This work is licensed under a Creative Commons Attribution-NonCommercial-NoDerivatives 4.0 International License.
NCBI Bookshelf. A service of the National Library of Medicine, National Institutes of Health.
ABSTRACT
The insecticidal proteins produced by Bacillus thuringiensis (Bt) have provided a uniquely specific, safe, and effective tool for the control of a wide variety of insect pests. Bt has been used in spray formulations for over 40 years, where it is considered remarkably safe, in large part because specific formulations harm only a narrow range of insect species. Today, Bt insecticidal protein genes have been incorporated into several major crops where they provide a model for genetic engineering in agriculture.
Effective protection of crops from insect pests afforded by insecticidal proteins has had a number of positive impacts on agriculture. Reduced insect damage im-proves crop yield, reduces fungal toxins in the food supply, and improves the livelihood of farmers. Replacement of toxic chemical pesticides with Bt has reduced hazards to the environment and farm workers. Bt-engineered crops are equally amenable to use in large or small scale farming operations and compatible with other agricultural practices and technologies.
Concerns associated with the use of Bt include potential for harm to non-target organisms, development of resistance in populations of target insects, and, for engineered crops, possible ecological consequences of gene flow to non-engineered crops and wild relatives. These concerns merit continued attention on a case-by-case basis in order to ensure that Bt technologies have the maximum positive impact with a minimum risk on agriculture. Prudent use of Bt technologies will also be key in maintaining their usefulness for a long period of time.
Front Matter
The American Academy of Microbiology is grateful for the generous support of the following organizations:
- American Society for Microbiology
- Cornell University
- U.S. Environmental Protection Agency
- U.S. Food and Drug Administration
- National Science Foundation
- Rockefeller Foundation
The opinions expressed in this report are those solely of the colloquium participants.
This report and others from the American Academy of Microbiology are available online: www.asmusa.org
Board of Governors, American Academy of Microbiology
Eugene W. Nester, Ph.D. (Chair), University of Washington
Joseph M. Campos, Ph.D., Children's National Medical Center
R. John Collier, Ph.D., Harvard Medical School
Marie B. Coyle, Ph.D., University of Washington
James E. Dahlberg, Ph.D., University of Wisconsin, Madison
Julian E. Davies, Ph.D., Cubist Pharmaceuticals, Inc.
Arnold L. Demain, Ph.D., Drew University
Lucia B. Rothman-Denes, Ph.D., University of Chicago
Abraham L. Sonenshein, Ph.D., Tufts University Medical Center
David A. Stahl, Ph.D., University of Washington
Judy D. Wall, Ph.D., University of Missouri
Colloquium Steering Committee
Eugene W. Nester, Ph.D. (Co-Chair), University of Washington
Linda S. Thomashow, Ph.D. (Co-Chair), USDA/Washington State University
Luca Comai, Ph.D., University of Washington
Milton Gordon, Ph.D., University of Washington
Noel Keen, Ph.D., University of California, Riverside
Anne M. Vidaver, Ph.D., University of Nebraska
Carol A. Colgan, American Academy of Microbiology
Colloquium Participants
Michael Adang, Ph.D., University of Georgia
Illimar Altosaar, Ph.D., University of Ottawa
Arthur I. Aronson, Ph.D., Purdue University
Lee A. Bulla, Ph.D., University of Texas, Dallas
Christopher Cullis, Ph.D., National Science Foundation
Peter R. Day, Ph.D., Rutgers University
Donald H. Dean, Ph.D., Ohio State University
Elizabeth D. Earle, Ph.D., Cornell University
David J. Ellar, Ph.D., University of Cambridge, England
Brian A. Federici, Ph.D., University of California, Riverside
Sarjeet S. Gill, Ph.D., University of California, Riverside
Milton Gordon, Ph.D., University of Washington
Peter M. Kareiva, Ph.D., The Nature Conservancy
John D. Kemp, Ph.D., New Mexico State University
Pal Maliga, Ph.D., Rutgers University
Michelle Marvier, Ph.D., Santa Clara University
Sharlene Matten, Ph.D., U.S. Environmental Protection Agency
Matthew Metz, Ph.D., University of Washington
William Moar, Ph.D., Auburn University
Eugene W. Nester, Ph.D., University of Washington
Sheikh Riazzuddin, Ph.D., National Centre of Excellence in Molecular Biology, Lahore, Pakistan
Ronald R. Sederoff, Ph.D., North Carolina State University
Anthony M. Shelton, Ph.D., Cornell University
Allison Snow, Ph.D., Ohio State University
Diane Stanley-Horn, Ph.D., University of Guelph
Guenther Stotzky, Ph.D., New York University
Linda S. Thomashow, Ph.D., USDA/Washington State University
Executive Summary
The insecticidal proteins produced by Bacillus thuringiensis (Bt) have provided a uniquely specific, safe, and effective tool for the control of a wide variety of insect pests. Bt has been used in spray formulations for over 40 years, where it is considered remarkably safe, in large part because specific formulations harm only a narrow range of insect species. Today, Bt insecticidal protein genes have been incorporated into several major crops where they provide a model for genetic engineering in agriculture.
Effective protection of crops from insect pests afforded by insecticidal proteins has had a number of positive impacts on agriculture. Reduced insect damage im-proves crop yield, reduces fungal toxins in the food supply, and improves the livelihood of farmers. Replacement of toxic chemical pesticides with Bt has reduced hazards to the environment and farm workers. Bt-engineered crops are equally amenable to use in large or small scale farming operations and compatible with other agricultural practices and technologies.
Concerns associated with the use of Bt include potential for harm to non-target organisms, development of resistance in populations of target insects, and, for engineered crops, possible ecological consequences of gene flow to non-engineered crops and wild relatives. These concerns merit continued attention on a case-by-case basis in order to ensure that Bt technologies have the maximum positive impact with a minimum risk on agriculture. Prudent use of Bt technologies will also be key in maintaining their usefulness for a long period of time.
Background
Introduction of transgenic crops has provided new approaches to improving crop quality and productivity, but, at the same time, these crops have aroused concerns about the safety of agricultural biotechnology in relation to human health and the environment. These issues are, for the most part, relevant to agricultural practices in general, and are embodied in crops engineered to produce an insecticidal protein from the common bacterium Bacillus thuringiensis (Bt).
The insecticidal properties of B. thuringiensis have long been recognized. While some accounts indicate that the Egyptians were aware of the insecticidal properties of what probably was Bt and used it to control pests, the organism was first isolated about 100 years ago in Japan from silkworm larvae suffering from the disease “flacherie.” The organism was named Bacillus thuringiensis by Berliner in 1911, who isolated it from a diseased flour moth larva. In 1954, Angus demonstrated that the crystalline protein inclusions produced by B. thuringiensis in the course of sporulation were responsible for the insecticidal action.
Insecticidal products of Bt were first commercialized in France in the late 1930s. For over 40 years Bt has been applied to crops as an insecticidal spray, a mixture of spores and the associated protein crystals. By 1995, 182 Bt products were registered by the United States Environmental Protection Agency (EPA), but in 1999 Bt formulations constituted less than two percent (2%) of the total sales of all insecticides. The use of Bt has increased as insects have become resistant to chemical insecticides. Recently, the use of Bt in pest control has increased substantially through more widespread use by both conventional and “organic” food producers, as well as in forestry.
In 1987, three reports were published that demonstrated that insecticidal crystal protein (ICP) genes from Bt could be introduced and expressed in the tissues of tobacco and tomato, resulting in pest-resistant transgenic plants. By 2001, an estimated 69% of the cotton, 26% of the corn, and 68% of the soybeans grown in the United States were genetically engineered. For cotton and corn, the genetic engineering has involved the introduction of Bt genes into these crops to confer insect resistance. Thus, Bt serves as a paradigm for transgenic plants. A timeline (Figure 1) highlighting important events in the development of Bt as an insecticide is given.

FIGURE 1.
TIMELINE OF IMPORTANT EVENTS IN THE DEVELOPMENT OF Bt AS AN INSECTICIDE.
The unprecedented and rapid adoption of transgenic crops since 1996 has raised questions among the public, governmental regulatory agencies, environmental groups, and the scientific community about the potential effects of these crops on non-target organisms, human health, and non-engineered crops or relatives of crop species. The potential toxicity of pollen from Bt corn on non-target organisms such as the monarch butterfly, the prospect of transgenic plants attaining special allergenic or toxic properties, and possible consequences of gene flow on crop genetic diversity and weed hardiness are some of the safety issues that have received attention in recent years. Other concerns are societal, and encompass impacts of the technology on international trade policy and cultural values.
Twenty-five (25) scientists with expertise in various aspects of Bt, plant biology, entomology, microbiology, and ecology were convened for a 2–1/2 day colloquium to address objectively the scientific basis surrounding these and other concerns about the safety and environmental consequences of Bt crops, particularly as compared with alternatives, such as conventional Bt sprays and synthetic insecticides. This report summarizes the conclusions reached in their discussions.
Bt's Family Tree
Bt is a member of the genus Bacillus, a diverse group of gram-positive, aerobic, spore-forming bacteria consisting of more than 20 species that differ in their basic biological properties. In addition to Bt, the most important species are B. subtilis, a source of industrial enzymes; B. cereus; and B. anthracis, the causative agent of anthrax. Members of the genus Bacillus generally are considered soil bacteria, and Bt is common in terrestrial habitats, including soil, living and dead insects, insect feces, granaries, and on the surfaces of plants. Bt occurs in nature predominantly as spores that can disseminate widely throughout the environment. B. anthracis also survives as spores, but there is no evidence of toxin gene transfer from B. anthracis to B. thuringiensis or B. cereus.
B. thuringiensis, B. cereus, and B. anthracis are closely related. Until recently they were differentiated based on biochemical, nutritional, and serological analyses, on the presence in Bt of insecticidal parasporal crystals visible by microscopy (Figure 2), and on pathogenicity to mammals. Insecticidal activity and pathogenicity in animals depend, in part, on the presence in B. thuringiensis and B. anthracis of plasmids, but the plasmids in the two species differ functionally and genetically, and those present in B. anthracis have never been found to be transferred to other species of Bacillus.
Accumulated molecular evidence suggests that B. thuringiensis and B. cereus should be considered a single species. They have been distinguished by the presence in B. thuringiensis of parasporal crystals, but this is now considered too narrow a criterion for taxonomic purposes. A clearer understanding of the relationships among these organisms has begun to emerge, based on the application of high-resolution analyses of the genetic variability among large numbers of isolates. Isolates of B. thuringiensis and B. cereus are highly diverse, whereas very little genetic variation exists among isolates of B. anthracis. Thus, when isolates are grouped in a phylogenetic tree on the basis of genetic similarity, isolates of B. anthracis are tightly clustered. In contrast, isolates of B. thuringiensis and B. cereus form several different clusters that contain isolates from both species. Because isolates within a cluster are more similar to each other than to isolates in other clusters, these analyses indicate that B. anthracis is genetically distinct from B. thuringiensis and B. cereus. This emerging picture of genetic relationships is consistent with what is known of the ecology of these species: B. thuringiensis and B. cereus are indigenous to habitats in and around soil and insects, whereas B. anthracis is restricted to growth in animal hosts because of its obligately pathogenic lifestyle.
How Does Bt Work?
Various strains and insecticidal proteins
The diversity within B. thuringiensis is reflected in the fact that more than 60 serotypes and hundreds of different sub-species have been described. The two most widely used in commercial insecticides are B. thuringiensis subsp. kurstaki (Btk), which kills a wide range of lepidopteran species that are important pests in agriculture and forestry, and B. thuringiensis subsp. israelensis (Bti), used primarily for the control of mosquito and blackfly larvae.
Most of the insecticidal activity of Bt is due to intracellular crystal inclusions produced during the process of sporulation. These parasporal crystals (Figure 2) are comprised of one or more related ICPs encoded by cry (crystal) and cyt (cytolytic) genes located mainly on plasmids. Like B. thuringiensis itself, the cry and cyt genes are highly diverse; more than 30 types of Cry proteins and 8 types of Cyt proteins have been described, and over 100 genes have been cloned and sequenced. Target insect specificity is determined mainly by the ICPs, although B. thuringiensis also produces a variety of other enzymes and insecticidal proteins that may contribute to its virulence. One hundred and twenty six (126) Bt microbial insecticides are currently registered in the US, but these are based on only four subspecies of B. thuringiensis. Only five ICP genes have been engineered into commercial Bt crops, but many others are available for future use.
Susceptible organisms
Insecticides are generally sought that target high-impact agricultural pests, disease vectors, or nuisance pests. Whether applied as a commercial spray or deployed in crops, Bt is highly specific, with toxicity limited to only some species of one of the major groups of insects—typically lepidoptera (butterflies/moths), coleoptera (beetles), or diptera (flies/mosquitos). New Bt proteins are being discovered which are active against other orders of insects and other pests, such as mites and nematodes. The number of susceptible organisms is expanding to include virtually all invertebrate plant pests, as well as insects that transmit human and animal pathogens. Key agricultural pests currently targeted with Bt insecticides include bollworms, stem borers, bud-worms, and leafworms in field crops and grains; the gypsy moth and spruce budworm in forests; the cabbage looper and diamondback moth in vegetable crops; and certain insects with chewing mouthparts such as beetles. While Bt sprays are used on many crops, only cotton, corn, and potatoes expressing Bt ICPs are commercially available. Mosquitoes and blackflies targeted with Bt sprays and aquatic treatments include the blackfly vectors of Onchocerca volvulus, the etiologic agent of river blindness; mosquito vectors of viral encephalitis and West Nile virus; and immature forms of many nuisance mosquito, blackfly, and midge species. Table 1 gives a sample list of organisms that are susceptible to Bt.
TABLE 1.
SOME AGRICULTURAL AND FORESTRY PESTS CONTROLLED BY Bt SPRAYS.
MECHANISMS OF ACTION AND RESISTANCE
Bt insecticides, whether in the form of a spray or a Bt crop, do not function on contact as most chemical insecticides do, but rather, as midgut toxins. They must be ingested by the target organism to be effective, and killing takes hours to days, longer than is required for synthetic insecticides.
In the case of Bt sprays, parasporal crystals ingested by insect larvae feeding on plant surfaces dissolve and the insecticidal proteins are activated by proteases in the juices of the midgut, which typically are akaline (pH 8–10.5). In Bt crops, the plant tissues produce specific ICPs in a soluble form. In either case, the active ICP then traverses the peritrophic membrane and binds to specific receptors on the midgut epithelium, forming pores and leading to loss of the trans-membrane potential, cell lysis, leakage of the midgut contents, paralysis, and death of the insect. ICP activation and pore formation is shown in Figure 3. One level of specificity in this interaction is provided by the midgut environment of the insect, and a second by the binding of ICPs only to membranes carrying the appropriately matched receptors. Insects that develop resistance to Bt most commonly exhibit decreased or altered receptor binding, although altered proteolytic activation also has been reported.
Stability, persistence, and uniformity of coverage are major factors in determining the probability that insects will develop resistance to Bt. When applied as a spray, Bt is relatively unstable; it can be washed off by rain or broken down by ultraviolet light and may need to be reapplied as frequently as every two to four days. This instability, along with variable, low-dosage residues on the plant may contribute to the emergence of resistant insect populations. These problems are avoided by higher and more uniform doses that can be obtained in Bt plants. In soil, the stability of ICPs is affected by microbial degradation. In general, the drier the soil, the longer an ICP will be stable, as there is less microbial degradation.
Current Uses of Bt
Sprays
Viable bacterial spores constitute the active ingredient in Bt spray formulations. Cry and Cyt ICPs are the primary cause of insect death. However, other components in sprayed Bt pesticides can contribute, especially in insects that are not very sensitive to Cry proteins. For example, the Bt spore is important to lethality to both gypsy moth larvae and to such pests as the beet armyworm and the cotton boll-worm. The Bt spore germinates after the gut is damaged and then begins vegetative growth, producing other insecticidal toxins and synergists. These include vegetative insecticidal proteins (VIPs), β-exotoxin, zwittermicin A, chitinases, and phospholipases.
Bt sprays comprise one to two percent of the global insecticide spray market, estimated to be U.S. $8 billion per annum. At one time, Bt sprays constituted $100 million in annual sales, but with the advent of transgenic plants engineered with ICP genes, sales have decreased to $40 million. Half of current sales are used in Canadian forests to control gypsy moths, spruce budworm, and other lepidopteran pests.
Bt sprays are used sporadically and typically over small areas. In tropical and subtropical areas (e.g., Hawaii), populations of diamondback moth have become resistant to Bt sprays following their intensive use. Crops sprayed with traditional Bt formulations include various vegetables, tree fruits, artichokes and berries. Sprays are chosen by organic farmers to meet guidelines for using strictly non-synthetic materials. An additional use of Bt is in the protection of stored commodities (e.g., wheat) from pest infestation.
Transgenic plants
Approximately 12 million hectares of insect-protected transgenic crops incorporating Bt ICPs are now planted annually worldwide. The vast majority of these crops are grown in the United States. Their total acreage has increased tenfold since 1996 and is expected to continue to increase along with other transgenic crops. In 2001 the number of farmers planting genetically engineered crops is expected to have exceeded 5 million, and global areas planted in transgenic crops are expected to have increased by 10% or more since 2000. In March of 2002, the Indian government announced that it would join the ranks of nations allowing commercial use of genetically engineered crops by licensing Bt cotton.
Bt crops currently are engineered to produce a single ICP continuously throughout all parts of the plant. The ICP gene is placed under the regulation of a promoter that is highly active in plants, and engineered so that codon preference is optimized for expression in plants. The most widely deployed transgenic crops expressing Bt ICP genes are Bt corn, which is grown in the U.S., China, Argentina, Canada, South Africa, Spain, Germany, and France; and Bt cotton, grown in the U.S., China, Australia, Argentina, South Africa, and Mexico. Many additional Bt crops are close to release, including rice, canola, and various vegetables and fruits.
Evaluation of Bt Technologies
Comparing Bt sprays and transgenic crops
There are advantages and disadvantages to the use of Bt in spray form. Like chemical pesticides, the timing, dosage, and formulation of the application can be controlled in any growing season to meet specific pest pressures. The drawbacks are that spray can drift during application, cannot be applied uniformly to all parts of the plant, and cannot be delivered to pests that are inside plant tissues. Further, insecticidal crystals and spores of Bt exposed on plant surfaces are highly sensitive to degradation by UV light and removal by water runoff. Multiple applications are therefore required to provide extended pest protection.
Bt transgenic crops also have strengths and weaknesses. ICPs are present at high concentrations in most or all tissues in current transgenic plants. This feature eliminates difficulties in targeting pests that burrow into plant tissues, as well as the labor and expenses associated with applying sprays. A potential limitation of engineered plants is that the ICP specificity cannot be changed within a growing season if resistance begins to develop. Engineered plants also currently lack the components found in bacterial formulations that act synergistically with the ICP to kill partially resistant insects. In the event that a sexually compatible relative is near a cultivated Bt crop, the risk that the Bt transgene could be transferred via pollen also is an issue of concern.
Differences between Bt and other pest control methods
Bt technologies—sprays or transgenic plants—will not control all insect pests. As with any pesticide, the potential exists for target insects to develop resistance. Both Bt sprays and Bt crops can only harm close relatives of the target pest, and only if eaten. The primary alternatives to Bt insecticides are synthetic chemical pesticides with much broader toxicity, impacting many non-target organisms including beneficial insects, fish, birds, and human beings. In the future, existing chemical pesticides, as well as new chemicals coming to market, will continue to provide alternatives to Bt. Although registered based on extensive safety testing, these chemicals are viewed as being less environmentally benign than Bt microbial insecticides and Bt crops.
Farm workers have benefited from the use of Bt sprays and Bt plants in place of hazardous insecticides. The high specificity of Bt ICPs not only leaves humans and other animals unaffected, but also makes these proteins harmless to the wide array of insects outside of their target range. A testament to its safety is that Bt is the only insecticide for which there are no mandated tolerance limits for residue in food. Bt transgenic plants more frequently displace synthetic insecticides than Bt sprays. Both uses of Bt can contribute to agriculture.
Insect pest management strategies that are available to agriculture need not be considered strictly as alternatives to Bt. Biocontrol through insect parasitoids or fungal pathogens, mating disruption, crop rotation, adjustments in date of planting, polyculture, and conventional chemical sprays are all compatible with Bt use. In particular, indigenous insect populations and introduced biocontrol agents that are harmed by broad spectrum chemical pesticides are not generally harmed by Bt. Thus, Bt is well-suited to Integrated Pest Management programs that preserve large segments of indigenous invertebrate predator and parasitoid populations.
Bt crops can significantly reduce the use of synthetic insecticides and are highly cost-effective in controlling economically important pests compared to existing insecticides. Bt crops provide ease of management by reducing the need for repeated pesticide applications, the labor costs for scouting to determine when to spray, the energy and equipment costs for applications, and the danger of pesticide exposure to farm workers. Conventional cotton is the most pesticide-intensive crop in the U.S., requiring upwards of 10 treatments per season. In Arizona, the deployment of Bt cotton has reduced chemical insecticide applications from 12 or 14 treatments to 2 to 6 treatments per season, with the number of applications depending on the growing region and the season. In 1999, Bt cotton in the U.S. resulted in a reduction of 1,200 metric tons of active ingredient of insecticides, and in China, a reduction of 15,000 tons of formulated insecticide. From 1996 to 2000, U.S. farmers have saved $100 million annually when reduced pesticide costs and increased yields are weighed against the added purchase cost of Bt cottonseed. A further benefit of Bt crops is reduction of certain food-contaminating toxins such as the fungal toxin fumonisin in corn.
Environmental Considerations
Despite concerns about the use of Bt sprays and Bt transgenic crops, both technologies have advantages over standard chemical insecticides. Unlike some insecticides that accumulate in biological systems, there are no data that Bt proteins do so. Bt sprays and Bt crops only kill the target pests and some closely related species, and only after ingestion of the Bt spray or plant. Bt cotton that targets the budworm and bollworm kills only these pests and related Lepidoptera when the plant is eaten. Bt corn is currently under development to control both the European corn borer and the corn rootworm. Corn rootworm costs growers sums in excess of $2 billion annually in crop losses and insecticide expenditures. Most chemical insecticides have a relatively broad range of activity against nontarget organisms including spiders, insect parasitoids and predators, as well as fish, birds, and humans. Lorsban®, now used to control corn rootworm, is broadly toxic to invertebrates and presents ecological and health concerns. However, Bt corn that targets both corn borer and corn rootworm will eliminate these risks to farm workers and the environment.
Impacts on Non-target Organisms
Bt has a long history of use in spray formulations and is generally considered a safe insecticide because it is highly specific. It directly affects only target organisms and closely related species, although the biotic community may be affected indirectly because of perturbations in insect populations. Populations of non-target insect species belonging to the same order as the target pest may be vulnerable. Initial concerns about the negative effects of pollen from Bt corn on the larvae of the monarch butterfly, Danaus plexippus, have been allayed by additional laboratory and field studies.
Field studies of transgenic crops have failed to demonstrate significant detrimental impacts of Bt on related nontarget organisms or their predators. Because chemical insecticides are generally used less frequently on Bt crops, it is likely that such crops will benefit non-target populations, especially those of parasitoids and predators that control insect pests. In fact, studies in the U.S., China, and elsewhere have documented larger populations of predatory bugs, spiders, and ants, and enhanced biodiversity of beneficial insects in Bt cotton fields as compared with conventional fields treated with chemical insecticides. Similar results have been reported in Bt versus sprayed conventional potato fields. Studies examining the impact of Bt formulations in aquatic environments have failed to show adverse effects. Similarly, field studies of forest applications of Bt sprays have shown that the impact is restricted to moths and butterflies and appears to be transient for many non-target organisms. Laboratory tests also have failed to show toxicity of Bt to birds, fish, and invertebrates, including earthworms.
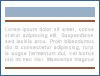
Box
The Monarch Butterfly, An Issue?
Toxicity tests on a standard set of organisms including avian and aquatic species, beneficial insects, soil invertebrates, and mammals are required before pesticide registration, providing a basis for assessing potential toxicity to non-target species. Fact sheets summarizing results from the testing of commercial Bt crops can be found at: www.epa.gov/pesticides/biopesticides/factsheets.
Laboratory and field tests can provide information about an organism's susceptibility to Bt, the dose of Bt that is toxic, and the fate of Bt in the environment. However, these tests say little about the risks to non-target organisms. Risk assessment measures both the degree of toxicity and the level of exposure and attempts to determine what effects Bt will have on a population under the conditions in which exposure will occur. For example, Bt sprays affect some non-target lepidopterans in the laboratory, but often the time of spraying does not coincide with the presence of susceptible non-target species, reducing their exposure to Bt in the field.
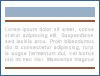
Box
Measuring Effects on Non-target Organisms.
Bt crops have only recently been deployed and until now relatively small sample sizes have been used in laboratory and field studies. Thus the data in support of the safety of Bt crops to non-target organisms is still limited. However, additional data are rapidly accumulating from field programs monitoring the actual impact of Bt use on ecosystems. Studies using large sample sizes of non-target organisms are underway to determine the potential of both short- and long-term effects of Bt crops. Initial results have failed to reveal significant non-target effects, in contrast to use of standard chemical pesticides.
Will soil-dwelling non-target organisms be affected by Bt ICPs? Laboratory studies have shown that actively growing Bt plants can increase the levels of ICPs in soil, and that soil can bind active Bt proteins for extended periods of time. However, there are no known toxicological effects of Bt ICPs on nontarget soil invertebrates or microbes. Root exu-dates from transgenic Bt corn appear to be nontoxic to earthworms, nematodes, protozoa, fungi, and bacteria. Because Bt is a natural inhabitant of soil, we also know that soil organisms have a long evolutionary association with Bt.
Resistance in Target Pests
Insects have the potential to develop resistance to insecticides. Based on laboratory selection experiments and models derived from these studies, the potential exists for development of resistance to Bt ICPs when these products are overused or used incorrectly. Several strategies are available for resistance management to Bt in spray formulations. These include the use of nontreated refuges, high dosage, mixtures of insecticidal toxins, and rotation or alternation of Bt toxins. After 40 years of use there is only one known example (the diamondback moth) of control failure due to resistance to Bt sprays having developed under field conditions, although other species have developed high levels of resistance through laboratory selections.
Because ICPs expressed in plants will see increasing use, concern that insect populations will develop resistance is justified. It is anticipated that resistance could arise if management strategies are not carefully followed, especially in crops based on single-gene technology. As with conventional pesticides, the evolution of resistance to ICPs will depend on the genetics of resistance, the competitiveness of resistant individuals in the field, and the implementation of resistance management strategies.
The resistance management program practiced most frequently for commercialized Bt crops is the “high dose/structured refuge strategy,” in which the crop produces an ICP at concentrations with a goal of more than 99.9% lethality in the target pest. High ICP concentrations in the tissues on which insects feed serve to counter incremental development of resistance. At the same time, from five to 50 percent (5–50%), depending on the crop, of the acreage is planted with a conventional version of the crop as a refuge for target insects. These conventional plants serve to sustain susceptible alleles within the insect population. Through random mating, rare recessive resistance genes are diluted out of the insect population and prevented from providing a selective advantage in offspring of the matings. This strategy will not be effective if cases develop where resistance is dominant.
Numerous studies have indicated that separate refuges are superior to seed mixtures in delaying the development of resistance in insects that can move between plants in the larval stage. Care must be taken in managing the insect population within the refuge to ensure that enough susceptible individuals will exist to breed out resistant individuals. The effectiveness of a refuge depends on consistent, appropriate implementation and monitoring. Grower compliance to a resistance management strategy is essential to delaying the development of resistance. Similarly, proper application of Bt sprays would help to defer the development of resistance resulting from overuse, but presently only Bt crops have mandated resistance management strategies.
New Bt crops that express multiple ICPs with different modes of action are currently being developed in a ‘gene stacking’ strategy. This technique should limit selection for resistance in target and secondary pest species because the probability of multiple, rare resistance mechanisms arising simultaneously is astronomically low.
Gene Flow to Other Organisms
Although it is theoretically possible for microorganisms to incorporate DNA from transgenic plants, this has only been accomplished under specifically optimized laboratory conditions and at a very low frequency. The probability that such transfers will occur in nature, and involve functional genes is even lower. There is no evidence that genes from non-engineered crops have been transferred to microorganisms and there is no basis to suggest that transgenes would transfer more readily than any other genes. Microbes are so much more likely to obtain genes, such as those for antibiotic resistance, from other microbes in the environment that the probability for such genes to be acquired from genetically engineered plant DNA is insignificant.
Gene transfer between closely related species of plants occurs via pollen under natural conditions all the time. Hybrids of conventional crops and wild relatives form in 12 of the 13 most common crops worldwide in some part of their agricultural range, and this process has been implicated in enhanced weediness about half of the time.
Gene flow of Bt ICP genes from Bt crops to wild relatives could occur where sexually compatible wild relatives are within the pollination range of the crop. Ecological consequences could result if the gene confers resistance to a pest that is a significant challenge to the wild relative. This might provide a selective advantage to the hybrid and its progeny through introgression of the gene into the wild relative's gene pool. Negative ecological impact—predicted on a case-by-case basis—should be weighed against impacts of the accepted alternative practices to Bt technology, such as use of conventional pesticides. In the U.S., the EPA has concluded that there is not a reasonable possibility of gene flow from Bt cotton, corn, or potatoes to wild relatives except in certain areas of Florida and Hawaii, where the use of cotton is restricted because of the presence of related wild species. There is a need for similar regulation globally, particularly in centers of crop origin, to insure that Bt crops are not grown in areas where potential outcrossing with wild or weedy relatives could introduce traits that might disturb biodiversity of cultivated crop species. Any gene flow that makes a weed more competitive in the wild will not be readily reversed in the way that use of some short-lived environmentally damaging chemical can be halted. There are a wide variety of methods to limit gene flow between related plant species (Table 2).
Human Health Considerations
Issues have been raised regarding consumer and worker safety of Bt use, whether applied as a foliar spray or expressed in plants. Food safety issues involve the potential toxicity and allergenicity of proteins, changes in the nutritional composition of plants, and the safety of the antibiotic resistance marker genes used during the process of genetic engineering. These issues are reviewed in a recent joint FAO/WHO publication. Bt proteins in all Bt plants and sprays registered for food consumption break down rapidly in simulated digestive systems, do not resemble any known food allergen or protein toxin and have no oral toxicity, even when administered in high doses.
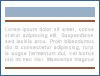
Box
The StarLink® Story.
In some cases, Bt crops can improve food safety. For example, approximately 59% of corn grain globally is contaminated with fumonisins, fungal toxins produced by the genus Fusarium that can cause liver and kidney damage in many species, and are probable human carcinogens. These fungal pathogens enter the plant through wounds caused by boring insects that are among the most important insect pests of corn worldwide. Bt corn is protected against damage from corn borers and consistently has 90% less fumonisin than conventional plants. Thus, protection against insect damage and subsequent fungal infection may have important health implications for consumers and farm animals exposed to fumonisins in their diet.
Bt sprays and transgenic crops have not had any known significant harmful effects on vertebrates, including mammals and human beings. There have been only a few reports of Bt bacteria being isolated from humans with wounds or infections, and in these rare cases there is no evidence that Bt was the cause of any lasting injury. It also has been shown that farm workers do not develop respiratory, cutaneous, or eye diseases from exposure to large amounts of Bt in sprays. They do develop antibodies to ICPs, but in no case has the presence of these antibodies been linked to acute or chronic disease. Thus, the use of Bt as a biological pesticide has proven to be remarkably safe for vertebrates, particularly in comparison with synthetic chemical insecticides. The health benefits to farm workers are clearly illustrated in a recent report indicating that farmers in China currently growing Bt cotton applied 80% less toxic insecticides than those growing conventional cotton varieties. The farmers growing Bt cotton also reported more than four-fold fewer instances of symptoms, such as headache, nausea, skin pain, or digestive problems from applying pesticides. Bt cotton is the GE crop most frequently planted by small farmers worldwide. Small farmers in developing countries are at particular risk of exposure to pesticides because they often apply pesticides by hand, without safety precautions, such as respirators, goggles, and gloves.
For the Future
Improving our knowledge
While transgenic crops are being adopted at unprecedented rates in large- and small-scale farming systems worldwide, the technology remains controversial. Debate has arisen on implications ranging from user, consumer, and environmental safety to global economics and world hunger. Many of the short- and long-term implications have been predicted based on past and ongoing experience, but much remains to be learned because the technology is new. Continued collaboration is therefore needed among scientists in academia, government, environmental groups, and industry to critically define, assess, and compare the risks and benefits of Bt sprays and crops relative to each other and to conventional pest control strategies. Scientific analyses should monitor actual on-farm practices, include conventional laboratory and field studies, and cover environmental impacts, effects on nontarget organisms and consumer safety. A thorough and objective evaluation of known and unresolved safety issues is essential to appropriate regulation of transgenic crops in relation to the security, sustainability, and economics of food production both on a regional scale and globally. The eventual worldwide acceptance of genetic engineering will depend not only on safety but also diverse cultural and societal norms.
With regard to Bt plants and formulations, many fundamental questions still require answers. For example, what is the baseline natural abundance and distribution of B. thuringiensis in the environment? When we breathe, how many Bt spores do we inhale? How many spores and crystals are consumed when we eat uncooked vegetables or organically grown crops sprayed with Bt? How does this compare with the amounts of ICPs ingested with Bt crops, and what is the relevance to consumer health? What factors influence the stability of insecticidal crystals and proteins introduced into soil in the form of sprays and plant exudates and residues, and under what conditions might persistence have biologically significant consequences?
Resistance of a pest to any insecticide is a serious concern. The U.S. Environmental Protection Agency requires specific insect resistance management programs for Bt corn, cotton, and potatoes, which include refugerequirements, annual resistance monitoring, and remedial action programs (http://www.epa.gov/pesticides/biopesticides/otherdocs/bt_brad2/4%20irm.pdf). These programs are not required for foliar sprays of Bt or other insecticides With increasing deployment of Bt crops, it is important to develop more sophisticated and reliable resistance monitoring and management strategies if sustained benefits are to be gained from Bt technology. The potential for resistance development in target and nontarget organisms needs to be better understood. What are the frequencies of resistance alleles and what are the best ways to detect them? Are they dominant, recessive, or polygenic? What are the mechanisms of action of ICPs at the molecular level and how do they influence target insect specificity and resistance? Do ICPs delivered in sprays and plants differ in their effects on specificity and resistance? Will increased deployment of Bt crops influence the rate of emergence of insect populations resistant to Bt sprays?
The need remains for a wide range of field studies to enable rigorous quantitative assessments of the direct and indirect effects of Bt sprays and crops on natural enemies and non-target organisms, especially as compared with treatments that include standard pesticide controls. Longer-term and more complex studies should evaluate possible impacts on important soil organisms. Additional information also is needed on the frequency with which pollen from Bt crops may fertilize other plants. In many cases, long-term environmental impact studies were not possible before Bt crops were commercialized because of the scale and duration over which monitoring is required. Agricultural systems are disruptive to the environment, so it is imperative that these effects be clearly distinguished from those specifically associated with Bt formulations and genetically engineered plants.

Research should continue to generate and evaluate Bt engineered crops that will benefit developing nations, such as the work being done by the International Rice Research Institute. Important contributions will also come from other international centers of the Consultative Group on International Agricultural Research that focus on wheat, corn, and potatoes. This research is enhanced by collaborations among scientists in academic, government, and international research foundation laboratories in both developing and developed countries. All phases of such projects—from design to field-testing and consideration of environmental issues—benefit from these collaborations.
Technology
Gene expression technology will play a critical role in the improvement of Bt products. ICP genes in transgenic plants can be genetically engineered for optimal efficacy and risk management. Refined engineering of genes may soon enable tissue-specific or inducible expression such that ICP proteins are synthesized only at sites in plants where insects are feeding.
For crops such as corn and cotton, in which the pollen does not inherit plastids, plastid transformation systems would prevent the dissemination of Bt transgenes through pollen. This also provides the opportunity to introduce unmodified bacterial genes instead of synthetic genes and high protein yields characteristic of plastid expression systems. Expression also can be engineered very specifically in the bacteria used in sprays by manipulating genetic elements that aid in synthesis of ICPs.

Protein engineering has the potential to make a significant contribution to Bt applications and insect control. For example, based on the current understanding of the structure and mode of action of ICPs, researchers have engineered proteins with increased toxicity to target insects. In the future, the same techniques can be used to narrow the host range, reducing the impact on non-target insects or biological control agents by increasing the specificity of pesticidal proteins. Genomic information of insects may suggest additional ways to improve the efficacy and specificity with which ICPs interact with their molecular targets. To augment the arsenal of insecticidal proteins, searches should continue for new varieties of ICPs and other types of insecticides of microbial origin.
Communication
Colloquium participants believe the scientific community could do a great deal more to encourage the expansion of government and university programs aimed at communicating to the general public the risks and benefits of using Bt insecticides and Bt crops. Scientists can work with their professional societies to present a balanced view of the risks and benefits of these new technologies. This can be done through white paper reports like this one, forums at scientific meetings, articles in newsletters, and being available to members of the press and the public. This report is available on the web site of the American Society for Microbiology (http://www.asmusa.org/acasrc/aca1.htm)
It is essential to foster public understanding that long-term environmental effects of Bt crops and products generally cannot be studied until after the products have been commercialized. Prior to the registration of the first Bt crop in 1995, the EPA evaluated studies of potential effects of Bt endotoxins in the environment. Based on their findings, they approved the use of Bt crops but realized that additional knowledge was needed over the longer term to assess the risks and benefits of this technology. Environmental effects cannot be conclusively studied in the laboratory; they must be studied in the field. This post-release analysis is essentially what is done to assess the environmental effects of traditional plant breeding and agricultural practices. The public should be reassured that the use and monitoring of transgenic crops continues to be more rigorously regulated by governmental agencies than any other agricultural technology.
The positive aspects and the risks of agricultural biotechnology should be clearly communicated to the public and compared with the benefits and risks of alternative technologies. Evidence collected to date indicates that genetic engineering of crops is at least as safe as conventional means of generating new crops. Many of the benefits of Bt crops, such as decreased production costs, reduced environmental impact, and lowered levels of contaminants in the food supply, are not easily recognized by the consumer. Alternative scenarios to the use of Bt crops should be presented, and the local, national, and international consequences of these scenarios should be considered in relation to food security, human health, and the environment.
Enhanced communication should be strongly encouraged among university and government researchers assessing the environmental effects of Bt crops worldwide. This is particularly important because Bt crops are grown in a variety of agroecosystems, the same insects are often a problem in different countries, and both insects and weedy species frequently span national borders. An international clearinghouse and database would facilitate information exchange pertaining to risks of emergence of resistance, nontarget effects, and potential gene flow to weeds and crops. In addition to its value to the scientific community, this centralized, science-based resource will help to build public confidence in genetic engineering by documenting the activities of ongoing research and monitoring programs.
Recommendations
Research
- ▪ Develop and maintain integrated international databases on Bacillus thuringiensis and ICPs.
- ▪ Continue support for culture collections of Bacillus thuringiensis isolates and strains of related species.
- ▪ Continue investigations into the persistence of ICPs and possible long-term effects on nontarget organisms and the environment.
- ▪ Evaluate and implement improved resistance management strategies.
- ▪ Evaluate gene flow to relatives of crops and improve strategies to manage it through cultural practices and engineered properties including the incorporation of Bt genes into the plastid genomes of engineered crop plants.
Communication
- ▪ Professional societies should help coordinate the dialogue between the international scientific community, the press, and the public.
- ▪ Scientists should participate in existing statewide outreach programs (such as cooperative extension services) that can disseminate information about the technology to the public and farming communities.
- ▪ A pamphlet for the public should be developed presenting objective information about Bt technologies.
- ▪ A web site on Bt should be developed as an educational tool for the public.
- ▪ An international database should be developed and maintained to centralize information concerning risk-benefit assessment issues associated with the global adoption of new technologies in agriculture.
References and Bibliography
- Aronson, AI, and Y Shai. 2001. Why Bacillus thuringiensis insecticidal toxins are so effective: unique features of their mode of action. FEMS Microbiol. Lett. 195:1–8. [PubMed: 11166987]
- Butler, L, C Zivkovich and BE Sample. 1995. Richness and abundance of arthropods in the oak canopy of West Virginia's Eastern Ridge and Valley Section during a study of impact of Bacillus thuringiensis with emphasis on macrolepidoptera larvae. Bull. Agr. For. Exp. Station West Virginia Univ. 711, 19pp.
- Cao, J, AM Shelton and ED Earle. 2001. Gene expression and insect resistance in transgenic broccoli containing a Bacillus thuringiensis cry1Ab gene with the chemically inducible PR-1a promoter. Molecular Breeding 8:207–216.
- Carpenter, JE, and LP Gianessi. 2001. Agricultural biotechnology: updated benefit estimates. January 2001. National Center for Food and Agricultural Policy, Washington, DC.
- Ellstrand, NC, HC Prentice and JF Hancock. 1999. Gene flow and introgression from domesticated plants into their wild relatives. Annu. Rev. Ecol. Systematics 30:539–563.
- Federici, BA. 1999. Bacillus thuringiensis in biological control, pp. 519–529. In Handbook of Biological Control. Eds. TS Bellows, G Gordh and TW Fisher. Academic Press, Inc. San Diego.
- Ferre, J, and J Van Rie. 2002. Biochemistry and genetics of insect resistance to Bacillus thuringiensis. Annu. Rev. Entomol. 47:501–533. [PubMed: 11729083]
- Frisvold, GB, R Transtad and JM Mortensen. 2000. Adoption of Bt cotton: regional differences in producer cost and returns. Proc. Beltwide Cotton Conf. 1:237–390.
- Glare, TR, and M O'Callaghan. 2000. Bacillus thuringiensis: Biology, Ecology and Safety. John Wiley & Sons, Ltd. UK. 350pp.
- Head, G, B Freeman, W Moar, J Ruberson and S Turnipseed. 2001. Natural enemy abundance in commercial bollgard and conventional cotton fields. In: Proc. of the Beltwide Cotton Conference, National Cotton Council, Memphis, TN.
- Hellmich, RL, BD Siegfried, MK Sears, DE Stanley-Horn, MJ Daniels, HR Mattila, T Spencer, KG Bidne and LC Lewis. 2001. Monarch larvae sensitivity to Bacillus thuringiensis-purified proteins and pollen. Proc. Natl. Acad. Sci. USA 98:11925–11930. [PMC free article: PMC59744] [PubMed: 11559841]
- Huang, J, S Rozelle, C Pray and Q Wang. 2002. Plant biotechnology in China. Science 295:674–677. [PubMed: 11809972]
- International Cotton Advisory Committee (ICAC) 2000. Report an expert panel on biotechnology in cotton. 1–17. (http://www
.icac.org/icac /meetings/plenary/59cairns/biotech .pdf) - Ishiwata, S. 1905. Concerning “Sotto-Kin” a bacillus of a disease of the silkworm. Rept. Assoc. Seric. Japan. Pp. 160–161.
- Jackson, P J, KK Hill, MT Laker, LO Ticknor and P Keim. 1999. Genetic comparison of Bacilllus anthracis and its close relatives using amplified fragment length polymorphism and polymerase chain reaction analysis. J. Appl. Microbiol. 87:263–269. [PubMed: 10475963]
- James, C. 2001. Global status of commercialized transgenic crops: 2000. ISAAA Briefs No. 23. ISAAA: Ithaca, NY.
- Losey, JE, LS Rayor and ME Carter. 1999. Transgenic pollen harms monarch larvae. Nature 399:214. [PubMed: 10353241]
- Maliga, P. 2002. Engineering the plastid genome of higher plants. Curr. Opin. Plant Biol. 5:164–172. [PubMed: 11856614]
- Miller, JC. 1990. Field assessment of the effects of a microbial pest control agent on non-target Lepidoptera. Amer. Entomol. 36:135–139.
- National Academy of Sciences. 2000. Genetically modified pest-protected plants: science and regulation. National Academy Press, Washington, DC. [PubMed: 25032472]
- National Agricultural Biotechnology Council. 2001. Genetically modified food and the consumer, NABC Report 13. Ithaca, NY.
- Nelson, GC, Ed. (2001). Genetically Modified Organisms in Agriculture: economics and politics. San Diego, Academic Press.
- Oberhauser, KS, MD Prysby, HR Mattila, DE Stanley-Horn, JK Sears, G Dively, E Olson, JM Pleasants, WF Lam and RL Hellmich. 2001. Temporal and spatial relationship between monarch larvae and corn pollen. Proc. Natl. Acad. Sci. USA 98:11913–11918. [PMC free article: PMC59742] [PubMed: 11559838]
- Pleasants, JM, RL Hellmich, GP Dively, MK Sears, DE Stanley-Horn, HR Mattila, JE Foster, TL Clark and GD Jones. 2001. Corn pollen deposition on milkweeds in and near cornfields. Proc. Natl. Acad. Sci. USA 98:11919–11924. [PMC free article: PMC59743] [PubMed: 11559840]
- Pray, CE, J Huang, D Ma and F Qiao. 2001. Impact of Bt cotton in China. World Development 29:813–825.
- Reed, GL, AS Jensen, J Riebe, G Head and JJ Duan. 2001. Transgenic Bt potato and conventional insecticides for Colorado potato beetle management: comparative efficacy and non-target impacts. Entomologia Experimentalis et Applicata.
- Roof, ME, and JA DuRant. 1997. On-farm experiences with Bt cotton in South Carolina, p. 861. In: Proc. of the Beltwide Cotton Conference, National Cotton Council, Memphis, TN.
- Saxena D, S Flores and G Stotzsky. 1999. Insecticidal toxin in root exudates from Bt corn. Nature 402:480. [PubMed: 10591205]
- Saxena, D, and G Stotzky. 2001. Bacillus thuringiensis (Bt) toxin released from root exudates and biomass of Bt corn has no apparent effect on earthworms, nematodes, protozoa, bacteria, and fungi in soil. Soil Biol. Biochem. 33:1225–1230.
- Schnepf, E, N Crickmore, J Van Rie, D Lereclus, J Baum, J Feitenson, DR Zeigler and DH Dean. 1998. Bacillus thuringiensis and its pesticidal crystal proteins. Microbiol. Mol. Biol. Rev. 62:775–806. [PMC free article: PMC98934] [PubMed: 9729609]
- Scott, SE, and MJ Wilkinson. 1999. Low probability of chloroplast movement from oilseed rape (Brassica napus) into wild Brassica rapa. Nat. Biotechnol.17:390–392. [PubMed: 10207890]
- Sears, MK, RL Hellmich, DE Stanley-Horn, KS Oberhauser, JM Pleaseants, HR Mattila, BD Siegfriedi and GP Dively 2001. Impact of Bt corn pollen on monarch butterfly populations: a risk assessment. Proc. Natl. Acad. Sci. USA 98:11937–11942. [PMC free article: PMC59819] [PubMed: 11559842]
- Shelton, AM, and RT Roush. 2000. Resistance to insect pathogens and strategies to manage resistance, pp. 829–846. In Field Manual of Techniques in Invertebrate Pathology. Eds. LA Lacey and HK Kaya. Kluwer Academic Press.
- Shelton, AM, JZ Zhao and RT Rousch. 2002. Economic, ecological, food safety, and social consequences of the deployment of Bt transgenic plants. Annu. Rev. Entomol. 47:845–881. [PubMed: 11729093]
- Stanley-Horn, DE, GP Dively, RL Hellmich, HR Mattila, MK Sears, R Rose, LCH Jesse, JE Losey, JJ Obrycki and L Lewis. 2001. Assessing the impact of Cry1Ab-expressing corn pollen on monarch butterfly larvae in field studies. Proc. Natl. Acad. Sci. USA 98:11931–11936. [PMC free article: PMC59745] [PubMed: 11559839]
- Ticknor, LO, A-B Kolstø, KK Hill, P Keim, MT Laker, M Tonks and PJ Jackson. 2001. Fluorescent amplified fragment length polymorphism analysis of Norwegian Bacillus cereus and Bacillus thuringiensis soil isolates. Appl. Environ. Microbiol. 67:4863–4873. [PMC free article: PMC93242] [PubMed: 11571195]
- US EPA. 2000. Off. Pestic. Programs, Biopesticides, and Pollut. Prev. Div. Biopesticides registration document; preliminary risks and benefits sections: Bacillus thuringiensis plant pesticides. U.S. Environmental Protection Agency, Washington, DC.
- US EPA. 2001. Off. Pestic. Programs, Biopesticides, and Pollut. Prev. Div. Biopesticides registration action document; revised risks and benefits sections: Bacillus thuringiensis Plant Pesticides. U.S. Environmental Protection Agency, Washington, DC.
- US EPA. 1995. Pesticide fact sheet for Bacillus thuringiensis subsp. kurstaki Cry1(A)b delta-endotoxin and the genetic material necessary for the production (plasmid vector pCIB4431) in corn. EPA publication number EPA731-F-95-004. U.S. Environmental Protection Agency, Washington, DC.
- Visconti, A. 2000. Fumonisin levels in corn and corn products – global perspectives. FDA Fumonisins Risk Assessment Workshop. January 10–12, 2000.
- Wagner, DL, JW Peacock, JL Carter and S E Talley. 1996. Field Assessment of Bacillus thuringiensis on non-target Lepidoptera. Environ. Entomol. 25:1444–1454.
- World Health Organization. 2000. Safety aspects of genetically modified foods of plant origin. Report joint FAO/WHO expert consultation on foods derived from biotechnology, Geneva, Switzerland, 29 May–2 June 2000. http://www
.who.int/fsf /GMfood/FAO-WHO_Consultation _report_2000.pdf - Zangerl, AR, D McKenna, CL Wraight, M Carrol, P Ficarello, R Warner, and MR Berenbaum. 2001. Effects of exposure to event 176 Bacillus thuringiensis pollen on monarch and black swallowtail caterpillars inder field conditions. Proc. Natl. Acad. Sci. USA 98:11908–11912. [PMC free article: PMC59741] [PubMed: 11559837]
- Zhao, JZ, Y Li, HL Collins and AM Shelton. 2002. Examination of the F2 screen for rare resistance alleles to Bacillus thuringiensis toxins in the diamondback moth. J. Econ. Entomol. 95:14–21. [PubMed: 11942749]
Footnotes
A more detailed bibliography for this report is available on line (http://www
.asmusa.org).
- NLM CatalogRelated NLM Catalog Entries
- Review Insect pathogens as biological control agents: Back to the future.[J Invertebr Pathol. 2015]Review Insect pathogens as biological control agents: Back to the future.Lacey LA, Grzywacz D, Shapiro-Ilan DI, Frutos R, Brownbridge M, Goettel MS. J Invertebr Pathol. 2015 Nov; 132:1-41. Epub 2015 Jul 27.
- Review Safety and advantages of Bacillus thuringiensis-protected plants to control insect pests.[Regul Toxicol Pharmacol. 2000]Review Safety and advantages of Bacillus thuringiensis-protected plants to control insect pests.Betz FS, Hammond BG, Fuchs RL. Regul Toxicol Pharmacol. 2000 Oct; 32(2):156-73.
- Review Bacillus thuringiensis (Bt) transgenic crop: an environment friendly insect-pest management strategy.[J Environ Biol. 2008]Review Bacillus thuringiensis (Bt) transgenic crop: an environment friendly insect-pest management strategy.Kumar S, Chandra A, Pandey KC. J Environ Biol. 2008 Sep; 29(5):641-53.
- Review The impact of secondary pests on Bacillus thuringiensis (Bt) crops.[Plant Biotechnol J. 2015]Review The impact of secondary pests on Bacillus thuringiensis (Bt) crops.Catarino R, Ceddia G, Areal FJ, Park J. Plant Biotechnol J. 2015 Jun; 13(5):601-12. Epub 2015 Mar 31.
- Review Economic, ecological, food safety, and social consequences of the deployment of bt transgenic plants.[Annu Rev Entomol. 2002]Review Economic, ecological, food safety, and social consequences of the deployment of bt transgenic plants.Shelton AM, Zhao JZ, Roush RT. Annu Rev Entomol. 2002; 47:845-81.
- 100 Years of Bacillus thuringiensis: A Critical Scientific Assessment100 Years of Bacillus thuringiensis: A Critical Scientific Assessment
Your browsing activity is empty.
Activity recording is turned off.
See more...