By agreement with the publisher, this book is accessible by the search feature, but cannot be browsed.
NCBI Bookshelf. A service of the National Library of Medicine, National Institutes of Health.
Gilbert SF. Developmental Biology. 6th edition. Sunderland (MA): Sinauer Associates; 2000.
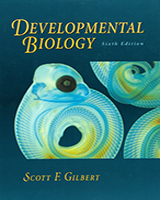
Developmental Biology. 6th edition.
Show detailsAlthough fertilization is often depicted as merely the means to merge two haploid nuclei, it has an equally important role in initiating the processes that begin development. These events happen in the cytoplasm and occur without the involvement of the nuclei.*
The mature sea urchin egg is a metabolically sluggish cell that is activated by the sperm. This activation is merely a stimulus, however; it sets into action a preprogrammed set of metabolic events. The responses of the egg to the sperm can be divided into “early” responses, which occur within seconds of the cortical reaction, and “late” responses, which take place several minutes after fertilization begins (Table 7.1; Figure 7.27).
Table 7.1
Events of sea urchin fertilization.

Figure 7.27
Model of possible pathway of egg activation in the sea urchin. (After Epel 1980; L. A. Jaffe, personal communication.)
Early responses
As we have seen, contact between sea urchin sperm and egg activates the two major blocks to polyspermy: the fast block, initiated by sodium influx into the cell, and the slow block, initiated by the intracellular release of calcium ions. The activation of all eggs appears to depend on an increase in the concentration of free calcium ions within the egg. Such an increase can occur in two ways: calcium ions can enter the egg from outside, or calcium ions can be released from the endoplasmic reticulum within the egg. Both mechanisms are used to different degrees in different species. In snails and worms, much of the calcium probably enters the egg from outside, while in fishes, frogs, sea urchins, and mammals, most of the calcium ions probably come from the endoplasmic reticulum. In both cases, a wave of calcium ions sweeps across the egg, beginning at the site of sperm-egg fusion (Jaffe 1983; Terasaki and Sardet 1991).
The presence of calcium ions is essential for activating the development of the embryo. If the calcium-chelating chemical EGTA is injected into the sea urchin egg, there is no cortical granule reaction, no change in membrane resting potential, and no reinitiation of cell division (Kline 1988). Conversely, eggs can be activated artificially in the absence of sperm by procedures that release free calcium into the oocyte. Steinhardt and Epel (1974) found that injection of micromolar amounts of the calcium ionophore A23187 into a sea urchin egg elicits most of the responses characteristic of a normally fertilized egg. The elevation of the fertilization envelope, a rise of intracellular pH, a burst of oxygen utilization, and increases in protein and DNA synthesis are all generated in their proper order. In most of these cases, development ceases before the first mitosis because the egg is still haploid and lacks the sperm centriole needed for division. Calcium release activates a series of metabolic reactions (Figure 7.27). One of these is the activation of the enzyme NAD+ kinase, which converts NAD+ to NADP+ (Epel et al. 1981). This change may have important consequences for lipid metabolism, since NADP+ (but not NAD+) can be used as a coenzyme for lipid biosynthesis. Thus, the conversion of NAD+ to NADP+ may be important in the construction of the many new cell membranes required during cleavage. Another effect of calcium release involves oxygen consumption. A burst of oxygen reduction (to hydrogen peroxide) is seen during fertilization, and much of this “respiratory burst” is used to crosslink the fertilization envelope. The enzyme responsible for this reduction of oxygen is also NADPH-dependent (Heinecke and Shapiro 1989). Lastly, NADPH helps regenerate glutathione and ovothiols, which may be crucial for scavenging free radicals that could otherwise damage the DNA of the egg and early embryo (Mead and Epel 1995).
Late responses
Shortly after the calcium ion levels rise in a sea urchin egg, its intracellular pH also increases.† The rise in intracellular pH begins with a second influx of sodium ions, which causes a 1:1 exchange between sodium ions from the seawater and hydrogen ions from the egg. This loss of hydrogen ions causes the pH to rise (Shen and Steinhardt 1978). It is thought that the pH increase and the calcium ion elevation act together to stimulate new protein synthesis and DNA synthesis (Winkler et al. 1980; Whitaker and Steinhardt 1982; Rees et al. 1995). If one experimentally elevates the pH of an unfertilized egg to a level similar to that of a fertilized egg, DNA synthesis and nuclear envelope breakdown ensue, just as if the egg were fertilized (Miller and Epel 1999).
The late responses of fertilization brought about by these ionic changes include the activation of DNA synthesis and protein synthesis. In sea urchins, a burst of protein synthesis usually occurs within several minutes after sperm entry. This protein synthesis does not depend on the synthesis of new messenger RNA; rather, it utilizes mRNAs already present in the oocyte cytoplasm (Figure 7.30; see Table 5.3). These messages include mRNAs encoding proteins such as histones, tubulins, actins, and morphogenetic factors that are utilized during early development. Such a burst of protein synthesis can be induced by artificially raising the pH of the cytoplasm using ammonium ions (Winkler et al. 1980).

Figure 7.30
A burst of protein synthesis at fertilization uses mRNAs stored in the oocyte cytoplasm. (A) Protein synthesis in embryos of the sea urchin Arbacia punctulata fertilized in the presence or absence of actinomycin D, an inhibitor of transcription. For the (more...)
The Activation of Gamete Metabolism
If calcium ion release is necessary for the activation of the oocyte, how does the sperm cause it to occur? We do not really know. As one investigator (Berridge 1993) stated, “Just how the sperm triggers the explosive release of calcium in the egg is still something of a mystery.” Recent data suggest that the production of inositol 1,4,5-trisphosphate (IP3) is the primary mechanism for releasing calcium ions from their intracellular storage.
The IP3 pathway is shown in Figure 7.28. The membrane phospholipid phosphatidylinositol 4,5-bisphosphate (PIP2) is split by the enzyme phospholipase C (PLC) to yield two active compounds: IP3 and diacylglycerol (DAG). IP3 is able to release calcium ions into the cytoplasm by opening the calcium ion channels of the endoplasmic reticulum. DAG activates protein kinase C, which in turn activates a protein that exchanges sodium ions for hydrogen ions, raising the pH of the egg (Swann and Whitaker 1986; Nishizuka 1986). This Na+/H+ exchange pump also needs calcium ions for activity. The result of PLC activation is therefore the liberation of calcium ions and the alkalinization of the egg, and both of the proteins it creates, IP3 and DAG, are involved in the initiation of development.
IP3 is formed at the site of sperm entry in sea urchin eggs, and can be detected within seconds of their being fertilized. The inhibition of IP3 synthesis prevents calcium release (Lee and Shen 1998), while injected IP3 can release sequestered calcium ions in sea urchin eggs, leading to the cortical granule reaction (Whitaker and Irvine 1984; Busa et al. 1985). Moreover, these IP3-mediated effects can be thwarted by preinjecting the egg with calcium-chelating agents (Turner et al. 1986).
IP3-responsive calcium channels have been found in the egg endoplasmic reticulum. The IP3 formed at the site of sperm entry is thought to bind to the IP3 receptors of these channels, effecting a local release of calcium (Ferris et al. 1989; Furuichi et al. 1989; Terasaki and Sardet 1991). Once released, the calcium ions can diffuse directly, or they can facilitate the release of more calcium ions by binding to calcium-sensitive receptors located in the cortical endoplasmic reticulum (McPherson et al. 1992). The binding of calcium ions to these receptors releases more calcium, and this released calcium binds to more receptors, and so on. The resulting wave of calcium release is propagated throughout the cell, starting at the point of sperm entry; and the cortical granules, which fuse with the cell membrane in the presence of high calcium concentrations, respond in a wave of exocytosis that follows the calcium ions. Mohri and colleagues (1995) have shown that IP3-released calcium is both necessary and sufficient for initiating the wave of calcium release.
IP3 is similarly found to release calcium ions in vertebrate eggs. As in sea urchins, waves of IP3 are thought to mediate calcium release from sites within the endoplasmic reticulum (Lechleiter and Clapham 1992; Miyazaki et al. 1992; Ayabe et al. 1995). Blocking the IP3 receptor in hamster eggs prevents the release of calcium at fertilization. Xu and colleagues (1994) found that blocking the IP3-mediated calcium release blocks every aspect of sperm-induced egg activation, including cortical granule exocytosis, mRNA recruitment, and cell cycle resumption.
The question then becomes, what initiates the production of IP3? In other words, what activates the phospholipase C enzymes? This question has not been easy to address, since (1) there are numerous types of PLC, (2) they can be activated through different pathways, and (3) different species can use different mechanisms to activate them. Results from recent studies of sea urchin eggs suggest that the active PLC is a member of the γ family of PLCs and is activated by a protein tyrosine kinase (Carroll et al. 1997, 1999; Williams et al. 1998).
WEBSITE
7.9 Biochemistry of egg activation. Inhibitors of phospholipase Cγ activity prevent the calcium ion flux across the echinoderm egg. This PLC is activated by protein kinases. This movie shows the evidence for this inhibition. http://www.devbio.com/chap07/link0709.shtml
The next question, then, is, what protein tyrosine kinase is activated? This question has not yet been satisfactorily answered. According to one model, the sperm receptor protein crosses the egg plasma membrane and has a protein tyrosine kinase activity in its cytoplasmic domain (Figure 7.29A). This structure would make it a classic receptor tyrosine kinase (see Chapter 5). However, the sequence of the putative bindin receptor reveals neither transmembrane nor kinase domains (Just and Lennarz 1997). According to a second model, the bindin receptor is linked to a protein tyrosine kinase and can activate the kinase, perhaps as a consequence of receptor crosslinking by the sperm (Figure 7.29B; see Giusti et al. 1999). A third possibility is that the activation of the IP3 pathway is caused not by the binding of sperm and egg, but by the fusion of the sperm and egg plasma membranes. McCulloh and Chambers (1992) have electrophysiological evidence that sea urchin egg activation does not occur until after sperm and egg cytoplasms are joined. They suggest that the egg-activating components are located on the sperm plasma membrane or in the cytoplasm. It is even possible that when the fusion of gamete membranes occurs, the sperm receptor tyrosine kinases (activated by the egg jelly to initiate the acrosomal reaction) activate the IP3 cascade for calcium release in the egg (see Gilbert 1994). In this scenario, shown in Figure 7.29C, bindin serves for cell-cell adhesion and membrane fusion, but not for signaling. Rather, the egg “activates itself” through the sperm.
Still another possibility is that the agent active in releasing the sequestered calcium comes from the sperm cytosol (Figure 17.29D). Support for the sperm’s carrying an activating molecule comes from the clinical procedure of intracytoplasmic sperm injection (ICSI) used to treat infertility when a man’s sperm count is low. Here, a single intact human sperm is injected directly into the cytoplasm of the egg. This results in egg activation, the formation of a male pronucleus, and normal embryonic development (Van Steirtinghem 1994). Kimura and colleagues (1998) have shown that the isolated head of a mouse sperm is capable of activating the mouse oocyte, and that the active portion of the sperm head appears to be the proteins surrounding the haploid nucleus. It is not known what role these perinuclear components may play in the normal physiology of egg activation.

Figure 7.28
The roles of inositol phosphates in initiating calcium release from the endoplasmic reticulum and the initiation of development. Phospholipase C splits PIP2 into IP3 and DAG. The IP3 releases calcium from the endoplasmic reticulum, and the DAG, with assistance (more...)

Figure 7.29
Possible mechanisms of egg activation. (A) The bindin receptor in the egg plasma membrane has tyrosine kinase activity. The tyrosine kinase activates PLC. (B) The bindin receptor activates a cytoplasmic tyrosine kinase. (C) An activated tyrosine kinase (more...)
Footnotes
- *
In certain salamanders, this developmental function of fertilization has been totally divorced from the genetic function. The silver salamander (Ambystoma platineum) is a hybrid subspecies consisting solely of females. Each female produces an egg with an unreduced chromosome number. This egg, however, cannot develop on its own, so the silver salamander mates with a male Jefferson salamander (A. jeffersonianum). The sperm from the male Jefferson salamander only stimulates the egg’s development; it does not contribute genetic material (Uzzell 1964). For details of this complex mechanism of procreation, see Bogart et al. 1989.
- †
Again, species-to-species variation is rampant. In the much smaller egg of the mouse, there is no elevation of pH after fertilization. Similarly in the mouse, there is no dramatic increase in protein synthesis immediately following fertilization (Ben-Yosef et al. 1996).
- The Activation of Egg Metabolism - Developmental BiologyThe Activation of Egg Metabolism - Developmental Biology
- Chain Q, 50S ribosomal protein L21eChain Q, 50S ribosomal protein L21egi|188596328|pdb|3CCU|QProtein
- rpl4p [Haloarcula marismortui ATCC 43049]rpl4p [Haloarcula marismortui ATCC 43049]Gene ID:3128386Gene
Your browsing activity is empty.
Activity recording is turned off.
See more...