By agreement with the publisher, this book is accessible by the search feature, but cannot be browsed.
NCBI Bookshelf. A service of the National Library of Medicine, National Institutes of Health.
Gilbert SF. Developmental Biology. 6th edition. Sunderland (MA): Sinauer Associates; 2000.
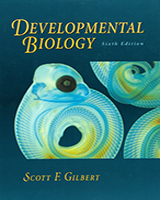
Developmental Biology. 6th edition.
Show detailsOnce in the gonad, the primordial germ cells continue to divide mitotically, producing millions of potential gamete precursors. The PGCs of both male and female gonads are then faced with the necessity of reducing their chromosomes from the diploid to the haploid condition. In the haploid condition, each chromosome is represented by only one copy, whereas diploid cells have two copies of each chromosome. To accomplish this reduction, the germ cells undergo meiosis.
After the germ cell's last mitotic division, a period of DNA synthesis occurs, so that the cell initiating meiosis doubles the amount of DNA in its nucleus. In this state, each chromosome consists of two sister chromatids attached at a common kinetochore (centromere). (In other words, the diploid nucleus contains four copies of each chromosome, but each chromosome is seen as two chromatids bound together.) Meiosis (shown in Figure 2.9) entails two cell divisions. In the first division, homologous chromosomes (e.g., the chromosome 3 pair in the diploid cell) come together and are then separated into different cells. Hence, the first meiotic division separates homologous chromosomes into two daughter cells such that each cell has only one copy of each chromosome. But each of the chromosomes has already replicated (i.e., each has two chromatids). The second meiotic division then separates the two sister chromatids from each other. Consequently, each of the four cells produced by meiosis has a single (haploid) copy of each chromosome.
The first meiotic division begins with a long prophase, which is subdivided into five stages. During the leptotene (Greek, “thin thread”) stage, the chromatin of the chromatids is stretched out very thinly, and it is not possible to identify individual chromosomes. DNA replication has already occurred, however, and each chromosome consists of two parallel chromatids. At the zygotene (Greek, “yoked threads”) stage, homologous chromosomes pair side by side. This pairing is called synapsis, and it is characteristic of meiosis. Such pairing does not occur during mitotic divisions. Although the mechanism whereby each chromosome recognizes its homologue is not known, synapsis seems to require the presence of the nuclear membrane and the formation of a proteinaceous ribbon called the synaptonemal complex. This complex is a ladderlike structure with a central element and two lateral bars (von Wettstein 1984; Schmekel and Daneholt 1995). The chromatin becomes associated with the two lateral bars, and the chromosomes are thus joined together (Figure 19.13). Examinations of meiotic cell nuclei with the electron microscope (Moses 1968; Moens 1969) suggest that paired chromosomes are bound to the nuclear membrane, and Comings (1968) has suggested that the nuclear envelope helps bring together the homologous chromosomes. The configuration formed by the four chromatids and the synaptonemal complex is referred to as a tetrad or a bivalent.

Figure 19.13
The synaptonemal complex. (A) Homologous chromosomes held together at the first meiotic prophase in a Neottiella oocyte. (B) Interpretive diagram of the synaptonemal complex structure. (A from von Wettstein 1971, courtesy of D. von Wettstein; B after (more...)
During the next stage of meiotic prophase, the chromatids thicken and shorten. This stage has therefore been called the pachytene (Greek, “thick thread”) stage. Individual chromatids can now be distinguished under the light microscope, and crossing-over may occur. Crossing-over represents exchanges of genetic material whereby genes from one chromatid are exchanged with homologous genes from another chromatid. Crossing-over may continue into the next stage, the diplotene (Greek, “double threads”) stage. Here, the synaptonemal complex breaks down, and the two homologous chromosomes start to separate. Usually, however, they remain attached at various places called chiasmata, which are thought to represent regions where crossing-over is occurring (Figure 19.14). The diplotene stage is characterized by a high level of gene transcription. In some species, the chromosomes of both male and female germ cells take on the “lampbrush” appearance characteristic of chromosomes that are actively making RNA (see below). During the next stage, diakinesis (Greek, “moving apart”), the centromeres move away from each other, and the chromosomes remain joined only at the tips of the chromatids. This last stage of meiotic prophase ends with the breakdown of the nuclear membrane and the migration of the chromosomes to the metaphase plate.

Figure 19.14
Chiasmata in diplotene bivalent chromosomes of salamander oocytes. Kinetochores are visible as darkly stained circles; arrows point to the two chiasmata. (Photograph courtesy of J. Kezer.)
During anaphase I, homologous chromosomes are separated from each other in an independent fashion. This stage leads to telophase I, during which two daughter cells are formed, each cell containing one partner of the homologous chromosome pair. After a brief interkinesis, the second division of meiosis takes place. During this division, the centromere of each chromosome divides during anaphase so that each of the new cells gets one of the two chromatids, the final result being the creation of four haploid cells. Note that meiosis has also reassorted the chromosomes into new groupings. First, each of the four haploid cells has a different assortment of chromosomes. In humans, in which there are 23 different chromosome pairs, there can be 223 (nearly 10 million) different types of haploid cells formed from the genome of a single person. In addition, the crossing-over that occurs during the pachytene and diplotene stages of prophase I further increases genetic diversity and makes the number of different gametes incalculable.
The events of meiosis appear to be coordinated through cytoplasmic connections between the dividing cells. Whereas the daughter cells formed by mitosis routinely separate from each other, the products of the meiotic cell divisions remain coupled to each other by cytoplasmic bridges. These bridges are seen during the formation of sperm and eggs throughout the animal kingdom (Pepling and Spradling 1998).
WEBSITE
19.3 Proteins involved in meiosis. The phenomenon of homologous pairing and crossing-over is being analyzed in several organisms and may involve DNA repair enzymes. http://www.devbio.com/chap19/link1903.shtml
WEBSITE
19.4 Human meiosis. Nondisjunction, the failure of chromosomes to sort properly during meiosis, is not uncommon in humans. Its frequency increases with maternal age. http://www.devbio.com/chap19/link1904.shtml
Big Decisions: Mitosis or Meiosis? Sperm or Egg?
In many species, the germ cells migrating into the gonad are bipotential and can differentiate into either sperm or ova, depending on their gonadal environment. When the ovaries of salamanders are experimentally transformed into testes, the resident germ cells cease their oogenic differentiation and begin developing as sperm (Burns 1930; Humphrey 1931). Similarly, in the housefly and mouse, the gonad is able to direct the differentiation of the germ cell (McLaren 1983; Inoue and Hiroyoshi 1986). Thus, in most organisms, the sex of the gonad and of its germ cells is the same.
But what about hermaphroditic animals, in which the change from sperm production to egg production is a naturally occurring physiological event? How is the same animal capable of producing sperm during one part of its life and oocytes during another part? Using Caenorhabditis elegans, Kimble and her colleagues identified two “decisions” that presumptive germ cells have to make. The first is whether to enter meiosis or to remain a mitotically dividing stem cell. The second is whether to become an egg or a sperm.
Recent evidence shows that these decisions are intimately linked. The mitotic/meiotic decision is controlled by a single nondividing cell at the end of each gonad, the distal tip cell. The germ cell precursors near this cell divide mitotically, forming the pool of germ cells; but as these cells get farther away from the distal tip cell, they enter meiosis. If the distal tip cell is destroyed by a focused laser beam, all the germ cells enter meiosis, and if the distal tip cell is placed in a different location in the gonad, germ line stem cells are generated near its new position (Figure 19.15; Kimble 1981; Kimble and White 1981). The distal tip cell extends long filaments that touch the distal germ cells. The extensions contain in their cell membranes the Lag-2 protein, a C. elegans homologue of Delta (Henderson et al. 1994; Tax et al. 1994; Hall et al. 1999). The Lag-2 protein maintains these germ cells in mitosis and inhibits their meiotic differentiation.
Austin and Kimble (1987) isolated a mutation that mimics the phenotype obtained when the distal tip cells are removed. It is not surprising that this mutation encodes Glp-1, the C. elegans homologue of Notch—the receptor for Delta. All the germ cell precursors of nematodes homozygous for the recessive mutation glp-1 initiate meiosis, leaving no mitotic population. Instead of the 1500 germ cells usually found in the fourth larval stage of hermaphroditic development, these mutants produce only 5 to 8 sperm cells. When genetic chimeras are made in which wild-type germ cell precursors are found within a mutant larva, the wild-type cells are able to respond to the distal tip cells and undergo mitosis. However, when mutant germ cell precursors are found within wild-type larvae, they all enter meiosis. Thus, the glp-1 gene appears to be responsible for enabling the germ cells to respond to the distal tip cell's signal.*
After the germ cells begin their meiotic divisions, they still must become either sperm or ova. Generally, in each ovotestis, the most proximal germ cells produce sperm, while the most distal (near the tip) become eggs (Hirsh et al. 1976). This means that the germ cells entering meiosis early become sperm, while those entering meiosis later become eggs. The genetics of this switch are currently being analyzed. The laboratories of Hodgkin (1985) and Kimble (Kimble et al. 1986) have isolated several genes needed for germ cell pathway selection, but the switch appears to involve the activity or inactivity of fem-3 mRNA. Figure 19.16 presents a scheme for how these genes might function. During early development, the fem genes, especially fem-3, are critical for the specification of sperm cells. Loss-of-function mutations of these genes convert XX nematodes into females (i.e., spermless hermaphrodites). As long as the FEM proteins are made in the germ cells, sperm are produced. The active fem genes are thought to activate the fog genes (whose loss-of-function mutations cause the feminization of the germ line and eliminate spermatogenesis). The fog gene products activate the genes involved in transforming the germ cell into sperm and also inhibit those genes that would otherwise direct the germ cells to initiate oogenesis.
Oogenesis can begin only when fem activity is suppressed. This suppression appears to act at the level of RNA translation. The 3´ untranslated region (3´ UTR) of the fem-3 mRNA contains a sequence that binds a repressor protein during normal development. If this region is mutated such that the repressor cannot bind, the fem-3 mRNA remains translatable, and oogenesis never occurs. The result is a hermaphrodite body that produces only sperm (Ahringer and Kimble 1991; Ahringer et al. 1992). The trans-acting repressor of the fem-3 message is a combination of the Nanos and Pumilio proteins (the same combination that represses hunchback message translation in Drosophila). The up-regulation of Pumilio expression may be critical in regulating the germ line switch from spermatogenesis to oogenesis, since Nanos is made constitutively. Nanos appears to be necessary in C. elegans (as it is in Drosophila) for the survival of all germ line cells (Kraemer et al. 1999). ▪
WEBSITE
19.5 Germ line sex determination inC. elegans. The establishment of whether a germ cell is to become a sperm or an egg involves multiple levels of inhibition. Translational regulation is seen in several of these steps. http://www.devbio.com/chap19/link1905.shtml

Figure 19.15
Regulation of the mitosis-or-meiosis decision by the distal tip cell of the C. elegans ovotestis. (A) Intact gonad early in development with regions of mitosis (light-colored cells) and meiosis. The plasma membranes of the distal tip cell's extensions (more...)

Figure 19.16
Model of sex determination switch in the germ line of C. elegans hermaphrodites. Sex determination in somatic tissues, showing a hierarchy of negative regulation. In the early larva, Pumilio is not synthesized, and the fem mRNA is able to be translated. (more...)
- *
The glp-1 gene appears to be involved in a number of inductive interactions in C. elegans. You will no doubt recall that glp-1 is also needed by the AB blastomere to receive inductive signals from the EMS blastomere to form pharyngeal muscles (see Chapter 8).
- Meiosis - Developmental BiologyMeiosis - Developmental Biology
Your browsing activity is empty.
Activity recording is turned off.
See more...