By agreement with the publisher, this book is accessible by the search feature, but cannot be browsed.
NCBI Bookshelf. A service of the National Library of Medicine, National Institutes of Health.
Gilbert SF. Developmental Biology. 6th edition. Sunderland (MA): Sinauer Associates; 2000.
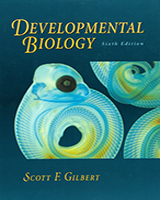
Developmental Biology. 6th edition.
Show detailsThere are two major ways of forming a neural tube. In primary neurulation, the cells surrounding the neural plate direct the neural plate cells to proliferate, invaginate, and pinch off from the surface to form a hollow tube. In secondary neurulation, the neural tube arises from a solid cord of cells that sinks into the embryo and subsequently hollows out (cavitates) to form a hollow tube. The extent to which these modes of construction are used varies among vertebrate classes. Neurulation in fishes is exclusively secondary. In birds, the anterior portions of the neural tube are constructed by primary neurulation, while the neural tube caudal to the twenty-seventh somite pair (i.e., everything posterior to the hindlimbs) is made by secondary neurulation (Pasteels 1937; Catala et al. 1996). In amphibians, such as Xenopus, most of the tadpole neural tube is made by primary neurulation, but the tail neural tube is derived from secondary neurulation (Gont et al. 1993). In mice (and probably humans, too), secondary neurulation begins at or around the level of somite 35 (Schoenwolf 1984; Nievelstein et al. 1993).
Primary neurulation
The events of primary neurulation in the chick and the frog are illustrated in Figures 12.3 and 12.4, respectively. During primary neurulation, the original ectoderm is divided into three sets of cells: (1) the internally positioned neural tube, which will form the brain and spinal cord, (2) the externally positioned epidermis of the skin, and (3) the neural crest cells. The neural crest cells form in the region that connects the neural tube and epidermis, but then migrate elsewhere; they will generate the peripheral neurons and glia, the pigment cells of the skin, and several other cell types.

Figure 12.3
Primary neurulation: neural tube formation in the chick embryo. (A, 1) Cells of the neural plate can be distinguished as elongated cells in the dorsal region of the ectoderm. Folding begins as the medial neural hinge point (MHP) cells anchor to notochord (more...)

Figure 12.4
Three views of neurulation in an amphibian embryo, showing early (left), middle (center), and late (right) neurulae in each case. (A) Looking down on the dorsal surface of the whole embryo. (B) Sagit-tal section through the medial plane of the embryo. (more...)
VADE MECUM
Chick Neurulation. By 33 hours of incubation, neurulation in the chick embryo is well underway. Both whole mounts and a complete set of serial cross sections through a 33-hour chick embryo are included in this segment so that you can see this amazing event. The serial sections can be displayed either as a continuum in movie format or individually, along with labels and color-coding that designates germ layers. [Click on Chick-Mid]
The process of primary neurulation appears to be similar in amphibians, reptiles, birds, and mammals (Gallera 1971). Shortly after the neural plate has formed, its edges thicken and move upward to form the neural folds, while a U-shaped neural groove appears in the center of the plate, dividing the future right and left sides of the embryo (see Figures 12.2C and 12.3). The neural folds migrate toward the midline of the embryo, eventually fusing to form the neural tube beneath the overlying ectoderm. The cells at the dorsalmost portion of the neural tube become the neural crest cells.
Neurulation occurs in somewhat different ways in different regions of the body. The head, trunk, and tail each form their region of the neural tube in ways that reflect the inductive relationship of the pharyngeal endoderm, prechordal plate, and notochord to its overlying ectoderm (Chapters 10 and 11). The head and trunk regions both undergo variants of primary neurulation, and this process can be divided into four distinct but spatially and temporally overlapping stages: (1) formation of the neural plate; (2) shaping of the neural plate; (3) bending of the neural plate to form the neural groove; and (4) closure of the neural groove to form the neural tube (Smith and Schoenwolf 1997; see Figure 12.2).
Formation and shaping of the neural plate
The process of neurulation begins when the underlying dorsal mesoderm (and pharyngeal endoderm in the head region) signals the ectodermal cells above it to elongate into columnar neural plate cells (Smith and Schoenwolf 1989; Keller et al. 1992 ). Their elongated shape distinguishes the cells of the prospective neural plate from the flatter pre-epidermal cells surrounding them. As much as 50% of the ectoderm is included in the neural plate. The neural plate is shaped by the intrinsic movements of the epidermal and neural plate regions. The neural plate lengthens along the anterior-posterior axis, narrowing itself so that subsequent bending will form a tube (instead of a spherical capsule).
In both amphibians and amniotes, the neural plate lengthens and narrows by convergent extension, intercalating several layers of cells into a few layers. In addition, the cell divisions of the neural plate cells are preferentially in the rostral-caudal (beak-tail; anterior-posterior) direction (Jacobson and Sater 1988; Schoenwolf and Alvarez 1989; Sausedo et al. 1997; see Figures 12.2 and 12.3). These events will occur even if the tissues involved are isolated. If the neural plate is isolated, its cells converge and extend to make a thinner plate, but fail to roll up into a neural tube. However, if the “border region” containing both presumptive epidermis and neural plate tissue is isolated, it will form small neural folds in culture (Jacobson and Moury 1995; Moury and Schoenwolf 1995).
WEBSITE
12.1 Formation of the floor plate cells. One of the major controversies in developmental neurobiology concerns the origin of the cells that form the ventral floor of the neural tube. It is possible that these cells are derived directly from the notochord and do not arise from the surface ectoderm. http://www.devbio.com/chap12/link1201.shtml
Bending of the neural plate
The bending of the neural plate involves the formation of hinge regions where the neural tube contacts surrounding tissues. In these regions, the presumptive epidermal cells adhere to the lateral edges of the neural plate and move them toward the midline (see Figure 12.3B). In birds and mammals, the cells at the midline of the neural plate are called the medial hinge point (MHP) cells. They are derived from the portion of the neural plate just anterior to Hensen's node and from the anterior midline of Hensen's node (Schoenwolf 1991a,b; Catala et al. 1996). The MHP cells become anchored to the notochord beneath them and form a hinge, which forms a furrow at the dorsal midline. The notochord induces the MHP cells to decrease their height and to become wedge-shaped (van Straaten et al. 1988; Smith and Schoenwolf 1989). The cells lateral to the MHP do not undergo such a change (Figures 12.3B,C). Shortly thereafter, two other hinge regions form furrows near the connection of the neural plate with the remainder of the ectoderm. These regions are called the dorsolateral hinge points (DLHPs), and they are anchored to the surface ectoderm of the neural folds. These cells, too, increase their height and become wedge-shaped.
Cell wedging is intimately linked to changes in cell shape. In the DLHPs, microtubules and microfilaments are both involved in these changes. Colchicine, an inhibitor of microtubule polymerization, inhibits the elongation of these cells, while cytochalasin B, an inhibitor of microfilament formation, prevents the apical constriction of these cells, thereby inhibiting wedge formation (Burnside 1973; Karfunkel 1972; Nagele and Lee 1987). After the initial furrowing of the neural plate, the plate bends around these hinge regions. Each hinge acts as a pivot that directs the rotation of the cells around it (Smith and Schoenwolf 1991).
Meanwhile, extrinsic forces are also at work. The surface ectoderm of the chick embryo pushes toward the midline of the embryo, providing another motive force for the bending of the neural plate (see Figure 12.3C; Alvarez and Schoenwolf 1992). This movement of the presumptive epidermis and the anchoring of the neural plate to the underlying mesoderm may also be important for ensuring that the neural tube invaginates into the embryo and not outward. If small pieces of neural plate are isolated from the rest of the embryo (including the mesoderm), they tend to roll inside out (Schoenwolf 1991a). The pushing of the presumptive epidermis toward the center and the furrowing of the neural tube creates the neural folds.
Closure of the neural tube
The neural tube closes as the paired neural folds are brought together at the dorsal midline. The folds adhere to each other, and the cells from the two folds merge. In some species, the cells at this junction form the neural crest cells. In birds, the neural crest cells do not migrate from the dorsal region until after the neural tube has been closed at that site. In mammals, however, the cranial neural crest cells (which form facial and neck structures) migrate while the neural folds are elevating (i.e., prior to neural tube closure), whereas in the spinal cord region, the crest cells wait until closure has occurred (Nichols 1981; Erickson and Weston 1983).
The closure of the neural tube does not occur simultaneously throughout the ectoderm. This is best seen in those vertebrates (such as birds and mammals) whose body axis is elongated prior to neurulation. Figure 12.5 depicts neurulation in a 24-hour chick embryo. Neurulation in the cephalic (head) region is well advanced, while the caudal (tail) region of the embryo is still undergoing gastrulation. Regionalization of the neural tube also occurs as a result of changes in the shape of the tube. In the cephalic end (where the brain will form), the wall of the tube is broad and thick. Here, a series of swellings and constrictions define the various brain compartments. Caudal to the head region, however, the neural tube remains a simple tube that tapers off toward the tail. The two open ends of the neural tube are called the anterior neuropore and the posterior neuropore.

Figure 12.5
Stereogram of a 24-hour chick embryo. Cephalic portions are finishing neurulation while the caudal portions are still undergoing gastrulation. (From Patten 1971; after Huettner 1949.)
Unlike neurulation in chicks (in which neural tube closure is initiated at the level of the future midbrain and “zips up” in both directions), neural tube closure in mammals is initiated at several places along the anterior-posterior axis (Golden and Chernoff 1993; Van Allen et al. 1993). Different neural tube defects are caused when various parts of the neural tube fail to close (Figure 12.6). Failure to close the human posterior neural tube regions at day 27 (or the subsequent rupture of the posterior neuropore shortly thereafter) results in a condition called spina bifida, the severity of which depends on how much of the spinal cord remains exposed. Failure to close the anterior neural tube regions results in a lethal condition, anencephaly. Here, the forebrain remains in contact with the amniotic fluid and subsequently degenerates. Fetal forebrain development ceases, and the vault of the skull fails to form. The failure of the entire neural tube to close over the entire body axis is called craniorachischisis. Collectively, neural tube defects are not rare in humans, as they are seen in about 1 in every 500 live births. Neural tube closure defects can often be detected during pregnancy by various physical and chemical tests.

Figure 12.6
Neurulation in the human embryo. (A) Dorsal and transverse sections of a 22-day human embryo initiating neurulation. Both anterior and posterior neuropores are open to the amniotic fluid. (B) Dorsal view of a neurulating human embryo a day later. The (more...)
Human neural tube closure requires a complex interplay between genetic and environmental factors. Certain genes, such as Pax3, sonic hedgehog, and openbrain, are essential for the formation of the mammalian neural tube, but dietary factors, such as cholesterol and folic acid, also appear to be critical. It has been estimated that 50% of human neural tube defects could be prevented by a pregnant woman's taking supplemental folic acid (vitamin B12), and the U.S. Public Health Service recommends that all women of childbearing age take 0.4 mg of folate daily to reduce the risk of neural tube defects during pregnancy (Milunsky et al. 1989; Czeizel and Dudas 1992; Centers for Disease Control 1992).
The neural tube eventually forms a closed cylinder that separates from the surface ectoderm. This separation is thought to be mediated by the expression of different cell adhesion molecules. Although the cells that will become the neural tube originally express E-cadherin, they stop producing this protein as the neural tube forms, and instead synthesize N-cadherin and N-CAM (Figure 12.7). As a result, the surface ectoderm and neural tube tissues no longer adhere to each other. If the surface ectoderm is experimentally made to express N-cadherin (by injecting N-cadherin mRNA into one cell of a 2-cell Xenopus embryo), the separation of the neural tube from the presumptive epidermis is dramatically impeded (Detrick et al. 1990; Fujimori et al. 1990).

Figure 12.7
Expression of N-cadherin and E-cadherin adhesion proteins during neurulation in Xenopus. (A) Normal development. In the neural plate stage, N-cadherin is seen in the neural plate, while E-cadherin is seen on the presumptive epidermis. Eventually, the (more...)
WEBSITE
12.2 Neural tube closure. The closing of the neural tube is a complex event that can be influenced by both genes and environment. The interactions between genetic and environmental factors are now being untangled. http://www.devbio.com/chap12/link1202.shtml
Secondary neurulation
Secondary neurulation involves the making of a medullary cord and its subsequent hollowing into a neural tube (Figure 12.8). Knowledge of the mechanisms of secondary neurulation may be important in medicine, given the prevalence of human posterior spinal cord malformations.

Figure 12.8
Secondary neurulation in the caudal region of a 25-somite chick embryo. (A) The medullary cord forming at the most caudal end of the chick tailbud. (B) The medullary cord at a slightly more anterior position in the tailbud. (C) The neural tube is cavitating (more...)
In frogs and chicks, secondary neurulation is usually seen in the neural tube of the lumbar (abdominal) and tail vertebrae. In both cases, it can be seen as a continuation of gastrulation. In the frog, instead of involuting into the embryo, the cells of the dorsal blastopore lip keep growing ventrally (Figure 12.9A, B). The growing region at the tip of the lip is called the chordoneural hinge (Pasteels 1937), and it contains precursors for both the posteriormost portion of the neural plate and the posterior portion of the notochord. The growth of this region converts the roughly spherical gastrula, 1.2 mm in diameter, into a linear tadpole some 9 mm long. The tip of the tail is the direct descendant of the dorsal blastopore lip, and the cells lining the blastopore form the neurenteric canal. The proximal part of the neurenteric canal fuses with the anus, while the distal portion becomes the ependymal canal (i.e., the lumen of the neural tube) (Figure 12.9C; Gont et al. 1993).

Figure 12.9
Movements of cells during secondary neurulation in Xenopus. (A) Involution of the mesoderm at the mid-gastrula stage. (B) Movements of the dorsal blastopore lip at the late gastrula/early neurula stage. Involution has ceased, and both the ectoderm and (more...)
- Formation of the Neural Tube - Developmental BiologyFormation of the Neural Tube - Developmental Biology
- BioProject Links for Protein (Select 2098123077) (1)BioProject
- Drosophila teissieri strain:GT53wDrosophila teissieri strain:GT53wDrosophila teissieri strain:GT53w RefSeq Genome sequencing and assemblyBioProject
Your browsing activity is empty.
Activity recording is turned off.
See more...