By agreement with the publisher, this book is accessible by the search feature, but cannot be browsed.
NCBI Bookshelf. A service of the National Library of Medicine, National Institutes of Health.
Kufe DW, Pollock RE, Weichselbaum RR, et al., editors. Holland-Frei Cancer Medicine. 6th edition. Hamilton (ON): BC Decker; 2003.
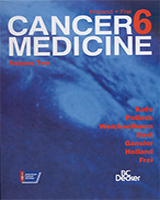
Holland-Frei Cancer Medicine. 6th edition.
Show detailsSoluble Factors Released by Tumor Cells
There is ample evidence that tumor cells produce immunosuppressive factors, and this has been verified to have local as well as systemic effects on immune function. In this section, we will discuss some of the better documented immunosuppressive factors released by tumor cells, their effects on immune function, and some strategies for therapeutic intervention based upon limiting the degree of immunosuppression associated with these molecules.
Cytokines
Transforming Growth Factor-β (TGF-β)
Tumors are actively immunosuppressive, and this fact is supported by extensive data revealing effects on immune cells found both in the tumor microenvironment and in the periphery. There are numerous mechanisms by which tumors mediate immunosuppression, including expression of Fas ligand (FasL) and secretion of various suppressive factors (reviewed in ref. 29). It is unequivocally established that many tumors produce transforming growth factor β-1 (TGF-β1) and -2 (TGF-β2), and extensive data support the conclusion that these factors are immunosuppressive for a variety of immune responses.29–35(Figure 15-1) In reality, the effects of TGF-β on immune responses are pleiotropic and can be manifested in numerous ways. For instance, there is evidence that TGF-βs inhibit T-cell and NK-cell responses to cytokines, including proliferative responses and production of cytokines.117,120–125 In contrast, there is also evidence that TGF-β serves as a chemoattractant for IL-2-activated NK cells, which would seem to foster potential antitumor effects of these cells.36,37 Similar conflicting effects of TGFs have also been observed in experimental systems.29 Therefore, it is best to consider the effects of TGF-β within the context of a given model system. For instance, it is generally held that IL-4 supports the development of Th2-type immune responses.38 However, in the presence of TGF-β, induction of an immune response driven by IL-4 resulted in Th1-type responses.39 These data clearly support the idea that it is best to consider the effects of TGF-β on an immune response to tumors within the context of that tumor's micro- and macroenvironments.

Figure 15-1
Tumor cells produce immunosuppressive factors such as TGFβ that inhibit normal anti-tumor function of immune effector cells.
Recent reports using a variety of tumor models have indicated that either the elimination of TGF-β or the inhibition of its function is of significance for tumorigenicity and/or immunogenicity of gliomas. Attempts at disruption of the production or function of TGF-β produced by gliomas have included irradiation and the use of protease inhibitors, which block the processing of the proform of TGF-β.33,40 In the case of irradiation, it was determined that TGF-β production on a per cell basis did not decrease and, in fact, was increased.40 This suggests that irradiation, while clearly applicable to therapy of gliomas, will not provide a means to eliminate the effects of TGF-β. It was determined, however, that using protease inhibitors was an efficient means of reducing the actions of TGF-β in vitro, but there has not yet been an analysis of this approach in vivo.33
An additional approach has involved gene transfection with decorin, a small, leucine-rich proteoglycan that binds and inactivates TGF-β.41–43 In these studies, it was determined that decorin transfection resulted in the abrogation of TFG-β activity in vitro and, more importantly, that cells expressing high levels of decorin were less tumorigenic in vivo. However, there is some controversy regarding whether the therapeutic effects of decorin are mediated by eliminating the effects of TGF-β.42 While further experimentation will be necessary to resolve this issue, the potential use of decorin in gene therapy protocols is apparent whether or not decorin:TGF-β interactions are involved in the loss of tumorigenicity.
There have also been more direct studies of the importance of TGF-β in tumorigenicity of gliomas. For instance, Jachimczak and colleagues treated human gliomas with TGF-β2-specific phosphorothioate-antisense (AS) oligodeoxynucleotides and determined that this resulted in a marked reduction in tumor-mediated suppression of lymphocyte proliferative responses to IL-2.44 In extending these types of observations, Fakhrai and colleagues transfected tumor cells with a constitutively expressed TGF-β AS construct and determined that reversal of TGF-β production resulted in a significant loss of tumorigenicity of 9L in vivo.45 This also resulted in an increase in the immunogenicity of 9L cells. Won and colleagues expressed a soluble form of the receptor type II receptor for TGF-β (TGFβ RII) in a mouse thymoma and determined that this tumor displayed reduced tumorigenicity and that there was a marked reversal of systemic immunosuppression in mice bearing this tumor compared to parental tumor.46 Witham and colleagues have recently determined that local production of TGF-β RII by glioma cells resulted in a significant reduction in tumorigenicity and, furthermore, that elimination of CD161+ cells (ie, NK and NK/T cells) reversed the protective effect of TGF-β RII.47 These data provide strong support for the in vivo significance of TGF-β RII and its capacity to promote immune responsiveness to tumors. These data also support the conclusion that reversal of production or activity of TGF-β produced by gliomas has significant influence on tumor establishment or progression in vivo. Data in these reports do not, however, resolve which immune mechanism(s) are operant in mediating the antitumor effects in vivo. Given the pleiotropic effects of TGF-β, it can be hypothesized that either nonadaptive or adaptive mechanisms, or both, are important. In that vein, it has been reported that TGF-β AS-transfected gliomas are more susceptible to lysis by lymphokine-activated killer (LAK) cells in vitro.48 As most LAK activity is derived from NK or NK-like cells, it is conceivable that these are the mechanisms operating in vivo in the models in which TGF-β AS is used. However, direct assessment of this is necessary and should represent a high priority in the field.
Interleukin-10 (IL-10)
CD4+ T helper cells, which are major regulatory cells in the immune system, are often defined functionally as being either Th1 or Th2 cells based upon differences in their production of cytokines, which govern the nature of a developing immune response. In terms of antitumor immunity, it is generally true that skewing toward greater production of Th1-type cytokines such as interferon-γ (IFNγ) results in greater cell-mediated immune responses and thus better antitumor responses. Skewing toward production of a Th2 response is indicated by elevated rates of production of cytokines such as IL-4, -5, or -10, and this often results in substantially greater humoral responses, which generally have less antitumor effect. IL-10 was originally defined as a factor produced by Th2 cells that inhibited the production of cytokines produced by Th1 cells, thereby influencing the development of immune responses toward a Th2 profile. There is evidence for elevated systemic levels of IL-10 in cancer patients, and this is associated with less effective antitumor responsiveness and a poorer prognosis for some tumors.49–55 Thus, dysregulation of antitumor responses could be mediated by skewing of cytokine production by T helper cells toward a Th2 phenotype (ie, IL-10). However, there is also substantial evidence that IL-10 is spontaneously secreted by a variety of tumor types, such as non-small-cell lung cancer, pancreatic cancer, leukemia, and squamous cell carcinomas of the head and neck.49–55 This represents a more direct evolution of immunosuppressive effect associated with tumors, although either source of IL-10 will obviously have a significant influence on the outcome of a developing antitumor response. In fact, there are multiple mechanisms of immunosuppression mediated by IL-10, and these include effects on T cell growth, differentiation, and function, as well as a direct effect on resistance of tumor cells to immune-mediated elimination. These mechanisms are illustrated in Table 15-1, where they are also compared with those mediated by TGF-β.
Table 15-1
Significance and Effects of Production of IL-10 and TGF-β by Tumor Cells.
Prostaglandins
Prostaglandins (PGs) have potent biological effects on the immune system, including suppression of T- and B-cell proliferation, inhibition of NK-cell-mediated cytotoxicity, and inhibition of tumor necrosis factor (TNF) production. PGs can also induce the production of IL-10, which has immunosuppressive effects, as indicated above.56 Over the past decade, a number of reports have provided data indicating that tumor cells can either directly produce PGs or induce macrophages in the tumor microenvironment to secrete PGs.57–64 For instance, several reports indicate that tumors, including colorectal carcinomas (CRCs) and head and neck cancers, produce prostaglandin E2 (PGE2), and this is responsible for some of the immunosuppressive effects associated with these tumors.57–64 The pathway responsible for these effects has recently been elucidated as being a consequence of cyclooxygenase (COX) overexpression in cancer cells, particularly COX-2.58, 65–67 Overexpression of COX-2 results in increased production of PGs by tumor cells and, in the case of PGE2, results in an upregulation of antiapoptotic proteins, such as Bcl-2, in tumor cells.66,67 This results in both the inhibition of immune function and a decrease in susceptibility of tumor cells to elimination by immune effector cells.
Elimination of Immune Effector Cells
Activation-Induced Cell Death
Activation-induced cell death (AICD) refers to induction of apoptosis of previously activated T cells upon subsequent encounter with antigen.68,69 This mechanism of elimination of T lymphocytes plays a significant role in downregulation of the immune response, in negative T-cell selection in the thymus, and in clonal deletion of activated T cells in order to maintain T-cell homeostasis. AICD was initially used to describe the apoptotic cascade induced in T lymphocytes in response to antigenic signaling.70 Resting mature T lymphocytes are activated when triggered via their antigen-specific T-cell receptor to elicit an appropriate immune response. In contrast, preactivated T cells may undergo AICD in response to the same signal. In the process of AICD, T-cell stimulation results in upregulated expression of surface death ligands, which in turn engage their counter-receptors on the same or a neighboring cell. AICD is mediated mainly following Fas (CD95):Fas ligand (FasL; CD95L) interactions (Figure 15-2), but the involvement of the TNF and TNF-related apoptosis-inducing ligand (TRAIL) systems have also been reported.71,72 T-cell susceptibility to AICD is regulated by the stage of T cell maturity, the previous activation history of T cells, and the presence of antigen-presenting cells.70,73,74 The majority of developing T cells in the thymus are eliminated by AICD following reaction with self-antigens. However, the role of Fas-FasL in thymocyte AICD is controversial, as negative intrathymic T cell selection proceeds normally in Fas-deficient lpr or FasL-deficient gld mice.75,76 In preactivated, mature T cells, AICD can be triggered via the TCR:CD3 complex or via other receptors known to activate T cells, such as CD2.77 Most of the studies regarding AICD were performed with anti-CD3- or anti-TCR-cross-linking antibodies, which induce AICD in mature, preactivated, but not resting T cells. Cross-linking of the TCR:CD3 complex induces AICD in T cells expressing either αβ or γδ TCR.78 AICD is dependent on those signal transduction molecules known to trigger effector function in T cells, including the TCR-ζ chain and protein tyrosine kinases, such as Lck, Fyn, and ZAP-70.79,80 The molecular regulation of AICD is still unclear; however, exposure to IL-2 has been reported to prime mature T lymphocytes for AICD.81,82 This increased susceptibility may be due to upregulation of Fas expression or to downregulation of endogenous inhibitors of the Fas cascade, such as FLICE inhibitory protein (FLIP).83 In contrast, various costimulatory signals, such as ligation of CD4 or CD28, protect T cells from AICD.84,85 Binding of CD28 on T cells by B7.1 or B7.2 expressed on presenting cells stimulates cytokine production, upregulates expression of endogenous inhibitors of apoptosis such as Bcl-XL and FLIP, and downregulates FasL.

Figure 15-2
Induction of apoptosis of lymphocytes or tumor cells following Fas:Fas ligand (FasL) interactions. A, Dysfunctional immune responses can result as some tumor cells express Fas ligand (FasL) and bind Fas expressed on some infiltrating lymphocytes resulting (more...)
AICD seems to be differentially regulated in T-helper subsets. Upon stimulation with phorbol ester and ionomycin or anti-TCR:CD3 Ab, Th1 clones express significantly higher levels of surface FasL than do Th2 clones.86 Although Th1 clones are considered to be more AICD sensitive than Th2 cells, the mechanisms regulating the relative resistance of Th2 clones are not clear. It has been suggested that a negative regulator of the Fas-cascade, such as the Fas inhibitor Fas-associated phosphatase-1 (Fap-1), may be induced in Th2 cells stimulated by anti-TCR:CD3.87,88
AICD is considered to be one of the mechanisms responsible for the increased apoptotic rate among TILs.89–91 Thus, antigenic stimulation within the tumor microenvironment might be involved in the enhanced expression and function of FasL on T cells, resulting in activation of autocrine or paracrine mechanisms of apoptosis. Such a scenario has been reported for human melanoma, where tumor cells caused apoptotic death of tumor-specific T cells only upon specific MHC Class I-restricted antigen recognition.90 This apoptotic death of antitumor T cells was blocked by anti-Fas mAb but mediated by FasL on activated T cells, as the melanoma tumor cells did not express either protein or mRNA for FasL.
Tumor “Counterattack”
In addition to AICD, a mechanism of tumor “counterattack” against Fas-expressing T lymphocytes has been proposed to explain the Fas-mediated apoptotic death of tumor-infiltrating lymphocytes. The Fas counterattack model proposes that FasL expressed on various types of tumor cells is utilized to mediate the death of Fas-expressing TILs.92,93 According to this model, FasL-expressing tumor cells counterattack the T lymphocytes utilizing the T cells' own principal mechanism of cytotoxicity. Various types of tumors have been reported to express FasL and induce apoptosis in T cells expressing Fas, including melanoma; colorectal carcinoma; colonic adenocarcinoma; head and neck squamous cell, ovarian, gastric, hepatocellular, and lung carcinomas; glioblastoma; esophageal tumors; and angiosarcomas.94–104 Most of the studies utilized in vitro experimental systems to demonstrate the tumor activity against cultured T lymphocytes. Other authors have reported that a significant increase in TIL apoptosis occurred in areas of FasL-expressing tumors or was consistently more frequent in FasL+ tumors.98,99,105 The level of lymphocyte infiltration has also been correlated with expression of FasL on tumor cells. Thus, angiosarcomas with low FasL expression were highly infiltrated, whereas sarcomas with high FasL expression were characterized by a reduced number of TILs.104 Fas expressed on rat histocytoma tumors, which were injected into the peritoneum of mice, killed a population of NK cells that would otherwise destroy the tumor.106 Despite numerous reports and accumulating evidence in support of the tumor counterattack model, however, the validity of the experimental data is highly controversial. It has now been recognized that most of the anti-FasL antibodies used for detection of FasL on tumor cells were not monospecific, leading to the report of false-positive results in multiple publications.107,108 Furthermore, in many of the studies assessing the inhibition of tumor counterattack by neutralizing anti-FasL antibodies or by Fas-Fc fusion proteins, there was no attempt to differentiate between tumor cells or T cells expressing FasL as the initiators of the attack. Such studies, therefore, could not distinguish between AICD and tumor counterattack as the mechanism responsible for the induction of the Fas cascade leading to T cell apoptosis.
Further controversy regarding the tumor counterattack model was also generated by in vivo studies indicating that rather than granting an immune privileged status to tumors, FasL on tumor cells mediated an inflammatory response, that is, tumor cells transfected with FasL underwent accelerated neutrophil-mediated rejection, and this tumor rejection was mediated through the membrane-bound form of FasL but not through soluble FasL.109,110 Interestingly, in the presence of TGF-β, the neutrophil-mediated rejection of the FasL-expressing tumor was inhibited, suggesting again that the cytokine milieu may affect the response to a FasL-expressing tumor.111
It is currently not clear why FasL triggers inflammation. It is possible that the expression of FasL on some tissues bears consequences that are different from those of its expression on lymphoid cells. The constitutive expression of FasL in the eye is antiinflammatory, whereas in the liver, its expression induces granulocytosis. This supports the hypothesis that it is possible that the response to FasL is tissue type-dependent. However, it must be stated that the granuolcytosis may also be explained as a response to a supraphysiologic expression of FasL, as naturally expressed FasL has not been reported to induce granulocytosis under physiologic conditions. The expression of Fas on neutrophils was required to induce the proinflammatory response.112,113 Thus, it is possible that under certain conditions, neutrophils respond to Fas triggering by production of inflammatory cytokines rather than apoptosis. The nonapoptotic response of neutrophils to FasL may be mediated by activation of caspases, which are known to be involved in generation of proinflammatory cytokines. For example, caspase-1 is responsible for processing of IL-1β into its mature form, whereas caspase-3 is involved in activation of the IL-16 and IL-18, two potent proinflammatory cytokines.114–116
The tumor counterattack model originated from initial studies demonstrating the involvement of FasL in mediating the immunologically privileged status of the retina of the eyes and the testes.117 However a role for FasL in protection of the testes or β-islet grafts from a counterimmunolgical response has been disputed in recent studies.118,119 This mechanism of immune evasion has been reevaluated, and some authors have retracted their earlier statements.120–122 Currently, the role of FasL as a mediator of immune privilege for certain tissues or types of tumors is controversial. However, there is no disputing that tumor induction of apoptosis in interacting lymphocytes is one of the immune evasion mechanisms used by cancer cells. As discussed below, apoptosis of immune cells at the tumor site may be induced by various tumor-derived cytokines. As demonstrated by several studies, AICD mediated by Fas-FasL interactions among T lymphocytes represents one of the lymphocyte apoptotic pathways occurring in the tumor microenvironment.90,123,124
Disruption of Signaling Pathways in Immune Cells
Impaired Expression of T-Cell Receptor ζ Chain
TILs and, to a lesser extent, peripheral T cells and NK cells from cancer patients or tumor-bearing animals have been reported to exhibit reduced expression of the TCRζ chain, a required structural component of the signaling complexes in these cells. The validity of this observation has been confirmed by numerous studies that reported a deficient expression of the ζ protein in T lymphocytes from patients with various types of tumors, including melanoma, renal cell carcinoma, ovarian carcinoma, cervical carcinoma, colon carcinoma, prostate cancer, and others.125–132 Decreased expression of this signaling protein has been correlated with immune dysfunction in cancer patients, including reduced proliferative responses following antigenic or mitogenic stimulation, reduced cytotoxic effector function, and reduced production of Th1 cytokines.125,128,133 In addition, a correlation has been demonstrated between tumor progression and aggressiveness and decreased levels of TCRζ expression.126,129,134 In patients with metastatic melanoma, decreased expression of the ζ-protein in peripheral blood T cells was associated with a poor prognosis and significantly shorter survival.135 In a retrospective immunohistochemical study performed on tumor biopsies obtained from 138 patients with oral carcinoma, it was demonstrated that low expression of the ζ-protein in cells at the tumor site correlated with poor survival.136
The mechanisms responsible for the decreased expression of ζ-protein in T lymphocytes of patients with cancer are not well understood. It was reported that splenic macrophages from tumor-bearing mice or normal spleen macrophages activated with zymosan A and lipopolysaccharide (LPS) were able to induce size-related changes in CD3-ζ, -γ, -δ, and -ε by contact-dependent interactions.137 Other studies have demonstrated that hydrogen peroxide secreted by tumor-derived macrophages or granulocytes downregulated the expression of the ζ chain.138–140 Recent studies demonstrated that Jurkat T cells cultured in media without L-Arg had a rapid decrease in expression of ζ-protein.141,142 The diminished expression of ζ-protein was paralleled by a decrease in ζ-mRNA, which had a significantly shorter half-life in the absence of L-Arg. However, in studies performed with cancer patients, no changes were detected in the ζ-mRNA level. Thus, it was assumed that the reduced expression of the ζ-protein was a posttranslational event. Various mechanisms may be involved in the downregulation of the ζ-protein, as a soluble factor derived from ovarian ascites was recently reported to have a reducing effect on ζ-mRNA.143
A posttranslational mechanism for ζ-protein downregulation was identified by studies demonstrating induction of an apoptotic cascade in T lymphocytes interacting with tumor cells obtained from ovarian cancer patients.97 Rabinowich and colleagues have identified the ζ-protein as a direct substrate for caspase-3 and -7.144 By site-directed mutagenesis, D84VLD87 was identified as one of the sites cleaved by both. Although it is currently unclear whether all T cells demonstrating reduced expression of ζ-protein are apoptotic or sensitized to apoptosis, these findings strongly suggest that the activation of intracellular caspases plays a role in ζ-protein degradation. Thus, caspase activation may represent a molecular mechanism for altered expression of the ζ-protein in patients with cancer or infectious diseases, in whom chronic activation or other apoptotic stimuli may induce the activities of these enzymes.
Subsequent studies by other investigators demonstrated a correlation between elevated caspase-3 activity and apoptosis of peripheral blood T cells and downregulation of the ζ-protein in patients with advanced cancer.132,145 In T lymphocytes induced to apoptose by ceramide, caspase-mediated ζ-protein degradation was found to correlate with downregulation in TCR expression.146 In renal cell carcinoma (RCC), approximately 15% of the TILs were apoptotic as assessed by terminal deoxynucleotidyl transferase-mediated nick end labeling (TUNEL) assays in situ.124 Interestingly, gangliosides, acidic glycosphingolipids, which usually reside at the outer leaflet of the plasma membrane, were identified in supernatants of RCC.147 Certain gangliosides are potent apoptotic inducers and may serve as inducers of apoptosis with subsequent caspase-mediated degradation of the ζ-protein.148 Gangliosides may also be shed into the systemic circulation and contribute to the apoptotic alterations observed in peripheral blood T cells.149
Recently, we demonstrated that the ζ-protein is also a direct substrate for granzyme B (GrB).150 Utilizing site-directed mutagenesis, we obtained evidence that the activity of GrB is directed toward different aspartic acid residues from that with recombinant caspase-3. GrB plays an important role in the apoptotic cascade induced in target cells as part of the cytotoxic mechanism mediated by granule exocytosis. Thus, in leukemic or virally infected T cells targeted by cytotoxic T cells, ζ-protein may serve as a specific substrate for GrB-mediated apoptosis. However, it is currently not known whether GrB has any role in the reduced expression of ζ-protein in T lymphocytes from cancer patients. The TCRζ chain has also been identified as an ubiquitinated protein targeted for proteosomal degradation.151–153 It is currently not known whether this mechanism of degradation has a role in the reduced expression of ζ-protein in cancer patients.
Impaired Activation of NFκB in T Cells from Cancer Patients
The nuclear factor κB (NFκB) signaling pathway is one of the best characterized of cellular signal transduction pathways intiating DNA transcription. In addition to regulating expression of genes involved in immune responses, such as IL-2, TNF, GM-CSF, M-CSF, and IFNγ, NKFB regulates the expression of various genes essential for cell survival, such as BcL-2 and Bcl-XL, and cellular inhibitors of apoptosis (cIAPs). It has been shown that the NFκB activation pathway was suppressed in peripheral blood T lymphocytes from some patients with RCC, as assessed by the electrophoretic mobility shift assay, which measures the DNA-binding ability of active NFκB (RelA) after stimulation with phorbol 12-myristate-13 acetate and ionomycin.154 Subsequent studies by the same group determined that the abnormal pattern of binding was due to impaired phosphorylation and degradation of its cytoplasmic inhibitor IκBα.155 The ganglioside GD3 and related structural analogs, such as ceramide C2, inactivated NFκB and stimulated a burst of reactive oxygen species (ROS) formation, which initiated a cell death cascade.156 Thus, gangliosides secreted by RCC may be directly involved in the inactivation of NFκB and the degradation of ζ-protein observed in this group of cancer patients.
Mechanisms of Resistance of Tumor Cells to Cytotoxicity
Immune effector cells mediate lysis of tumor target cells by one of several mechanisms, including (1) exocytosis of contents of cytoplasmic granules, including cytolysin and a family of serine proteases called granzymes, which can induce necrotic cell death and which support the induction of apoptosis in combination with additional factors and/or receptors; (2) induction of apoptosis via binding of cell surface receptors on immune cells (eg, FasL) to receptors on tumor cells (eg, Fas); and (3) release of soluble toxins (eg, TNF), which bind to their corresponding receptors on tumor cells and induce apoptosis. Additionally, tumor cells can be killed following cell surface binding by complement-fixing antibodies, which activate the complement pathway. In this mechanism, antibody binding to tumor cells activates an enzymatic cascade that is initiated with binding of CD3b to the antibody and results in the ultimate formation of a membrane attack component (MAC) composed of C5b-9. The MAC forms a pore of cells in the plasma membrane, which results in disruption of cellular integrity and subsequent lysis. As there is some continual low level of complement activation in the vascular system, mechanisms for tight regulation of the activation of complement have evolved to provide a degree of protection for normal cells. Cell surface molecules such as CD35, CD46, CD55, and CD59 are widely expressed and function as membrane complement regulatory proteins (mCRPs), which provide this protection. CD35 is expressed by erythrocytes, leukocytes, and glomerular podocytes.157 CD46 is expressed ubiquitously, except by erythrocytes.158 CD55 is ubiquitously expressed, except by NK cells.159 CD59 is expressed ubiquitously.160 CD35 and CD46 serve as cofactors for factor I-mediated cleavage of C3b; CD35 also has decay accelerating factor (DAF) function, as it accelerates the conversion of C3 or C5 convertases. CD55, originally described as DAF, accelerates the conversion of C3 convertases (C4b2a and C3bBb) and C5 convertases (C4b2aC3b and C3bBbC3b) by displacing C2a or Bb from the complexes. CD59 blocks cytotoxicity by binding C9 and thus inhibiting the insertion of C9 into the membrane to form a MAC.
Each of these processes involves a cascade of molecular events resulting in immune-mediated elimination of tumor cells. Given that fact, there are numerous points in the processes that, if disrupted, block the cytotoxic effect. Beyond that, a number of mechanisms have evolved that affect cytotoxicity as normal regulators of this process. This is of obvious importance to limit the opportunity for expression of cytotoxicity against normal cells. However, dysregulation of these mechanisms can also result in a loss of cytotoxicity against tumor cells.
Disruption of Induction of Apoptosis
Cytotoxic T cells and NK cells induce apoptosis in target cells by two major mechanisms: the engagement of death receptors on target cells and the action of granule proteins that have been exocytosed.161 Each of these mechanisms represents a series of pathways that converge on caspases, the principal effector proteases of apoptosis. Ligation of Fas receptors on target cells by FasL on cytotoxic cells initiates a death cascade, which involves recruitment of FADD and caspase-8. Although Fas is the dominant death receptor pathway utilized by cytotoxic lymphocytes, cytotoxic signaling via TNF receptor 1 (TNFR1) and TRAIL receptor (TRAILR) also converge on FADD and caspase-8 activity.162,163 The granule-exocytosis pathway is mediated by perforin and granzymes.161 While perforin by itself cannot induce apoptosis, it is required to release granzymes from their endolysosomal compartments, allowing them to access their substrates in the cytoplasm.164
GrB, the prototypic member of the granzyme family of serine proteases, shares substrate specificity with caspases for cleavage after aspartate residues. GrB has been reported to cleave caspases, including -3, -6, -7, -8, and -10, in vitro. It has been assumed that it can initiate apoptosis at multiple points along the caspase-dependent apoptotic cascade.161,164,165 GrB can also initiate a death cascade through a caspase-independent mechanism that is partly inhibitable by Bcl-2.166 Consistent with these observations, recent reports have suggested that the preferred mechanism for rapid induction of apoptosis by GrB is mediated by the cleavage of Bid, which initiates a mitochondrial apoptotic cascade.167–169 Bid, a BH3-only proapoptotic Bcl-2 family member, is cleaved by caspase-8, lysosomal proteases, or GrB. Although cleaved at different sites, each of the resultant truncated Bids (tBid) translocates to the mitochondrial outer membrane, where it triggers the release of mitochondrial intermembrane cytochrome c. Upon its release, cytochrome c, in concert with apoptosis-activating factor-1 (APAF-1) and dATP, activates caspase-9, leading to the subsequent activation of caspase-3. Mitochondrial-mediated apoptosis may also proceed in a caspase-independent manner; concomitant with the release of cytochrome c, other intermembrane proapoptotic proteins are released, including AIF, HtrA2, and SMAC. Apoptosis-inducing factor (AIF) translocates to the nucleus, where it induces caspase-independent chromatin condensation and DNA fragmentation.170 HtrA2 and SMAC are inhibitors of the IAP family and thus function by relieving caspases inhibition by cIAPs. However, SMAC also has a proapoptotic function that is IAP-independent.171 In addition, GrB can also cause DNA degradation in cells that lack functional caspases. This DNA degradation is mediated by the endonuclease ICAD (DNA fragmentation factor), which is directly activated by GrB in vitro and in target cells.172
Resistance to apoptosis can be acquired by cancer cells through alterations in the components of the apoptotic machinery. Such alterations may serve for the selection of CTL-resistant tumor cell variants that could survive under immune pressure.
Resistance to Fas-mediated cytotoxicity is acquired by a variety of tumors through downregulation of Fas expression. Loss in Fas expression has been detected in melanoma, pulmonary adenocarcinoma, and esophageal cancer.94,173,174 It has also been demonstrated that Fas resistance was associated with an increased number of metastases.175 Although in most cases the mechanisms responsible for the loss in cell surface Fas expression have not been explored, somatic mutations of the Fas gene were detected in certain human malignancies.176
Fas- and TRAILR-mediated pathways are efficiently inhibited by FLIP, a caspase-8 inhibitory protein that blocks DED-DED interactions between FADD and caspase-8 or -10.177 Recent studies demonstrated that overexpression of FLIP protects tumor cells from in vivo immune response in mouse models.178,179 These findings suggest that FLIP overexpression may be considered as a mechanism of immune evasion. However, upregulated expression of FLIP has been identified in only a few types of tumors.180,181 Another inhibitor of Fas signaling, Fap-1, has been demonstrated to negatively regulate the Fas cascade and also to exhibit an increased expression in hepatoblastomas and in HTLV-1-infected cells.182,183
Actin cytoskeleton has been shown to be required for Fas-mediated apoptosis.184 Actin cytoskeleton integrity and the ezrin-mediated linkage between actin and Fas are essential for Fas-mediated apoptosis in CD4 T cells.185 Although downregulated expression of ezrin and disruption of cytoskeletal actin may function to block Fas-mediated cytotoxicity, such naturally occurring alterations have not yet been identified in tumor cells.
The requirement for caspases in GrB-mediated apoptosis has been evaluated in numerous studies.161 It has been concluded that GrB can induce DNA fragmentation and cell death in a caspase-independent manner. However, in the presence of caspase inhibitors, the ensuing apoptotic process is slow and inefficient. This conclusion suggests that endogenous expression of caspase inhibitors, such as XIAP, c-IAP-1 and c-IAP-2, will not completely block GrB-mediated cytotoxicity but serve to slow the death process significantly. An efficient mechanism for inhibition of GrB has recently been elucidated with the identification of proteinase inhibitor-9 (PI-9), a serpin inhibitor of GrB.186,187 This human serpin is found in the cytoplasm and nucleus of CTL (cytolytic T cells), and can form a tight complex with GrB via serpin-proteinase interaction. It has been proposed, but not yet directly investigated, that PI-9 serves to protect CTL from misdirected autologous GrB. Recent studies have shown that PI-9, and also its murine homologue SPI-6 (68% identity), are expressed in a variety of murine and human tumors.188,189 The significance of the PI-9 family as a tumor mediator of immune escape remains to be determined. An additional specific inhibitor of GrB, adenovirus L4-100K protein, has recently been identified as a GrB substrate with a direct inhibitory effect on GrB.190
The Bid-mediated mitochondrial apoptotic cascade has been determined to be a significant component of the GrB mechanism of cytotoxicity.168 Thus, positive and negative regulators of the mitochondrial apoptotic cascade are also involved in controlling the apoptotic response to GrB. Significant protection from GrB-mediated cell death has been observed in cells with upregulated expression of antiapoptotic Bcl-2 family members, such as Bcl-2 and Bcl-XL,166,169,171 or in cells deficient in expression of Bax and Bak, proapoptotic Bcl-2 family members.167,191 Such mitochondrial mechanisms for in vivo regulation of GrB appear to be widely utilized by tumor cells. Overexpression of Bcl-2 without associated chromosomal translocations has been reported in a wide variety of cancers. Mutations of the Bak gene were identified in gastric and colorectal cancers, whereas Bax deficiency was reported in colon carcinoma and hemapoietic malignancies. 192–194 A variety of experiments have provided conclusive evidence that elevations in Bcl-2 expression or decreases in Bak and Bax cause resistance to chemotherapeutic drugs, while decreases in Bcl-2 expression promote apoptotic responses to anticancer drugs.195,196 Although altered expression of various Bcl-2 proteins may significantly affect the mitochondrial component of GrB cytolytic activity, it is unlikely to completely block the apoptotic effects of GrB, demonstrated to have multiple entry points within the cell apoptotic machinery.
Expression of Membrane Complement Regulatory Proteins by Tumor Cells
As there is evidence for production of tumor-specific antibodies in many patients and a continual availability of complement components in these patients, there have been many studies directed at understanding why there is not an efficient elimination of tumor cells via complement-mediated cytotoxicity. This has frequently been directed toward the study of the expression of mCRPs on tumor cells and whether this affects complement-mediated lysis. The majority of solid tumor cells have, in fact, been reported to express CD46, CD55, and CD59; however, there is little data available regarding expression of CD35 on tumor cells. This includes data regarding expression on tumors from the respiratory tract, alimentary tract, kidneys, skin, central nervous system (CNS), eye, breast, and male and female genital tracts. There are contrasting reports indicating a lack of expression of mCRPs on some lung tumors, such as non-small-cell lung carcinomas; a subset of breast carcinomas; and some renal tumors.
There is evidence of a greater level of expression of CD46, CD55, and CD59 on tumor cells in comparison to normal tissue; however, some data also suggest comparable levels of expression. The lack of a clear indication of elevated levels of mCRPs on tumor cells versus normal cells has been attributed to the fact that CD55 and CD59 are GPI-anchored proteins and are easily subjected to cleavage by phospholipases. CD46 requires proteolytic cleavage for release from the plasma membrane. However, there is evidence for soluble CD46, CD55, and CD59 in sera and even evidence for elevated levels of CD46 in the sera of cancer patients.197–199 Interestingly, the soluble forms of CD46 and CD55 have been shown to inhibit complement activation. These data suggest that both cell surface mCRPs and soluble CRPs might represent mechanisms utilized by tumors to escape elimination by the immune system (Figure 15-3).

Figure 15-3
A, Immune effector cells release contents of cytolytic granules that form transmembrane pores in the plasma membrane of tumor cells allowing internalization of molecules that induce apoptosis. B, Some tumor cells express high level of molecules that block (more...)
As an indicator of the influence of expression of CD46, CD55, and CD59 on lysis of tumor cells, a number of in vitro studies have been carried out that block binding of CD46 or enzymatically cleave CD55 and CD59 from tumor cells followed by a determination of their susceptibility to complement-mediated lysis. As would be predicted, all three mCRPs were found to provide a means of inhibition of lysis of tumor cells, with CD55 and CD59 having the prevalent role.200–207 In determining an in vivo role for mCRPs as a mechanism utilized by tumors for protection from immune elimination, a correlation has been sought between increased levels of expression of mCRPs in tumor and reduced signs of complement activation. While the available data are limited, it has been reported that there was a strong correlation between high levels of expression of CD55 and low levels of C3 deposition in thyroid carcinoma.205 In another study, C3 deposition was found associated with blood vessels but not in tumor nests.207
At this point, there is a growing body of evidence supporting the use of mCRPs by tumor cells as a means of escaping elimination by complement-mediated lysis. It will be necessary to generate additional data to assess the extent of this phenomenon and to determine whether expression of mCRPs also disrupts the function of molecules with homology to C9, such as cytolysin, which is exocytosed from cytoplasmic granules by NK and T cells in the process of cell-mediated cytotoxicity. It will also be of interest to determine whether reversing the effects of tumor-associated mCRPs will provide an improvement in the elimination of tumors using antibody-based immunotherapies that rely upon complement activation or antibody-dependent cellular cytotoxicity (ADCC).
Evasion of Immune Reactivity by Tumor Cells
In addition to directly eliminating immune cells and suppressing their function by release of inhibitory factors that directly or indirectly suppress immune function, tumors also evolve mechanisms for evasion of recognition by immune cells. The recognition of tumor cells by effector T cells is based upon a complex pathway of processing and presentation of endogenous tumor antigens in the context of MHC Class I molecules. In essence, cellular proteins are degraded in the multicatalytic proteosome, particularly by the LMP-2 and -7 subunits, in the cytoplasm of tumor cells. Peptides are then transported into the endoplasmic reticulum via proteins known as transporters of antigenic peptide-1 (TAP-1) and TAP-2. Peptides are then inserted into a binding groove of MHC Class I heavy chains that have associated with β2 microglobulin, and the entire complex is transported to the cell surface. Once expressed on the cell surface, the complex is available for recognition by T cells. Given the complexity of this process, it can be predicted that tumor cells evolve that have a disruption in some component of this pathway and thus escape detection by the immune system. This has, in fact, been shown to be the case. Loss of MHC Class I expression can be based upon mutations in any of the proteins involved in processing antigenic peptides or in Class I molecules themselves. Overtly, it has been reported that as many as 40% of tumors have alterations in expression of Class I molecules (reviewed in ref. 208). Given the relatively high number of changes in Class I expression in tumors, it does not seem likely that alterations in the coding regions of structural genes for Class I heavy chains are primarily responsible for this loss of expression, as this would require multiple mutations to affect expression. Therefore, alterations in transcription of Class I proteins or other proteins involved in antigen processing, or alterations in proteins involved in antigen processing, are more likely candidates for affecting this mechanism of immune evasion.
There seems to be a correlation between both the degree of tumor differentiation and expression of MHC Class I, that is, less well-differentiated tumor cells have reduced expression of Class I. This has been reported for a variety of tumor types, including breast, lung, basal cell carcinomas, and larynx.208 Furthermore, there is an association between metastasis, tumor progression, and poorer prognosis for tumors having reduced expression of MHC Class I.209,210 These data provide compelling evidence for loss of Class I determinants representing a major factor in tumor progression.
Disruption of Antigen Processing and Presentation by Tumor Cells
There is substantial evidence for reduced expression of proteins involved in antigen processing and presentation, and its importance for antitumor immunity as it results in a lack of recognition of tumor cells. There is evidence for heterogeneous downregulation of expression of LMP-2, LMP-7, TAP-1, and TAP-2 in a variety of tumor types, including small-cell lung carcinomas, hepatocellular carcinomas, melanomas, and renal cell carcinomas. This results in a loss of transport of antigenic peptides into the endoplasmic reticulum and therefore a loss of expression of the peptide/MHC complex on the tumor cell surface. Well-characterized models of this defect are represented by the murine tumor RMA-S and the human large-cell lymphoma (LCL),211–228 which have been shown to lack expression of MHC Class I determinants, even though Class I genes are transcribed normally in both cell lines. It was determined that there were deletions in the MHC Class II region corresponding to the region encoding the TAP transport proteins.225 Furthermore, it has been reported that restoring expression of TAP in these cell lines by transfection with cDNAs encoding TAP restored expression of MHC Class I determinants.225 These data all strongly support the conclusion that selection for tumor cell mutants that have disruptions in processing of antigenic peptides can be an important means of evasion of the immune response.
Reduced Expression of MHC Class I Determinants
Deletions in genes encoding proteins involved in processing of antigenic peptides can be important for evasion of immune responses. However, it is also clear that tumor cells lose expression of MHC Class I protein that is not based upon alterations in expression of proteins involved in antigen processing. As this results in a loss of recognition of tumor cells by MHC Class I-restricted T cells, which are putatively the most important for elimination of tumor cells, the consequences on immune mediated elimination of these cells is obvious. Loss of expression of Class I determinants may manifest as a selective loss of human leukocyte antigens HLA-A, -B, or -C, or may be represented by an imbalance in expression of HLA-A as opposed to HLA-B, and vice versa.208
- Mechanisms of Immunosuppression - Holland-Frei Cancer MedicineMechanisms of Immunosuppression - Holland-Frei Cancer Medicine
Your browsing activity is empty.
Activity recording is turned off.
See more...