NCBI Bookshelf. A service of the National Library of Medicine, National Institutes of Health.
National Research Council (US) and Institute of Medicine (US) Committee on State of the Science of Nuclear Medicine. Advancing Nuclear Medicine Through Innovation. Washington (DC): National Academies Press (US); 2007.

Advancing Nuclear Medicine Through Innovation.
Show detailsIn this chapter, we describe the role of nuclear medicine imaging in patient care and review how these imaging approaches contribute to the diagnosis of disease, to the assessment of the disease-related risk to patients, and to individualizing treatment strategies for improving patient outcomes and survival. The chapter is organized into the following sections:
- Background (3.1),
3.1. BACKGROUND
Nuclear medicine imaging non-invasively provides functional information at the molecular and cellular level that contributes to the determination of health status by measuring the uptake and turnover of target-specific radiotracers in tissue. These functional processes include tissue blood flow and metabolism, protein—protein interactions, expression of cell receptors in normal and abnormal cells, cell—cell interactions, neurotransmitter activity, cell trafficking and homing, tissue invasion, and programmed cell death. By providing information on these processes, nuclear medicine imaging offers a broad array of tools for probing normal and disease-related states of tissue function and response to treatment.
The addition of anatomic imaging provided by computed tomography (CT) to functional imaging of positron emission tomography (PET) and single photon emission computed tomography (SPECT) has further expanded the utility and accuracy of nuclear medicine imaging. By using combined-modality PET/CT and SPECT/CT devices, functional processes can be localized within the body to an anatomically identified or, in some instances, as yet unidentifiable structural alteration. These devices have enhanced the accuracy with which disease can be detected, aided in the determination of the extent and severity of disease, enhanced the accuracy for identifying disease-related risk, and improved the ability to monitor patient response to therapy.
3.2. CURRENT STATE OF NUCLEAR MEDICINE IMAGING AND EMERGING PRIORITIES
This section describes the use of nuclear medicine imaging for three types of diseases to illustrate its impact on patient diagnosis and management and to identify emerging priorities. The three types of disease are cancer (Section 3.2.1), cardiovascular disease (Section 3.2.2), and neurological disorders (e.g., Alzheimer’s disease) (Section 3.2.3). In addition, the use of nuclear medicine imaging in drug development is discussed in Section 3.2.4.
3.2.1. Cancer
Cancer develops when cells begin to divide out of control. One hallmark of cancer cells is that they consume larger amounts of glucose than normal cells, because of a shift in energy production. This shift is known as “the Warburg effect” (Sidebar 3.1). Fluorine-18-fluorodeoxyglucose (FDG)- PET (Sidebar 2.2) has exploited this feature of cancer cells to detect differences between cancer and normal cells in the consumption of glucose. The accumulation of FDG in cancer cells represents an in vivo correlate of the abnormal mitochondrial function found in many types of cancer cells. Differences in rate of glucose utilization distinguish malignant from benign tumors and identify the presence and spread of tumor metastases as measures of disease severity. This information is important for tumor staging and for designing therapeutic strategies.
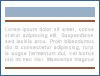
SIDEBAR 3.1
The Warburg Effect. Cells generate energy in two main ways: oxidative phosphorylation in mitochondria and glycolysis in the cytoplasm. In oxidative phosphorylation, 38 adenosine triphosphate (ATP) molecules are generated per glucose molecule. In contrast, (more...)
There is increasing evidence that imaging with FDG-PET may have an even greater impact on patient management as a way of monitoring tumor response to therapy (Juweid and Cheson 2006, Weber and Wieder 2006). Changes in glucose consumption can be detected using FDG-PET, where a reduction in tumor uptake of FDG predicts the likely effectiveness of chemotherapy. As Figure 3.1 illustrates, a favorable response can be detected by PET, but not with CT, as early as 2 weeks from initiation of chemotherapy in a patient with esophageal cancer (Wieder et al. 2005). At 2 weeks, the tumor volume as measured with CT had decreased minimally (diameter from 21 mm to 19 mm), while the FDG uptake had declined by about 50 percent. At 3 months, the tumor volume has strikingly decreased and the FDG uptake is only faintly visible. By contrast, in patients where there is a persistent high uptake of FDG, the absence of a therapeutic response is noted. Therefore, early assessment of tumor response to therapy with FDG-PET has the potential to considerably reduce the side effects and costs of ineffective therapies.

FIGURE 3.1
Monitoring the effects of chemotherapy on tumor volume and glucose uptake with serial multislice computed tomography (MSCT) and PET imaging in a patient with cancer of the esophagus. Imaging was performed before the start of treatment (left), 2 weeks (more...)
As noted above, tumor imaging with FDG-PET has been clinically useful in oncology because it captures the increased rate of glucose utilization. Yet, its utility has some limitations due to organ-specific utilization of glucose. For example, its use in diagnosing prostate and liver cancers has been limited due to the low metabolic activities of these cancers. The brain, in contrast, uses glucose for normal function. This characteristic has made it difficult to delineate tumors from normal brain tissue by FDG-PET. Similarly, increased glucose utilization is also observed when the body responds to damage (i.e., inflammation). Therefore, certain inflammatory processes cannot be differentiated from tumor tissue by FDG-PET.
However, depending on the radiotracer used, PET provides diagnostic information based on other types of metabolic activity, such as amino acid1 metabolism, cell proliferation, and tissue hypoxia.2 For example, amino acids and amino acid analogs3 have been labeled with fluorine-18 or carbon-11 and have been reported to be superior to FDG for imaging of brain tumors (Pirotte et al. 2004, Nariai et al. 2005, W. Chen et al. 2006). Another class of PET tracers that has shown promise is radiolabeled thymidine analogs (Shields 2006). The use of these tracers is based on the hypothesis that they mimic the biological behavior of thymidine, and thereby provide a measurement of DNA synthesis and cell proliferation in vivo. By extension, these tracers provide an accurate measure of tumor growth (Shields et al. 1998). Analogues of thymidine such as fluorine-18-fluoro-L-thymidine and fluorine-18-1-(2'-deoxy-2'-fluoro-β-darabinofuranosyl) thymine, also are being investigated as potential agents for monitoring early response to therapy.
Another promising application of PET is the use of probes, such as fluorine-18-fluoromisonidazole, that detect tumor hypoxia, which can affect tumor response to radiation therapy (Rajendran et al. 2006). The phe nomenon that cells are more sensitive to radiation in the presence of oxygen is well-established (Mottram 1936), and resistance to radiation has been observed in some tumors with considerable hypoxic fractions. Radiolabeled peptides, antibody fragments, and, more recently, nanoparticles targeting different cell surface molecules also hold promise for tumor imaging and for targeted radionuclide therapy. Several of these radiolabeled peptides and antibody fragments targeting a variety of cell surface molecules have entered clinical trials (Sharkey and Goldenberg 2005).
These emerging radiotracer approaches hold promise for further individualization of cancer treatment. They will allow for the imaging of biological processes that are characteristic of cancer cells. For example, a variety of new anti-cancer drugs such as inhibitors of epithelial growth factor receptors have been found highly effective for killing cancer cells (Sequist et al. 2007). If additional tumor characteristics can be identified with target-specific molecular probes, it will become possible to select specific treatment strategies for individual patients and improve the probability of treatment success.
In addition, hybrid imaging devices, such as PET/CT, which combines the functional information provided by PET with the anatomic information provided by CT, have transformed staging and restaging of patients with cancer. As noted earlier, PET has the ability to detect differences in metabolic activity. However, without the map of the body that is provided by conventional imaging methods, it is difficult to pinpoint the organ or organ region in which abnormal activity is occurring. The diagnostic accuracy of PET/CT imaging with FDG exceeds that of PET or CT alone (Lardinois et al. 2003). Figure 3.2 depicts images taken with PET/CT in a patient with lung cancer. PET/magnetic resonance imaging (MRI), another hybrid imaging modality that merges anatomical and functional information, is currently under development (Cherry 2006). Although its role in patient care needs to be determined, it holds particular promise for studies of the brain.

FIGURE 3.2
Staging of lung cancer with FDG and PET/CT. The whole-body image (Panel A) shows normal FDG uptake in the brain and the urinary bladder. In addition, several regions of intensely increased FDG uptake are seen in the chest. On the cross-sectional images (more...)
3.2.2. Cardiovascular Disease
In cardiology, nuclear medicine imaging has assumed an important role in the diagnosis as well as the management of patients with coronary artery disease.4 Myocardial perfusion imaging (Sidebar 3.2) is the most widely used approach in patients with suspected cardiac disease. Perfusion imaging of the heart is highly accurate for detecting the presence of coronary artery disease. In addition, the test can predict a patient’s risk for further cardiac disease (e.g., non-fatal heart attack) and cardiac death. This allows physicians to provide better care to patients with advanced and disabling cardiac disease by guiding therapeutic decisions; the therapies can range from conservative, drug-based management of disease to more aggressive forms of intervention, such as surgery to restore blood flow. Because of the high prevalence of coronary artery disease, myocardial perfusion imaging studies have become the most widely used nuclear medicine imaging test. More than 7 million myocardial perfusion imaging studies are performed each year in the United States alone (Heinz Schelbert, UCLA, personal communication, March 8, 2007).
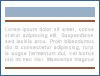
SIDEBAR 3.2
Myocardial Perfusion Imaging. Myocardial perfusion imaging is a test that allows doctors to examine blood flow to the heart muscle (i.e., myocardium). The patient is first injected with material labeled with a radionuclide, such as technetium-99m, thallium-201, (more...)
Approaches that primarily employ PET have been useful in delineating patterns of metabolism of both the healthy and the diseased heart. Metabolic activity demonstrated with PET and FDG in myocardial regions with diminished blood flow predicts an improvement in contractile function if blood flow is restored by coronary artery revascularization (Tillisch et al. 1986). Furthermore, radiotracers of fuel substrates of the heart, such as carbon-11-labeled fatty acid, glucose, and acetate, that can be imaged with PET offer a means for identifying changes in the heart’s substrate metabolism that are associated with age, obesity, or diabetes (Davila-Roman et al. 2002, Kates et al. 2003). Better understanding of these metabolic changes provides a framework for developing therapeutic strategies that may delay or avert progressive deterioration of heart muscle function and possible heart failure. Existing imaging techniques also offer a means for assessing the effectiveness of gene- and cell-based approaches for repairing the injured heart muscle tissue or for improving cardiac function. Changes in blood flow in response to angiogenic gene therapy with vascular endothelial growth factor, for example, can be monitored non-invasively (Udelson and Spiegler 2001). Similarly, effects of stem cell transplants on blood flow and metabolism on ischemically injured myocardium (i.e., after an acute myocardial infarction) can be demonstrated (Dobert et al. 2004). Nuclear medicine imaging will likely play an important role in the development and design of new therapies for cardiac disease.
New radiotracer techniques that are currently being investigated hold promise for the early diagnosis of coronary atherosclerosis.5 Inflammation of lipid-rich deposits in the wall of the major arteries, called atherosclerotic plaques, can rupture and cause non-fatal heart attacks or cardiac death. Currently, blood biomarkers, such as C-reactive protein, that measure acute inflammation, have been associated with future coronary events; however, they do not indicate where the atherosclerotic plaque is located. The ability to pinpoint the locations of plaques within the coronary arteries may help predict which individuals are predisposed to serious cardiac events. Cardiovascular imaging with tomographic modalities such as CT is expected to help identify patients with vulnerable plaques earlier than with conventional coronary angiography (Schoenhagen et al. 2004). Nuclear medicine imaging studies have also indicated that localization may indeed be possible (Dunphy et al. 2005). For example, in patients at risk for stroke, FDG uptake was found to be considerably increased in diseased carotid arteries, reflecting severe inflammation of atherosclerotic lesions with a high potential of plaque rupture (Tawakol et al. 2006).
In patients with cardiovascular disease, hybrid imaging techniques such as PET/CT, SPECT/CT, and PET/MRI will likely facilitate the assessment of functional consequences of disease-related structural alterations. Conversely, they will also allow molecular and cellular processes to be assessed in absolute units and assigned accurately to structural alterations. These advantages offer opportunities for improving disease detection, characterization, and treatment, as well as treatment monitoring in patients with cardiovascular disease. Their benefits could include more comprehensive assessments of cardiovascular health and disease and improved targeting of coronary vascular interventions, as well as accurate measurement of the severity of atherosclerotic disease, atherosclerotic plaques, and the effectiveness of plaque stabilizing therapies (Tarahan et al. 2006).
3.2.3. Neurological Disorders
A third clinical specialty where nuclear medicine imaging has played an important role in patient care is neurology. Radiotracer approaches aid in brain tumor evaluation and early identification of recurrence, in the planning of surgical treatment of seizure disorders, and, importantly, in assessing neurodegenerative disorders. As in other tumors, FDG is used in the diagnosis and characterization of brain tumors.
However, as noted earlier, the diagnostic accuracy with FDG has remained limited due to the high rate of glucose metabolism, and the high radiotracer uptake in normal brain tissue. This limitation has prompted the development and application of radiotracers such as carbon-11 methyl-methionine, fluorine-18-fluoro-l-phenylalanine, or fluorine-18-fluoro-L-thymidine, which serve as markers of amino acid transport and metabolism and DNA synthesis. These radiotracers target tumor tissue in the brain and contribute to the grading of tumor aggressiveness and, more importantly, to distinguishing tumor recurrence from post-surgical tissue reactions and scar tissue formation (Chen et al. 2005, P. Chen et al. 2006) (Figure 3.3).

FIGURE 3.3
MRI and PET brain images in a patient with a brain tumor (grade II oligodendroglioma). The tumor in the left brain hemisphere as seen on the MRI image (left panel) is associated with diminished FDG uptake and thus reduced glucose utilization (center) (more...)
In seizure disorders, PET imaging with FDG has been found useful for localizing potentially epileptogenic regions of the brain, and their spatial distribution and extent. Accurate identification of such aberrant brain tissue is critical for determining eligibility of patients for surgical treatment approaches designed to abolish seizure disorders that are inadequately controlled by medications. Neurodegenerative disorders, such as Alzheimer’s (Figure 3.4), Pick’s, and Huntington’s diseases (Sidebar 3.3), are typically associated with decreased glucose metabolism in certain parts of the brain. Each of these disorders is associated with diminished metabolism in specific brain regions that are distinguishable by FDG-PET (Silverman et al. 2001). Serial brain imaging studies with FDG-PET also allows monitoring of the rate of disease progression (Alexander et al. 2002).

FIGURE 3.4
FDG-PET brain images in a normal volunteer (left panel) and in a patient with Alzheimer’s disease (right panel). Tomographic slices through the brain at the level of inferior parietal/superior temporal cortex are shown. The color displayed in (more...)
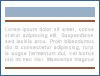
SIDEBAR 3.3
Neurodegenerative Disorders. Alzheimer’s disease is the most common form of dementia among elderly people, affecting an estimated 4.5 million Americans. It is a brain disorder that seriously affects a person’s ability to carry out daily (more...)
Clinically it is difficult to differentiate mild cognitive impairment that is the result of a neurodegenerative disorder from that which derives from non-neurodegenerative causes or normal aging. This has prompted research and development of novel radioligands for targeting β-amyloid in senile plaques and tau in neurofibrillary tangles as noninvasive neuropathologic markers (Figure 3.5). Clinical studies support the promise of these novel radiotracers (e.g., the carbon-11-labeled Pittsburgh compound B (PIB) or the fluorine-18-labeled-2-dialkylamino-6-acylmalononitrile-substituted naphthalenes) for separating early stages of neurodegeneration from other age-related causes of cognitive impairment (Klunk et al. 2004). These radiotracers also appear to be useful for monitoring disease progression and outcomes of drug treatment (Engler et al. 2006).

FIGURE 3.5
PIB PET brain images in a patient with Alzheimer’s disease (AD, left) and in a normal control person. The PET PIB images are compared to anatomic maps of the brain generated with MRI which do not indicate any abnormalities. Note the intense radiotracer (more...)
Another important future goal will be to develop improved diagnostics and protective therapies for neurological disorders, such as Alzheimer’s and Parkinson’s diseases. To reach this goal, a more detailed understanding of the molecular changes that occur in the brain during the early stages of dis ease development will be required. Furthermore, increasing the availability of radiotracers that have been developed to pinpoint specific molecular changes and monitor response to therapy will enable us to reach this goal. For example, many radiotracers, such as fluorine-18-fluoroDOPA, for assessing the integrity of the brain dopamine system have been developed but are not readily available. Making these and other radiotracers more widely available could result in more accurate diagnosis and differential diagnoses of Parkinson’s disease (Piccini and Whone 2004). In addition, other neurotransmitter systems (e.g., cholinergic, noradrenergic, serotonergic) also degenerate in Parkinson’s disease, and the use of imaging to monitor changes in these systems, particularly in relation to disease progression, could represent another important future research direction (Brooks 2007).
Similarly, treating psychiatric disorders, such as depression, schizophrenia, and addiction, is a special challenge. Many of the existing treatments are inadequate, with major side effects or high non-response rates. In the future, the widespread availability of highly specific radiotracers that can be used in basic neuroscience research in humans can be expected to aid in understanding the biological processes of these diseases and, ultimately, the development of better treatments (Koob 2006, Zipursky et al. 2007).
3.2.4. Drug Development
In addition to its role in patient care, nuclear medicine imaging has the potential to accelerate the drug development process and substantially reduce the time and expense of bringing a drug to market. As described in Chapter 2, use of nuclear medicine imaging during the drug development process could identify which drugs should advance from animal to human studies, validate the mechanism of drug localization, evaluate drug distribution to target tissue, establish the drug occupancy of receptor sites, assess the actions of new agents on specific molecular targets or pathways, and determine appropriate dose range and regimen (Eckelman 2003).
Already, the pharmaceutical industry increasingly relies on small animal imaging laboratories for drug development and evaluation. Small animal imaging laboratories equipped with nuclear medicine imaging devices such as microPET or microSPECT but also with microCT, microMRI, and optical (e.g., bioluminescence and fluorescence) imaging systems offer an ideal environment for rapid and cost-efficient screening and development of new molecular probes and drugs. The new image-based assays account therefore for most of the newly developed radiopharmaceuticals in both academia and industry. Using small-animal imaging instruments and radiolabeled versions of drug candidates in which a carbon atom or other atoms of the drug molecule are substituted with a radionuclide of the same element, the binding of drug candidates to target and non-target tissues can be determined with relative ease and tissue pharmacokinetics can be studied. Furthermore, with targeted radiotracers, the efficiency of target occupancy and inhibition can be assessed non-invasively. Based on these animal data, imaging biomarkers can be developed to monitor treatment effects and to determine optimal drug doses on a molecular level in clinical studies. The small imaging devices also offer a means for rapid screening of potential drug candidates. For example, the effects of novel compounds on cell proliferation or cell metabolism can be determined in small-animal tumor models with fluorine-18-FLT and fluorine-18-FDG and thus serve as a “generic pharmacodynamic readout” (Leyton et al. 2005).
3.3. IMPEDIMENTS TO PROGRESS AND CURRENT AND FUTURE NEEDS
As described above, nuclear medicine imaging has the potential to further improve patient care in a variety of ways. However, the actual number of new radiotracers introduced into clinical practice over the past 10 years has been very limited. For example, although this chapter discusses a significant number of PET agents, only one agent (FDG) is used in more than 95 percent of all clinical PET studies. It is clear that the transfer of promising radiopharmaceutical and molecular probes from small animals to humans faces considerable hurdles. In part, this reflects the uncertain path that any drug must follow as it goes from discovery into application. For radiopharmaceuticals there are the added economic concerns of the relatively small market, even for common indications such as cancer. Some specific barriers are listed below.
- Regulatory Impediments. Taken together, the regulatory impediments that limit approval and reimbursement for novel radiopharmaceuticals are the most important barrier to the continuing development and introduction of novel radiopharmaceuticals into clinical nuclear medicine practice. For example, the 1997 FDA Modernization Act, and the congressional and regulatory action that accompanied the enacting of this legislation, made possible the implementation of FDG-PET imaging as a clinical reality. A time table was proposed in the act, according to which more complete regulatory guidance should have been developed by the Food and Drug Administration (FDA) for facilitated regulation within 2 years. We are at the 10-year mark now, and no new regulation has yet been enacted to deal with the special features required for review and approval, or with clearance for reimbursement of novel nuclear medicine imaging procedures. This lack of clarity about process limits incentive to develop new agents and discourages commercial investment. It would be helpful if more spe cific guidance for diagnostic imaging drugs could be developed soon by the FDA.The recent introduction of the exploratory investigational new drug (eIND) by the FDA will likely help in bringing new radiotracers into the human environment; the eIND limits the requirements for extensive toxicology testing, which, given the long history of safety of tracer procedures, may be excessive. Additional benefits would come from targeted support for phase 0 and phase I clinical trials of new agents within academic centers, which are still the most likely sites for development of new agents. The growing complexities of clinical regulations for early clinical trials for diagnostic imaging agents pose considerable financial burdens that are frequently beyond the means of investigators in academia. In the past, such costs were often defrayed by diversion of clinician income. However, with continued reductions in reimbursement, increased competition between imaging centers for patients, and increasing administrative and personnel costs, clinical practice resources have declined and are no longer sufficient to support initial clinical evaluation studies of new molecular probes.The need for patient confidentiality and for protection and safety of human subjects is acknowledged by investigators, but regulatory and oversight requirements by institutional review boards6 are, at times, excessive. Associated administrative burdens, together with excessive delays for institutional review and approval of study protocols have impeded clinical research. Stringent confidentiality requirements, such as those mandated by the Health Insurance Portability and Accountability Act,7 pose additional difficulties (DHHS 2003). In many instances, these requirements have precluded long-term follow-up studies of patients, which are needed to assess the efficacy of new diagnostic approaches. There is growing concern that these regulatory hurdles have impeded translational research to the extent that some major clinical investigations have migrated from the United States to other countries with less stringent requirements.
- Limited Radiotracer Availability and Distribution. Radiotracers for nuclear medicine imaging are supplied on a dose basis through networks of radiopharmacies and radiopharmaceutical distribution centers. Most radiopharmaceutical distribution centers in the United States are located within a less than 100-mile radius of nuclear medicine imaging facilities. This allows for a steady and reliable supply of radiotracers including those labeled with relatively short-lived positron emitting radionuclides such as fluorine-18-deoxyglucose (110-min physical half-life). Supply of compounds labeled with radionuclides of shorter physical half-lives (e.g., 10 to 30 min) is, however, not possible through these distribution centers. Their use is therefore confined to institutions capable of onsite radionuclide production and radiotracer chemistry. Labeling of these radiotracers with longer lived radionuclides such as fluorine-18 will be important, because it will provide greater clinical availability and use. This impediment is further explored in Chapters 5 and 6.
- Need for Standardization and Harmonization of Nuclear Imaging Procedures. Procedural aspects of nuclear medicine imaging vary, at times greatly, across institutions and thus may complicate or in some instances even preclude meaningful assessments of the clinical value and efficacy of nuclear medicine imaging. Examples of the characteristics that vary include the timing of image acquisition after radiotracer administration, data handling, and data storage. Accordingly, there is a need for greater uniformity of nuclear medicine imaging, including universally accepted image-derived measures of regional tissue function. Standardization of imaging study protocols, of image formatting, data handling, and data storage, as well as of image-derived parameters, will be especially critical for design and performance of multi-center clinical trials for drug evaluation and determination of efficacy of newly developed imaging approaches.
Footnotes
- 1
Amino acids are the building blocks of proteins.
- 2
In medicine, hypoxia refers to a shortage of oxygen in the body.
- 3
In chemistry, an analog refers to a substance which is similar in structure to another substance.
- 4
Coronary artery disease is caused by inadequate blood supply to the heart. This is generally caused by the narrowing or partially blockage of the arteries. Undetected or untreated coronary artery disease can lead to serious complications, such as a heart attack.
- 5
Atherosclerosis is a disease of the arteries in which fatty material builds up. The buildup is called plaque.
- 6
Institutional review boards are internal groups who review and monitor biomedical research being conducted in human subjects at a given institution.
- 7
The Health Insurance Portability and Accountability Act of 1996 mandated the adoption of Federal privacy protections for individually identifiable health information.
- Nuclear Medicine Imaging in Diagnosis and Treatment - Advancing Nuclear Medicine...Nuclear Medicine Imaging in Diagnosis and Treatment - Advancing Nuclear Medicine Through Innovation
- Chain C, Dual specificity mitogen-activated protein kinase kinase dSOR1Chain C, Dual specificity mitogen-activated protein kinase kinase dSOR1gi|2678057213|pdb|8BW9|CProtein
- Mch modifier of chinchilla [Mus musculus]Mch modifier of chinchilla [Mus musculus]Gene ID:104242Gene
Your browsing activity is empty.
Activity recording is turned off.
See more...