By agreement with the publisher, this book is accessible by the search feature, but cannot be browsed.
NCBI Bookshelf. A service of the National Library of Medicine, National Institutes of Health.
Purves D, Augustine GJ, Fitzpatrick D, et al., editors. Neuroscience. 2nd edition. Sunderland (MA): Sinauer Associates; 2001.
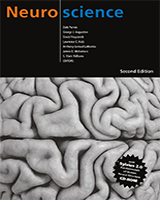
Neuroscience. 2nd edition.
Show detailsPostsynaptic conductance changes and the potential changes that accompany them alter the probability that an action potential will be produced in the postsynaptic cell. At the neuromuscular junction, synaptic action increases the probability that an action potential will occur in the postsynaptic muscle cell; indeed, the large amplitude of the EPP ensures that an action potential always is triggered. At many other synapses, PSPs actually decrease the probability that the postsynaptic cell will generate an action potential. PSPs are called excitatory (or EPSPs) if they increase the likelihood of a postsynaptic action potential occurring, and inhibitory (or IPSPs) if they decrease this likelihood. Given that most neurons receive inputs from both excitatory and inhibitory synapses, it is important to understand more precisely the mechanisms that determine whether a particular synapse excites or inhibits its postsynaptic partner.
The principles of excitation just described for the neuromuscular junction are pertinent to all excitatory synapses. The principles of postsynaptic inhibition are much the same as for excitation, and are also general. In both cases, neurotransmitters binding to receptors open or close ion channels in the postsynaptic cell. Whether a postsynaptic response is an EPSP or an IPSP depends on the type of channel that is coupled to the receptor, and on the concentration of permeant ions inside and outside the cell. In fact, the only factor that distinguishes postsynaptic excitation from inhibition is the reversal potential of the PSP in relation to the threshold voltage for generating action potentials in the postsynaptic cell.
Consider, for example, a neuronal synapse that uses glutamate as the transmitter. Many such synapses have receptors that, like the ACh receptors at neuromuscular synapses, open ion channels that are nonselectively permeable to cations. When these glutamate receptors are activated, both Na+ and K+ flow across the postsynaptic membrane. The reversal potential (Erev) for the postsynaptic current is approximately 0 mV, whereas the resting potential of neurons is approximately -60 mV. The resulting EPSP will depolarize the postsynaptic membrane potential, bringing it toward 0 mV. For the particular neuron shown in Figure 7.6A, the action potential threshold voltage is -40 mV. Thus, the EPSP increases the probability that the postsynaptic neuron will produce an action potential, defining this synapse as excitatory.

Figure 7.6
Reversal potentials and threshold potentials determine postsynaptic excitation and inhibition. (A) If the reversal potential for a PSP (0 mV) is more positive than the action potential threshold (-40 mV), the effect of a transmitter is excitatory, and (more...)
As an example of inhibitory postsynaptic action, consider a neuronal synapse that uses GABA as its transmitter. At such synapses, the GABA receptors typically open channels that are selectively permeable to Cl-. When these channels open, negatively charged chloride ions can flow across the membrane. Assume that the postsynaptic neuron has a resting potential of -60 mV and an action potential threshold of -40 mV, as in the previous example. If ECl is -70 mV, as is typical for many neurons, transmitter release at this synapse will inhibit the postsynaptic cell (because ECl is more negative than the action potential threshold). In this case, the electrochemical driving force (Vm - Erev) causes Cl- to flow into the cell, generating an outward PSC (because Cl- is negatively charged) and consequently a hyperpolarizing IPSP (Figure 7.6B). Because ECl is more negative than the action potential threshold, the conductance change arising from the binding of GABA keeps the postsynaptic membrane potential more negative than threshold, thereby reducing the probability that the postsynaptic cell will fire an action potential.
However, not all inhibitory synapses produce hyperpolarizing IPSPs. For instance, in the neuron just described, if ECl were -50 mV instead of -70 mV, then the synapse would still be inhibitory because the reversal potential of the IPSP remains more negative than the action potential threshold (-40 mV). Because the electrochemical driving force now causes Cl- to flow out of the cell, however, the IPSP is actually depolarizing (Figure 7.6C). Nonetheless, this depolarizing IPSP inhibits the postsynaptic cell because the cell's membrane potential is kept more negative than the threshold potential for action potential initiation. Another way to think about this peculiarity is that if another depolarizing input were to bring the cell's resting potential to -41 mV, just below threshold for firing an action potential, the opening of these GABA-activated channels would result in a hyperpolarizing current, bringing the membrane potential closer to -50 mV, the reversal potential for these channels. Thus, while EPSPs depolarize the postsynaptic cell, IPSPs can hyperpolarize or depolarize; indeed, an inhibitory conductance change may produce no potential change at all and still exert an inhibitory effect.
Although the particulars of postsynaptic action can be complex, a simple rule distinguishes postsynaptic excitation from inhibition: An EPSP has a reversal potential more positive than the action potential threshold, whereas an IPSP has a reversal potential more negative than threshold (Figure 7.6D). Intuitively, this rule can be understood by realizing that an EPSP will tend to depolarize the membrane potential so that it exceeds threshold, whereas an IPSP will always act to keep the membrane potential more negative than the threshold potential.
- Excitatory and Inhibitory Postsynaptic Potentials - NeuroscienceExcitatory and Inhibitory Postsynaptic Potentials - Neuroscience
Your browsing activity is empty.
Activity recording is turned off.
See more...