA). In fact, some of the lowest monitored levels of benzene across the state are in the Barnett Shale—other parts of Texas have higher benzene concentrations, Dr. Honeycutt said.
In the town of Dish, Texas, many residents were concerned about the effects of shale gas development. The state health department visited the town in 2010 to collect biological samples from 28 residents. The health department examined VOCs in blood and VOC breakdown products in urine, and took samples of tapwater. They compared these data to National Health and Nutrition Examination Survey data and concluded that community-wide exposures from gas wells or compressor stations have not occurred in the sample population.
The results of the analyses of all of TCEQ’s samples are online in the form of an interactive map.3 It is possible to click on a region of the map to determine the concentrations found there, and it is also possible to enter an address to find the data from the nearest station. According to Dr. Honeycutt, this is a great public relations and communications tool.
TCEQ’s Current Activities
Dr. Honeycutt concluded his remarks by noting that the issues TCEQ did document were found early in the monitoring process. Almost all of those were human and mechanical failures on the part of the companies. Corrective actions for those—tightening valves, replacing gaskets, closing hatches—were easy to implement. TCEQ is still actively executing compliance investigations with handheld monitors, but when an issue is found, there is usually an easy fix for the problem. TCEQ is also implementing new rules for best management practices. It is conducting outreach and attempting to educate well operators and the public. For the well operators, TCEQ instructs them about the differences between percentages and parts per billion as they pertain to monitoring emissions. For the public, they host open houses to disseminate information about shale gas development and monitoring activities. Transparency and citizen access to information is important; therefore all data are available on TCEQ’s website.
AIR POLLUTION EXPOSURE AND RISK NEAR UNCONVENTIONAL NATURAL GAS DRILL SITES: AN EXAMPLE FROM GARFIELD COUNTY, COLORADO
John Adgate, Ph.D., M.S.P.H.
Professor and Chair, Department of Environmental and Occupational Health
Colorado School of Public Health
John Adgate began his presentation by describing the term “unconventional natural gas drilling” as the cradle-to-grave process of developing a well and extracting the gas resources. He explained that in response to a citizen request, the Colorado School of Public Health performed a health impact assessment (HIA) on the potential risks of unconventional natural gas drilling (Witter et al., 2011). Natural gas well sites were planned for the area around the retirement community of Battlement Mesa in Garfield County, Colorado. Residents wanted to know what the minimum safe distance was—how close could a drilling site be to their homes and not pose any health risks. HIA is an apt tool for evaluating risk in a situation such as this. Dr. Adgate pointed out that the aim of his presentation was to elaborate on some of the key questions around HIA, and how HIA is used in the public process.
Dr. Adgate provided some context about Garfield County in Colorado. A unique feature of Colorado is that in many locations there is a “split estate,” which means that the people who live on the land and the people who possess the mineral rights for the land are often two different parties. A split estate often creates interesting contrasts and pressures when the mineral rights owners want to develop their resources. Part of the conflict in Battlement Mesa, Garfield County, arose from the split estate issue.
There are about 5 million citizens in the state of Colorado and approximately 80 percent of them live east of the Rocky Mountains. Garfield County is in the western part of the state, and has a population of about 50,000. Dr. Adgate noted that in 2010, Garfield County issued 1,806 drilling permits, making it the county that granted the second highest number of permits (Weld County issued the most, at 1,854 permits). Currently, there are more than 8,000 natural gas wells in Garfield County. Across the state, natural gas wells are becoming more common, and development is starting to encroach on populated areas—thus, increasing citizen interest in the effects of drilling on the environment and human health.
Colorado is a very dry state with interesting meteorological patterns. In Garfield County mornings typically bring rising warm air, with subsequent evening cooling leading to downslope winds. Dr. Adgate explained that in Garfield County most of the gas development is occurring along the valley floor, and these weather patterns have implications for what people are exposed to in and around these sites. In addition, the Colorado River bisects Garfield County, and is a source of drinking water for many people. A major interstate also runs through the valley, and it is a source of some of the same air toxics that are associated with natural gas drilling.
The HIA in Garfield County
Dr. Adgate discussed the process used to conduct the HIA. Garfield County has long-term air monitoring stations operated by their Department of Public Health. These stations collected canister air samples over 24-hour periods that were analyzed in EPA-certified labs. The lab quantified 78 nonmethane VOCs. Overall, there were 163 ambient air samples; one was collected every 6 days over the span of almost 3 years. These samples were used to characterize ambient air levels in the natural gas development area between 2008 and 2010; this was the period over which production peaked and then started to wane.
To collect additional data for the HIA, representatives of Garfield County Public Health staff visited a well site to conduct air sampling. Surrounding the well site was the supporting infrastructure that develops over time, and farther away were previously established agricultural zones, ranches, and beehives. There were no houses within 1,000 feet of this particular operation. The team noted that hatches were open on the tanks that hold the wastewater after it flows out of the well, and they were able to smell odors.
To characterize the peak emissions of the well development process Garfield County Public Health staff collected samples from the period of flowback and well completion while at the well site. The flowback process is when the water used for fracturing exits the well, usually carrying hydrocarbons. There were 24 samples collected at distances between about 150 and 500 feet from these well sites, and they were used as part of this human health risk assessment process.
To develop risk estimates, many questions needed to be addressed. How long are these wells in place? How long does it take to develop a well? How many wells are on a pad? How many pads are there in a community? To address some of these, EPA methods were used to estimate risks over two durations: subchronic (short-term effects) and chronic (longer-term effects). The subchronic exposure scenario assumed a 20-month period,4 because this is the length of time a resident would be exposed to pollutants from well development. The chronic exposure scenario assumes a 30-year period because natural gas could be produced from a mature well for 20–30 years.
Dr. Adgate noted that to address uncertainty in these estimations, many assumptions were made during these calculations. One assumption concerned the two distance-related categories of exposure. Exposures were divided into “near” and “far” based on citizen complaints about odor. Essentially, those who could smell gas well activities and lived less than half a mile away were classified as near to a well. Everyone else was classified as living far from the well. Also, the analysis used “default” options that assume reasonable maximum exposure and are designed to be health protective. They presume that a resident remains in the town 24 hours per day, 350 days per year, which necessitates using the upper bound (95 percent upper confidence limit) of mean concentration estimates for the various air toxics.
To conduct the human health risk assessment, researchers from the Colorado School of Public Health used standard EPA methodology. The researchers combined existing data with interesting new data that had not yet been reported in the scientific literature. The longer-term and peak data just described went into creating exposure scenarios. There were four scenarios: chronic-far, subchronic-far, chronic-near, subchronic-near. Both far scenarios relied only on the ambient air data from the permanent monitoring stations. The subchronic-near scenario used only the 24 air samples from the peak well-completion phase. The chronicnear scenario used the time-weighted average of the peak wellcompletion samples (a duration of 20 months) and ambient air samples over a duration of 30 years.
To quantify noncancer health risks, hazard indexes (HIs) were used. The HI is the sum of all applicable semiquantitative hazard quotients (HQs). For each compound detected, an HQ is computed, which is the ratio of the estimated exposure to the reference concentration (RfC). RfCs are values that are suggestive of exposures at which noncancer health effects may occur, and are catalogued in the EPA’s Integrated Risk Information System. This system provides both chronic and subchronic RfCs, and each RfC was applied in the appropriate scenario. Dr. Adgate said that the meaningful question is whether the HI is greater than 1. If it is less than 1, it is possible to be confident of few health effects. If it is greater than 1, then risks to health are more likely.
The process of estimating cancer risk is distinct from estimating noncancer health effects. The long-term average exposure for each carcinogen was estimated for a 30-year time period using the ambient air sampling data. This exposure was multiplied by an inhalation unit risk value, which indicates potency of the carcinogen, to compute the lifetime excess cancer risk. The risk level was summed across the different carcinogens that were detected to compute cumulative lifetime cancer risk. The lifetime cancer risk is an indication of the number of excess cancers in a population of 1 million people. The criterion used to evaluate risk from carcinogens is whether the risks are greater than 1 in 1 million (i.e., is there more than 1 excess cancer within a population of 1 million people).
Findings of the HIA
Dr. Adgate reiterated that there were four different scenarios used to calculate HIs: chronic-far, subchronic-far, chronic-near, subchronic-near. For noncancer health effects, it was determined that the HI is greater for those living closer to well sites (see Figure 5-3), although most HIs did not reach a level of concern. The HI for chronic-far was 0.4, and the HI for chronic-near was 1. The HI for subchronic-far was 0.2, and the HI for subchronic-near was 5 (McKenzie et al., 2012). Only the HI for subchronicnear was above 1—which is above the level of concern. The next question is what can be done about this, that is, how can exposures and risk be reduced.

FIGURE 5-3
Hazard indexes by duration of exposure and distance from source. SOURCE: Adgate, 2012.
Instead of averaging all HQs to find a single HI, it is possible to categorize toxics by health outcome and calculate an HI for neurological effects only, or respiratory effects only, etc. For the subchronic-near exposures, the data were parsed on the basis of health end points. For neurological effects, the HI was 4. For respiratory effects, the HI was 2. For hematological effects, the HI was 3. For developmental effects, the HI was 1 (see Figure 5-4) (McKenzie et al., 2012). The first three health end point categories are in the range of concern, Dr. Adgate noted, where action likely should be taken to reduce exposure. For example, new controls could be mandated to be put in place to limit citizens’ exposures.

FIGURE 5-4
Hazard indexes by health end point: Near wells, 20-month exposure scenario. SOURCE: Adgate, 2012.
The biggest contributor to the hazard index was trimethylbenzenes, which were responsible for 46 percent of the estimated risk. The next biggest contributors were aliphatic hydrocarbons (21 percent) and xylenes (15 percent) (McKenzie et al., 2012). Adgate noted that benzene was a relatively small contributor, at only 5 percent. So, a range of compounds is responsible for the health effects, and a fair amount is known about these from a toxicological standpoint. These data may inform some of the practices aimed at reducing risks and health effects.
Dr. Adgate noted that the estimated cancer risks for a 30-year exposure were in the range that does not typically warrant concern. There was a 6 in 1 million risk for residents living far from a well, and 10 in 1 million risk for residents living close to a well. This is above the 1 in 1 million risk level, but below the level at which the EPA typically requires remediation. Overall, concentrations were similar to or higher than those observed in many urban areas. Benzene, although it contributed little to the noncancer health effects, was, along with ethylbenzene, the main risk driver for cancer risk.
Residents living near well completion activities are potentially exposed to substantial levels of air toxics. Subchronic noncancer cumulative and end point specific hazard indexes are greater than 1 for residents living near well pads. Also, estimated cancer risks and chronic noncancer hazard indexes are greater for these residents living near the well pads, but are within a generally acceptable range.
There are many uncertainties and limitations within this study, Dr. Adgate noted. The sample size was small, using only 24 samples to assess 78 compounds collected from 150 to 500 feet around the well source. In general, limited data exist on emissions around well completion sites. In addition, nonmethane pollutant emissions appear to vary substantially by field type, number of wellheads, completion process, and controls used. Only a limited suite of volatile compounds was explored here, which excluded other primary or secondary pollutants (e.g., aldehydes, diesel exhaust, etc.). In summary, Dr. Adgate said that short-term exposures are probably important to address and that prevention strategies should be directed at minimizing exposures during well completion activities to reduce potential subchronic noncancer risks.
AIR QUALITY RESPONDENT
Bernard D. Goldstein, M.D.
Professor Emeritus, Department of Environmental and Occupational Health
Graduate School of Public Health, University of Pittsburgh
Dr. Goldstein began his commentary by noting that in southwestern Pennsylvania, Shell is constructing a plant to manufacture ethylene through the process of cracking, or heating hydrocarbons to induce unsaturation. It is currently in the middle of the permitting process. During this process, the plant’s potential for emitting ozone precursors will be examined.
He noted that Dr. Robinson’s point about emissions being an aggregate issue is extremely relevant to the southwestern Pennsylvania case. This one plant, which will have to go through all of the ozone considerations in a region that has borderline-elevated ozone levels may produce fewer emissions than all of the Marcellus Shale wells combined. But each individual Marcellus Shale well does not have to go through the ozone permitting process because it is a small source of emissions. There is an “emissions threshold,” and below this threshold, no regulation is required.
This threshold also exists for the Emergency Planning and Community Right-to-Know Act (Executive Order No. 12,969). This law states that reports must be made to the community if the amount of a chemical stored in or released from a factory or other such structure exceeds a certain limit. This is especially relevant for emergency management, so that firemen and other first responders can be educated about the risks of these chemicals in case there is ever a major problem. But not one of the individual Marcellus Shale sites exceeds the threshold to initiate this kind of communication or training. Again, that is an issue of the aggregate versus the individual, which Dr. Robinson laid out well.
Ozone and formaldehyde are two key examples of emissions that result from natural gas development and use, and that may affect health. According to Dr. Goldstein, the ozone issue has a particular irony to it, in that if the ozone standard is exceeded, or an area starts to approach nonattainment, a state implementation plan needs to be developed. To meet the ozone standard, this plan will likely mandate restriction of some industrial development. The irony is, of course, that the Marcellus Shale is being sold to Pennsylvanian communities as, if you will, industrial development.
Formaldehyde is another interesting issue. Formaldehyde is a onecarbon aldehyde. Methane is a one-carbon hydrocarbon. Burning methane is pretty clean. The one thing of concern with incomplete combustion of methane is formaldehyde.
Regarding emissions of pollutants such as ozone and formaldehyde, what the public health community is requesting is access to information so that health studies can begin. The public health community is concerned about an impact of shale gas drilling on health. It is recognized that there may be an impact on the industry due to the health community’s evaluation of these compounds’ potential adverse health effects.
Current research into ozone health effects is likely to reduce the ozone standard because of findings relating ozone exposure to premature mortality. There is a similar battle over formaldehyde. Based on recent research it appears that formaldehyde induces leukemia in humans. Research on formaldehyde will affect how natural gas is used, but it needs to be thought of as a bidirectional issue, and the public health community needs to continue to communicate—to advocate for controls that protect health.
Finally, Dr. Goldstein emphasized, it is important to make a distinction between the two types of air pollutants that have been discussed. One kind includes pollutants that no one wants in the air, that is, NOx, ozone, and formaldehyde. The other type includes pollutant that industry can sell, be it methane or benzene. What is evident is that, over time, industry does a better job recovering products that they can then sell in the market, particularly if companies are pushed to emit less. So, a distinction between these two types of pollutants needs to be made in terms of strategies to reduce emissions.
DISCUSSION
During the discussion period a question was raised about fugitive methane and motivations to reduce it. Dr. Robinson responded that there are motivations to try to reduce fugitive methane. He noted that fugitive methane is associated with what is called completion venting, which is one of the last stages in bringing a well online. Very high estimates of fugitive methane imply that there is venting into the atmosphere and steps to be more environmentally friendly are not being taken. Estimates of fugitive methane are unclear, but are said to range as high as 7 percent; however, 2 percent is about the level where people begin to become concerned about the impact on climate. Climate is the motivator to reduce fugitive methane.
Another audience member raised a question about geographic air pollution and monitoring. The participant stated that NOx, VOCs, and methane are not ozone but atmospherically transform into ozone. There is some research that has looked at how NOx can scavenge ozone where it is being released, and that ozone concentrations are not necessarily highest where these ozone precursors are emitted. The participant asked the panel whether this fact had any implications for where to measure ozone. Dr. Robinson responded that this phenomenon is known as the NOx titration effect, where weekend levels of ozone are higher than on weekdays despite the fact that emissions are typically lower on weekends. This phenomenon will change the spatial pattern of what is going on, and so it is important to keep that in mind. Dr. Robinson also pointed out that considering secondary pollutant formation is also important. This depends on the chemical state of the atmosphere, that is, how much NOx there is relative to ozone. The effect of additional NOx will depend on geography and sensitivity. Modeling suggests that the northeast United States is more NOx sensitive and that additional NOx would have a bigger impact. Other regions of the country may be more VOC sensitive. So this factor should be taken into consideration when setting emission controls.
REFERENCES
- Adgate J. Air pollution exposure and risk near unconventional natural gas drill sites: Examples from Garfield County, Colorado. PowerPoint presentation at the Institute of Medicine workshop on the Health Impact Assessment of New Energy Sources: Shale Gas Extraction; Washington, DC: 2012.
- Considine TJ, Watson R, Blumsack S. The Pennsylvania State University, College of Earth and Mineral Sciences, Department of Energy and Mineral Engineering; 2010. [May 30, 2013]. (The economic impacts of the Pennsylvania Marcellus Shale natural gas play: An update). http:
//marcelluscoalition .org/wp-content /uploads/2010/05/PA-Marcellus-Updated-Economic-Impacts-5 .24.10.3.pdf. - EPA (U.S. Environmental Protection Agency). Diesel retrofit technology: An analysis of the cost-effectiveness of reducing particulate matter emissions from heavy-duty diesel engines through retrofits. Washington, DC: Office of Transportation and Air Quality; 2006.
- EPA. 2012. [May 30, 2013]. (History of the Clean Air Act). http://epa
.gov/oar/caa/caa_history .html#caa70. - Honeycutt M. Air quality impacts of natural gas operations in Texas. PowerPoint presentation at the Institute of Medicine workshop on the Health Impact Assessment of New Energy Sources: Shale Gas Extraction; Washington, DC: 2012.
- Kemball-Cook S, Bar-Ilan A, Grant J, Parker L, Jung J, Santamaria W, Mathews J, Yarwood G. Ozone impacts of natural gas development in the Haynesville Shale. Environmental Science & Technology. 2010;44(24):9357–9363. [PubMed: 21086985]
- Lash GG, Engelder T. Tracking the burial and tectonic history of Devonian shale of the Appalachian Basin by analysis of joint intersection style. Geological Society of America Bulletin. 2009;121:265–277.
- McKenzie LM, Witter RZ, Newman LS, Adgate JL. Human health risk assessment of air emissions from development of unconventional natural gas resources. Science of the Total Environment. 2012;424:79–87. [PubMed: 22444058]
- The Nature Conservancy. The Nature Conservancy’s roots of innovation 2010 annual report. Arlington, VA: The Nature Conservancy; 2010.
- Robinson AL. Air pollutant emissions from shale gas development and production. PowerPoint presentation at the Institute of Medicine workshop on the Health Impact Assessment of New Energy Sources: Shale Gas Extraction; Washington, DC: 2012.
- Roy AA, Adams PJ, Robinson AL. Journal of the Air & Waste Management Association. 2013. Air pollutant emissions from the development, production, and processing of Marcellus Shale natural gas. 10.1080/1096 2247.2013.826151. [PubMed: 24620400]
- TCEQ (Texas Commission on Environmental Quality). 2013. [May 30, 2013]. (Ozone design values for the DFW area: Comparison of ozone design values for the Dallas-Fort Worth area for the years 1991 through 2009). http://www
.tceq.state .tx.us/publications /pd/020/10-02/dfw-alt-text. - U.S. Census Bureau. Dallas, Texas: 2013. [May 30, 2013]. (State & county quickfacts). http://quickfacts
.census .gov/qfd/states/48/4819000.html. - U.S. Energy Information Administration. Annual energy outlook 2013 with projections to 2040. Washington, DC: U.S. Energy Information Administration; 2013.
- Witter R, McKenzie L, Towle M, Stinson K, Scott K, Newman L, Adgate J. Health Impact Assessment for Battlement Mesa, Garfield County Colorado. February 2011 Draft. Denver, CO: Colorado School of Public Health, University of Colorado Denver; 2011.
Footnotes
- 1
An AMCV is an air quality standard designed to protect health.
- 2
The probability that 1 in 100,000 individuals will contract cancer in a lifetime from continuous exposure to the chemical concentration.
- 3
See http://www
.tceq.texas .gov/airquality/barnettshale /bshale-viewer (accessed May 30, 2013). - 4
In Garfield County, 9 months to 1 year are required to develop a well, a well may undergo hydraulic fracturing multiple times, and there are usually multiple wells in a pad. Thus, the well development process may take up to 20 months or more.
- PubMedLinks to PubMed
- Air Quality - Health Impact Assessment of Shale Gas ExtractionAir Quality - Health Impact Assessment of Shale Gas Extraction
Your browsing activity is empty.
Activity recording is turned off.
See more...