By agreement with the publisher, this book is accessible by the search feature, but cannot be browsed.
NCBI Bookshelf. A service of the National Library of Medicine, National Institutes of Health.
Kufe DW, Pollock RE, Weichselbaum RR, et al., editors. Holland-Frei Cancer Medicine. 6th edition. Hamilton (ON): BC Decker; 2003.
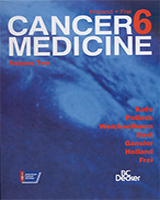
Holland-Frei Cancer Medicine. 6th edition.
Show detailsThe activation of oncogenes involves genetic changes to cellular protooncogenes. The consequence of these genetic alterations is to confer a growth advantage to the cell. Three genetic mechanisms activate oncogenes in human neoplasms: (1) mutation, (2) gene amplification, and (3) chromosome rearrangements. These mechanisms result in either an alteration of protooncogene structure or an increase in protooncogene expression (Figure 6-5). Because neoplasia is a multistep process, more than one of these mechanisms often contribute to the genesis of human tumors by altering a number of cancer-associated genes. Full expression of the neoplastic phenotype, including the capacity for metastasis, usually involves a combination of protooncogene activation and tumor suppressor gene loss or inactivation.

Figure 6-5
Schematic representation of the main mechanisms of oncogene activation (from protooncogenes to oncogenes). The normal gene (protooncogene) is depicted with its transcibed portion (rectangle). In the case of gene amplification, the latter can be duplicated (more...)
Mutation
Mutations activate protooncogenes through structural alterations in their encoded proteins. These alterations, which usually involve critical protein regulatory regions, often lead to the uncontrolled, continuous activity of the mutated protein. Various types of mutations, such as base substitutions, deletions, and insertions, are capable of activating protooncogenes.76 Retroviral oncogenes, for example, often have deletions that contribute to their activation. Examples include deletions in the aminoterminal ligand-binding domains of the erb B, kit, ros, met, and trk oncogenes.6 In human tumors, however, most characterized oncogene mutations are base substitutions (point mutations) that change a single amino acid within the protein.
Point mutations are frequently detected in the ras family of protooncogenes (K-ras, H-ras, and N-ras).77 It has been estimated that as many as 15% to 20% of unselected human tumors may contain a ras mutation. Mutations in K-ras predominate in carcinomas. Studies have found K-ras mutations in about 30% of lung adenocarcinomas, 50% of colon carcinomas, and 90% of carcinomas of the pancreas.78 N-ras mutations are preferentially found in hematologic malignancies, with up to a 25% incidence in acute myeloid leukemias and myelodysplastic syndromes.79,80 The majority of thyroid carcinomas have been found to have ras mutations distributed among K-ras, H-ras, and N-ras, without preference for a single ras family member but showing an association with the follicular type of differentiated thyroid carcinomas.81,82 The majority of ras mutations involve codon 12 of the gene, with a smaller number involving other regions such as codons 13 or 61.83 Ras mutations in human tumors have been linked to carcinogen exposure. The consequence of ras mutations is the constitutive activation of the signal-transducing function of the ras protein.
Another significant example of activating point mutations is represented by those affecting the ret protooncogene in multiple endocrine neoplasia type 2A syndrome (MEN2A).
Germline point mutations affecting one of the cysteines located in the juxtamembrane domain of the ret receptor have been found to confer an oncogenic potential to the latter as a consequence of the ligand-independent activation of the tyrosine kinase activity of the receptor. Experimental evidences have pointed out that these mutations involving cysteine residues promote ret homodimerization via the formation of intermolecular disulfide bonding, most likely as a result of an unpaired number of cysteine residues.84,85
Gene Amplification
Gene amplification refers to the expansion in copy number of a gene within the genome of a cell. Gene amplification was first discovered as a mechanism by which some tumor cell lines can acquire resistance to growth-inhibiting drugs.86 The process of gene amplification occurs through redundant replication of genomic DNA, often giving rise to karyotypic abnormalities called double-minute chromosomes (DMs) and homogeneous staining regions (HSRs).87 DMs are characteristic minichromosome structures without centromeres. HSRs are segments of chromosomes that lack the normal alternating pattern of light- and dark-staining bands. Both DMs and HSRs represent large regions of amplified genomic DNA containing up to several hundred copies of a gene. Amplification leads to the increased expression of genes, which in turn can confer a selective advantage for cell growth.
The frequent observation of DMs and HSRs in human tumors suggested that the amplification of specific protooncogenes may be a common occurrence in neoplasia.88 Studies then demonstrated that three protooncogene families-myc, erb B, and ras-are amplified in a significant number of human tumors (Table 6-2). About 20% to 30% of breast and ovarian cancers show c-myc amplification, and an approximately equal frequency of c-myc amplification is found in some types of squamous cell carcinomas.89 N-myc was discovered as a new member of the myc protooncogene family through its amplification in neuroblastomas.90 Amplification of N-myc correlates strongly with advanced tumor stage in neuroblastoma (Table 6-3), suggesting a role for this gene in tumor progression.91,92 L-myc was discovered through its amplification in small-cell carcinoma of the lung, a neuroendocrine-derived tumor.93 Amplification of erb B, the epidermal growth factor receptor, is found in up to 50% of glioblastomas and in 10% to 20% of squamous carcinomas of the head and neck.77 Approximately 15% to 30% of breast and ovarian cancers have amplification of the erbB-2 (HER-2/neu) gene. In breast cancer, erbB-2 amplification correlates with advanced stage and poor prognosis.94 Members of the ras gene family, including K-ras and N-ras, are sporadically amplified in various carcinomas.
Table 6-2
Oncogene Amplification in Human Cancers.
Table 6-3
Neuroblastoma.
Chromosomal Rearrangements
Recurring chromosomal rearrangements are often detected in hematologic malignancies as well as in some solid tumors.37,95,96 These rearrangements consist mainly of chromosomal translocations and, less frequently, chromosomal inversions. Chromosomal rearrangements can lead to hematologic malignancy via two different mechanisms: (1) the transcriptional activation of protooncogenes or (2) the creation of fusion genes. Transcriptional activation, sometimes referred to as gene activation, results from chromosomal rearrangements that move a proto-oncogene close to an immunoglobulin or T-cell receptor gene (see Figure 6-5). Transcription of the protooncogene then falls under control of regulatory elements from the immunoglobulin or T-cell receptor locus. This circumstance causes deregulation of protooncogene expression, which can then lead to neoplastic transformation of the cell.
Fusion genes can be created by chromosomal rearrangements when the chromosomal breakpoints fall within the loci of two different genes. The resultant juxtaposition of segments from two different genes gives rise to a composite structure consisting of the head of one gene and the tail of another. Fusion genes encode chimeric proteins with transforming activity. In general, both genes involved in the fusion contribute to the transforming potential of the chimeric oncoprotein. Mistakes in the physiologic rearrangement of immunoglobulin or T-cell receptor genes are thought to give rise to many of the recurring chromosomal rearrangements found in hematologic malignancy.97 Examples of molecularly characterized chromosomal rearrangements in hematologic and solid malignancies are given in Table 6-4. In some cases, the same protooncogene is involved in several different translocations (ie, c-myc, ews, and ret).
Table 6-4
Molecularly Characterized Chromosome Rearrangements in Tumors.
Gene Activation
The t(8;14)(q24;q32) translocation, found in about 85% of cases of Burkitt lymphoma, is a well-characterized example of the transcriptional activation of a proto-oncogene. This chromosomal rearrangement places the c-myc gene, located at chromosome band 8q24, under control of regulatory elements from the immunoglobulin heavy chain locus located at 14q32.98 The resulting transcriptional activation of c-myc, which encodes a nuclear protein involved in the regulation of cell proliferation, plays a critical role in the development of Burkitt lymphoma.99 The c-myc gene is also activated in some cases of Burkitt lymphoma by translocations involving immunoglobulin light-chain genes.100,101 These are t(2;8)(p12;q24), involving the κ locus located at 2p12, and t(8;22)(q24;q11), involving the κ locus at 22q11 (Figure 6-6). Although the position of the chromosomal breakpoints relative to the c-myc gene may vary considerably in individual cases of Burkitt lymphoma, the consequence of the translocations is the same: deregulation of c-myc expression, leading to uncontrolled cellular proliferation.

Figure 6-6
C-myc translocations found in Burkitt lymphoma. A, t(8;14)(q24;q32) translocation involving the locus of immunoglobulin heavy-chain gene located at 14q32. B, t(8;14)(q24;q32) translocation where only 2 exons (Ex) of c-myc are translocated under regulatory (more...)
In some cases of T cell acute lymphoblastic leukemia (T-ALL), the c-myc gene is activated by the t(8;14)(q24;q11) translocation. In these cases, transcription of c-myc is placed under the control of regulatory elements within the T-cell receptor α locus located at 14q11.102 In addition to c-myc, several protooncogenes that encode nuclear proteins are activated by various chromosomal translocations in T-ALL involving the T-cell receptor α or β locus. These include HOX11, TAL1, TAL2, and RBTN1/Tgt1.103–105 The proteins encoded by these genes are thought to function as transcription factors through DNA-binding and protein-protein interactions. Overexpression or inappropriate expression of these proteins in T cells is thought to inhibit T-cell differentiation and lead to uncontrolled cellular proliferation.
A number of other protooncogenes are also activated by chromosomal translocations in leukemia and lymphoma. In most follicular lymphomas and some large cell lymphomas, the bcl-2 gene (located at 18q21) is activated as a consequence of t(14;18)(q32;q21) translocations.72,73 Overexpression of the bcl-2 protein inhibits apoptosis, leading to an imbalance between lymphocyte proliferation and programmed cell death.74 Mantle cell lymphomas are characterized by the t(11;14)(q13;q32) translocation, which activates the cyclin d1 (bcl-1) gene located at 11q13.106,107 Cyclin D1 is a G1 cyclin involved in the normal regulation of the cell cycle. In some cases of T cell chronic lymphocytic leukemia and prolymphocytic leukemia, the tcl-1 gene at 14q32.1 is activated by inversion or translocation involving chromosome 14.108 The tcl-1 gene product is a small cytoplasmic protein whose function is not yet known.
Gene Fusion
The first example of gene fusion was discovered through the cloning of the breakpoint of the Philadelphia chromosome in chronic myelogenous leukemia (CML).109 The t(9;22)(q34;q11) translocation in CML fuses the c-abl gene, normally located at 9q34, with the bcr gene at 22q11 (Figure 6-7).110 The bcr/abl fusion, created on the der(22) chromosome, encodes a chimeric protein of 210 kDa, with increased tyrosine kinase activity and abnormal cellular localization.111 The precise mechanism by which the bcr/abl fusion protein contributes to the expansion of the neoplastic myeloid clone is not yet known. The t(9;22) translocation is also found in up to 20% of cases of acute lymphoblastic leukemia (ALL). In these cases, the breakpoint in the bcr gene differs somewhat from that found in CML, resulting in a 185 kDa bcr/abl fusion protein.112 It is unclear at this time why the slightly smaller bcr/abl fusion protein leads to such a large difference in neoplastic phenotype.

Figure 6-7
Gene fusion. The t(9;22)(q34;q11) translocation in chronic myelogenous leukemia (CML) determines the fusion of the c-abl gene with the bcr gene. Such a gene fusion encodes an oncogenic chimeric protein of 210 kDa. Chr = chromosome.
In addition to c-abl, two other genes encoding tyrosine kinases are involved in distinct gene fusion events in hematologic malignancy. The t(2;5)(p23;q35) translocation in anaplastic large cell lymphomas fuses the NPM gene (5q35) with the ALK gene (2p23).113 ALK encodes a membranespanning tyrosine kinase similar to members of the insulin growth factor receptor family. The NPM protein is a nucleolar phosphoprotein involved in ribosome assembly. The NPM/ALK fusion creates a chimeric oncoprotein in which the ALK tyrosine kinase activity may be constitutively activated. The t(5;12)(q33;p13) translocation, characterized in a case of chronic myelomonocytic leukemia, fuses the tel gene (12p13) with the tyrosine kinase domain of the PDGF receptor b gene (PDGFR-b at 5q33).114 The tel gene is thought to encode a nuclear DNA-binding protein similar to those of the ets family of protooncogenes.
Gene fusions sometimes lead to the formation of chimeric transcription factors.68,95 The t(1;19)(q23;p13) translocation, found in childhood pre-B-cell ALL, fuses the E2A transcription factor gene (19p13) with the PBX1 homeodomain gene (1q23).115 The E2A/PBX1 fusion protein consists of the amino-terminal transactivation domain of the E2A protein and the DNA-binding homeodomain of the PBX1 protein. The t(15;17)(q22;q21) translocation in acute promyelocytic leukemia (PML) fuses the PML gene (15q22) with the RARA gene at 17q21.116 The PML protein contains a zinc-binding domain called a RING finger that may be involved in protein-protein interactions. RARA encodes the retinoic acid alpha-receptor protein, a member of the nuclear steroid/thyroid hormone receptor superfamily. Although retinoic acid binding is retained in the fusion protein, the PML/RARA fusion protein may confer altered DNA-binding specificity to the RARA ligand complex.117 Leukemia patients with the PML/RARA gene fusion respond well to retinoid treatment. In these cases, treatment with all-trans retinoic acid induces differentiation of PML cells.
The ALL1 gene, located at chromosome band 11q23, is involved in approximately 5% to 10% of acute leukemia cases overall in children and adults.118,119 These include cases of ALL, acute myeloid leukemia, and leukemias of mixed cell lineage. Among leukemia genes, ALL1 (also called MLL and HRX) is unique because it participates in fusions with a large number of different partner genes on the various chromosomes. Over 20 different reciprocal translocations involving the ALL1 gene at 11q23 have been reported, the most common of which are those involving chromosomes 4, 6, 9, and 19.120 In approximately 5% of cases of acute leukemia in adults, the ALL1 gene is fused with a portion of itself.121 This special type of gene fusion is called self-fusion.122 Self-fusion of the ALL1 gene, which is thought to occur through a somatic recombination mechanism, is found in high incidence in acute leukemias with trisomy 11 as a sole cytogenetic abnormality. The ALL1 gene encodes a large protein with DNA-binding motifs, a transactivation domain, and a region with homology to the Drosophila trithorax protein (a regulator of homeotic gene expression).123,124 The various partners in ALL1 fusions encode a diverse group of proteins, some of which appear to be nuclear proteins with DNA-binding motifs.125,126 The ALL1 fusion protein consists of the aminoterminus of ALL1 and the carboxyl terminus of one of a variety of fusion partners. It appears that the critical feature in all ALL1 fusions, including self-fusion, is the uncoupling of the ALL1 amino-terminal domains from the remainder of the ALL1 protein.
Solid tumors, especially sarcomas, sometimes have consistent chromosomal translocations that correlate with specific histologic types of tumors.127 In general, translocations in solid tumors result in gene fusions that encode chimeric oncoproteins. Studies thus far indicate that in sarcomas, the majority of genes fused by translocations encode transcription factors.128 In myxoid liposarcomas, the t(12;16)(q13;p11) fuses the FUS (TLS) gene at 16p11 with the CHOP gene at 12q13.129 The FUS protein contains a transactivation domain that is contributed to the FUS/CHOP fusion protein. The CHOP protein, which is a dominant inhibitor of transcription, contributes a protein-binding domain and a presumptive DNA-binding domain to the fusion. Despite knowledge of these structural features, the mechanism of action of the FUS/CHOP oncoprotein is not yet known. In Ewing sarcoma, the t(11;22)(q24;q12) fuses the EWS gene at 22q12 with the FLI1 gene at 11q24.130 Like FUS, the EWS protein contains three glycine-rich segments and an RNA-binding domain. The FLI1 protein contains an ets-like DNA-binding domain. The EWS/FLI1 fusion protein combines a transactivation domain from EWS with the DNA-binding domain of FLI1. In alveolar rhabdomyosarcoma, the t(2;13)(q35;q14) fuses the PAX3 gene at 2q35 with the FKHR gene at 13q14.131 The PAX3 protein, a transcription factor that activates genes involved in development, is a paired-box homeodomain protein with two distinct DNA-binding domains. The FKHR protein encodes a conserved DNA-binding motif (the forkhead domain) similar to that first identified in the Drosophila forkhead homeotic gene. The PAX3/FKHR fusion protein is a chimeric transcription factor containing the PAX3 DNA-binding domains, a truncated forkhead domain, and the carboxy-terminal FKHR regions.
In DP, an infiltrating skin tumor, both a reciprocal translocation t(17;22)(q22;q13) and supernumerary ring chromosomes derived from the t(17;22) have been described.
Although early successful studies in this field have been performed with lymphomas and leukemia, as we have discussed before, the first chromosomal abnormality in solid tumors to be characterized at the molecular level as a fusion protein was an inversion of chromosome 10 found in papillary thyroid carcinomas.132 In this tumor, two main recurrent structural changes have been described, including inv(10) (q112.2; q21.2), as the more frequent alteration, and a t(10;17)(q11.2;q23). These two abnormalities represent the cytogenetic mechanisms which activate the protooncogene ret on chromosome 10, forming the oncogenes RET/ptc1 and RET/ptc2, respectively. Alterations of chromosome 1 in the same tumor type have then been associated to the activation of NTRK1 (chromosome 1), an NGF receptor which, like RET, forms chimeric fusion oncogenic proteins in papillary thyroid carcinomas.133 A comparative analysis of the oncogenes originated from the activation of these two tyrosine kinase receptors has allowed the identification and characterization of common cytogenetic and molecular mechanisms of their activation. In all cases, chromosomal rearrangements fuse the tK portion of the two receptors to the 5′ end of different genes that, due to their general effect, have been designated as activating genes. In the majority of cases, the latter belong to the same chromosome where the related receptor is located, 10 for RET and 1 for NTRK1.
Furthermore, although functionally different, the various activating genes share the following three properties: (1) they are ubiquitously expressed; (2) they display domains demonstrated or predicted to be able to form dimers or multimers; (3) they translocate the tK-receptor-associated enzymatic activity from the membrane to the cytoplasm.
These characteristics can explain the mechanism(s) of oncogenic activation of ret and NTRK1 protooncogenes. In fact, following the fusion of their tK domain to activating gene, several things happen: (1) ret and NTRK1, whose tissue-specific expression is restricted to subsets of neural cells, become expressed in the epithelial thyroid cells; (2) their dimerization triggers a constitutive, ligand-independent transautophosphorylation of the cytoplasmic domains and as a consequence, the latter can recruit SH2 and SH3 containing cytoplasmic effector proteins, such as Shc and Grb2 or phospholipase C (PLCγ), thus inducing a constitutive mitogenic pathway; (3) the relocalization in the cytoplasm of ret and NTRK1 enzymatic activity could allow their interaction with unusual substrates, perhaps modifying their functional properties.
In conclusion, in PTCs, the oncogenic activation of ret and NTRK1 protooncogenes following chromosomal rearrangements occurring in breakpoint cluster regions of both protooncogenes could be defined as an ectopic, constitutive, and topologically abnormal expression of their associated enzymatic (tK) activity.134
- Mechanisms of oncogene activation - Holland-Frei Cancer MedicineMechanisms of oncogene activation - Holland-Frei Cancer Medicine
Your browsing activity is empty.
Activity recording is turned off.
See more...