By agreement with the publisher, this book is accessible by the search feature, but cannot be browsed.
NCBI Bookshelf. A service of the National Library of Medicine, National Institutes of Health.
Gilbert SF. Developmental Biology. 6th edition. Sunderland (MA): Sinauer Associates; 2000.
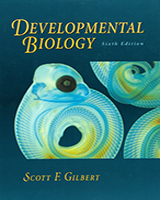
Developmental Biology. 6th edition.
Show detailsIn juxtacrine interactions, proteins from the inducing cell interact with receptor proteins of adjacent responding cells. The inducer does not diffuse from the cell producing it. There are three types of juxtacrine interactions. In the first type, a protein on one cell binds to its receptor on the adjacent cell. We saw this type of juxtacrine interaction when we discussed the interaction between the Bride of sevenless protein and its receptor, Sevenless. In the second type, a receptor on one cell binds to its ligand on the extracellular matrix secreted by another cell. In the third type, the signal is transmitted directly from the cytoplasm of one cell through small conduits into the cytoplasm of an adjacent cell.
The Notch pathway: Juxtaposed ligands and receptors
While most known regulators of induction are diffusible proteins, some inducing proteins remain bound to the inducing cell surface. In one such pathway, cells expressing the Delta, Jagged, or Serrate proteins in their cell membranes activate neighboring cells that contain the Notch protein in their cell membranes. Notch extends through the cell membrane, and its external surface contacts Delta, Jagged, or Serrate proteins extending out from an adjacent cell. When complexed to one of these ligands, Notch undergoes a conformational change that enables it to be cut by a protease. The cleaved portion enters the nucleus and binds to a dormant transcription factor of the CSL family. When bound to the Notch protein, the CSL transcription factors activate their target genes (Figure 6.29A; Lecourtois and Schweisguth 1998; Schroeder et al. 1998; Struhl and Adachi 1998 ).

Figure 6.29
Mechanism of Notch activity. (A) Model for the activation of Notch. A ligand (Delta, Jagged, or Serrate protein) on one cell binds to the extracellular domain of the Notch protein on an adjacent cell. This binding causes a shape change in the intracellular (more...)
Notch proteins are extremely important receptors in the nervous system. In both the vertebrate and Drosophila nervous system, the binding of Delta to Notch tells the receiving cell not to become neural (Figure 6.29B; Chitnis et al. 1995; Wang et al. 1998). In the vertebrate eye, the interactions between Notch and its ligands seem to regulate which cells become optic neurons and which become glial cells (Dorsky et al. 1997; Wang et al. 1998).
WEBSITE
6.8 Notch mutations. Mutations in Notch proteins can cause nervous system abnormalities in humans. Humans have more than one Notch gene and more than one ligand. Their interactions may be critical in neural development. http://www.devbio.com/chap06/link0608.shtml
The extracellular matrix as a source of critical developmental signals
Proteins and functions of the extracellular matrix
The extracellular matrix consists of macromolecules secreted by cells into their immediate environment. These macromolecules form a region of noncellular material in the interstices between the cells. The extracellular matrix is a critical region for much of animal development. Cell adhesion, cell migration, and the formation of epithelial sheets and tubes all depend on the ability of cells to form attachments to extracellular matrices. In some cases, as in the formation of epithelia, these attachments have to be extremely strong. In other instances, as when cells migrate, attachments have to be made, broken, and made again. In some cases, the extracellular matrix merely serves as a permissive substrate to which cells can adhere, or upon which they can migrate. In other cases, it provides the directions for cell movement or the signal for a developmental event.
Extracellular matrices are made up of collagen, proteoglycans, and a variety of specialized glycoprotein molecules, such as fibronectin and laminin. These large glycoproteins are responsible for organizing the matrix and cells into an ordered structure. Fibronectin is a very large (460-kDa) glycoprotein dimer synthesized by numerous cell types. One function of fibronectin is to serve as a general adhesive molecule, linking cells to one another and to other substrates such as collagen and proteoglycans. Fibronectin has several distinct binding sites, and their interaction with the appropriate molecules results in the proper alignment of cells with their extracellular matrix (Figure 6.32). As we will see in later chapters, fibronectin also has an important role in cell migration. The “roads” over which certain migrating cells travel are paved with this protein. Fibronectin paths lead germ cells to the gonads and lead heart cells to the midline of the embryo. If chick embryos are injected with antibodies to fibronectin, the heart-forming cells fail to reach the midline, and two separate hearts develop (Heasman et al. 1981; Linask and Lash 1988).

Figure 6.32
Fibronectin in the developing frog embryo. (A) Fluorescent antibodies to fibronectin show fibronectin deposition as a green band in the Xenopus embryo during gastrulation. The fibronectin will orient the mesoderm movements of the cells. (B) Structure (more...)
Laminin and type IV collagen are major components of a type of extracellular matrix called the basal lamina (Figure 6.33). This basal lamina is characteristic of the closely knit sheets that surround epithelial tissue. The adhesion of epithelial cells to laminin (upon which they sit) is much greater than the affinity of mesenchymal cells for fibronectin (to which they must bind and release if they are to migrate). Like fibronectin, laminin plays a role in assembling the extracellular matrix, promoting cell adhesion and growth, changing cell shape, and permitting cell migration (Hakamori et al. 1984).

Figure 6.33
Location and formation of extracellular matrices in the chick embryo. The scanning electron micrograph shows the extracellular matrix at the junction of the epithelial cells (above) and mesenchymal cells (below). The epithelial cells synthesize a tight, (more...)
Integrins, the cell receptors for extracellular matrix molecules
The ability of a cell to bind to adhesive glycoproteins depends on its expressing a cell membrane receptor for the cell-binding site of these large molecules. The main fibronectin receptors were identified by using antibodies that block the attachment of cells to fibronectin (Chen et al. 1985; Knudsen et al. 1985). The fibronectin receptor complex was found not only to bind fibronectin on the outside of the cell, but also to bind cytoskeletal proteins on the inside of the cell. Thus, the fibronectin receptor complex appears to span the cell membrane and unite two types of matrices. On the outside of the cell, it binds to the fibronectin of the extracellular matrix; on the inside of the cell, it serves as an anchorage site for the actin microfilaments that move the cell (Figure 6.34). Horwitz and co-workers 1986; Tamkun et al. 1986) have called this family of receptor proteins integrins because they integrate the extracellular and intracellular scaffolds, allowing them to work together. On the extracellular side, integrins bind to the sequence arginine-glycine-aspartate (RGD), found in several adhesive proteins in extracellular matrices, including fibronectin, vitronectin (found in the basal lamina of the eye), and laminin (Ruoslahti and Pierschbacher 1987). On the cytoplasmic side, integrins bind to talin and α-actinin, two proteins that connect to actin microfilaments. This dual binding enables the cell to move by contracting the actin microfilaments against the fixed extracellular matrix.

Figure 6.34
Speculative diagram relating the binding of cytoskeleton to the extracellular matrix through the integrin molecule. (After Luna and Hitt 1992.)
Bissell and her colleagues (1982; Martins-Green and Bissell 1995) have proposed that the extracellular matrix is capable of inducing specific gene expression in developing tissues, especially those of the liver, testis, and mammary gland, in which the induction of specific transcription factors depends on cell-substrate binding (Figure 6.35; Liu et al. 1991; Streuli et al. 1991; Notenboom et al. 1996). Often, the presence of bound integrin prevents the activation of genes that specify apoptosis (Montgomery et al. 1994; Frisch and Ruoslahti 1997). The chondrocytes that produce the cartilage of our vertebrae and limbs can survive and differentiate only if they are surrounded by an extracellular matrix and are joined to that matrix through their integrins (Hirsch et al. 1997). If chondrocytes from the developing chick sternum are incubated with antibodies that block the binding of integrins to the extracellular matrix, they shrivel up and die. While the mechanisms by which bound integrins inhibit apoptosis remain controversial (see Howe et al. 1998), the extracellular matrix is obviously an important source of signals that can be transduced into the nucleus to produce specific gene expression.

Figure 6.35
Role of the extracellular matrix in cell differentiation. Light micrographs of rat Sertoli testis cells grown for two weeks (A) on tissue culture plastic dishes and (B) on dishes coated with basal lamina. The two photographs were taken at the same magnification, (more...)
Some of the genes induced by matrix attachment are being identified. When plated onto tissue culture plastic, mouse mammary gland cells will divide (Figure 6.36). Indeed, the genes for cell division (c-myc, cyclinD1) are expressed, while the genes for the differentiated products of the mammary gland (casein, lactoferrin, whey acidic protein) are not expressed. If the same cells are plated onto plastic coated with a laminin-containing basement membrane, the cells stop dividing and the differentiated genes of the mammary gland are expressed. This happens only after the integrins of the mammary gland cells bind to the laminin of the extracellular basement membrane. Then the gene for lactoferrin is expressed, as is the gene for p21, a cell division inhibitor. The c-myc and cyclinD1 genes become silent. Eventually, all the genes for the developmental products of the mammary gland are expressed and the cell division genes remain turned off. At this time, the mammary cells will have enveloped themselves in the basement membrane, forming a secretory epithelium reminiscent of the mammary gland tissue. The binding of integrins to laminin is essential for the transcription of the casein gene, and the integrins act in concert with prolactin (see Figure 6.21) to activate that gene's expression (Roskelley et al. 1994; Muschler et al. 1999).

Figure 6.36
Basement membrane-directed gene expression in mammary gland tissue. (A) Mouse mammary gland tissue divides when placed on tissue culture plastic. Cell division genes are on, and the genes capable of synthesizing the differentiated products of the mammary (more...)
Recent studies have shown that the binding of integrins to the extracellular matrix can stimulate the RTK-Ras pathway. When an integrin on the cell membrane of one cell binds to the fibronectin or collagen secreted by a neighboring cell, the integrin can activate the tyrosine kinase cascade through an adaptor protein-like complex that connects the integrin to the Ras G protein (Figure 6.37A; Wary et al. 1998). Cadherins and other cell adhesion molecules can also transmit signals by “hijacking” the FGF receptors (Williams et al. 1994b; Clark and Brugge 1995). Cadherins, for example, are able to bind to the intramembrane region of FGF receptors and thereby dimerize these receptors just like the normal FGF ligands (Figure 6.37B; Williams et al. 1994a; Doherty et al. 1995).

Figure 6.37
Two types of activation by cell adhesion molecules. (A) Cell-substrate adhesion molecules such as integrins may transmit a signal from the cytoplasmic portion of the integrin protein to the Ras G protein through a cascade involving caveolin and Fyn proteins. (more...)
Direct transmission of signals through gap junctions
Throughout this chapter we have been discussing the reception of signals by cell membrane receptors. In these mechanisms, the receptor is in some manner altered by binding the ligand, so that its cytoplasmic domain transmits the signal into the cell. Another mechanism transmits small, soluble signals directly through the cell membrane, for the membrane is not continuous in all places. There are regions called gap junctions that serve as communication channels between adjacent cells (Figure 6.38A,B). Cells so linked are said to be “coupled,” and small molecules (molecular weight <1500) and ions can freely pass from one cell to the other. In most embryos, at least some of the early blastomeres are connected by gap junctions. The ability of cells to form gap junctions with some cells and not with others creates physiological “compartments” within the developing embryo (Figure 6.38C).

Figure 6.38
Gap junctions. (A) Electron micrograph of a row of gap junctions connecting two apposed cells. (B) Fluorescence micrograph of gap junctions in a 17-day embryonic mouse kidney tubule. (C) Compartments formed by gap junction proteins between cells that (more...)
The gap junction channels are made of connexin proteins. In each cell, six identical connexins in the membrane group together to form a transmembrane channel containing a central pore. The channel complex of one cell connects to the channel complex of another cell, enabling the cytoplasms of both cells to be joined (Figure 6.38D). Different types of connexin proteins have separate, but overlapping, roles in normal development. For example, connexin-43 is found throughout the developing mouse embryo, in nearly every tissue. However, if the connexin-43 genes are knocked out by gene targeting, the mouse embryo will still develop. It appears that most of the functions of the connexin-43 protein can be taken over by other related connexin proteins—but not all. Shortly after birth, connexin-43-deficient mice take gasping breaths, turn bluish, and die. Autopsies of these mice show that the right ventricle—the chamber that pumps blood through the pulmonary artery to the lungs—is filled with tissue that occludes the chamber and obstructs blood flow (Reaume et al. 1995; Huang et al. 1998). Although loss of the connexin-43 protein can be compensated for in many tissues, it appears to be critical for normal heart development.
The importance of gap junctions in development has been demonstrated in amphibian and mammalian embryos (Warner et al. 1984). When antibodies to connexins were microinjected into one specific cell of an 8-cell Xenopus blastula, the progeny of that cell, which are usually coupled through gap junctions, could no longer pass ions or small molecules from cell to cell. Moreover, the tadpoles that resulted from such treated blastulae showed defects specifically relating to the developmental fate of the injected cell (Figure 6.39). The progeny of the injected cell did not die, but they were unable to develop normally (Warner et al. 1984). In the mouse embryo, the first eight blastomeres are also connected to one another by gap junctions. Although loosely associated with one another, these eight cells move together to form a compacted embryo. If compaction is inhibited by antibodies against connexins, the treated blastomeres continue to divide, but further development ceases (Lo and Gilula 1979; Lee et al. 1987). If antisense RNA to connexin messages is injected into one of the blastomeres of a normal mouse embryo, that cell will not form gap junctions and will not be included in the embryo (Bevilacqua et al. 1989).

Figure 6.39
Developmental effects of gap junctions. Section through Xenopus tadpoles in which one of the blastomeres at the 8-cell stage was injected with (A) a control antibody or (B) an antibody against connexins. The side formed by the injected blastomere lacks (more...)
WEBSITE
6.9 Connexin mutations. Mutations in human connexin proteins cause congenital malformations of the heart and ear. In many cases, one connexin can substitute for another, but when the connexins cannot compensate, a mutant phenotype results. http://www.devbio.com/chap06/link0609.shtml
- Juxtacrine Signaling - Developmental BiologyJuxtacrine Signaling - Developmental Biology
Your browsing activity is empty.
Activity recording is turned off.
See more...