From: The Internal Anatomy of the Brain
NCBI Bookshelf. A service of the National Library of Medicine, National Institutes of Health.
Purves D, Augustine GJ, Fitzpatrick D, et al., editors. Neuroscience. 2nd edition. Sunderland (MA): Sinauer Associates; 2001.
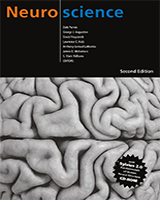
Neuroscience. 2nd edition.
Show detailsBox CFunctional Brain Imaging: PET, SPECT, and fMRI
The most informative brain imaging techniques for monitoring brain function now rely on detecting small changes in blood flow to visualize active areas of the brain. The brain utilizes a remarkably large fraction of the body's energy resources (about 20% of circulating glucose is consumed by the brain). Not surprisingly, at any given moment the most active nerve cells use more glucose and oxygen than relatively quiescent neurons. To meet the increased metabolic demands of particularly active neurons, the local flow of blood to the relevant brain area increases. Detecting and mapping these local changes in cerebral blood flow form the basis for three widely used functional brain imaging techniques: positron emission tomography (PET), single-photon emission computerized tomography (SPECT), and functional magnetic resonance imaging (fMRI). Because these techniques reveal patterns of activity in the intact brain, they have greatly enhanced the ability to understand both normal brain function and abnormal brain states associated with a variety of pathologies.
In PET scanning, unstable positron-emitting isotopes are synthesized in a cyclotron by bombarding nitrogen, carbon, oxygen, or fluorine with protons. Examples of the isotopes used include 15O (half-life, 2 min), 18F (110 min), and 11C (20 min). These probes can be incorporated into many different reagents (including water, precursor molecules of specific neurotransmitters, or glucose) and used to analyze specific aspects of brain function. When the radiolabeled compounds are injected into the bloodstream, they distribute according to the physiological state of the brain. Thus, labeled oxygen and glucose accumulate in more metabolically active areas, and labeled transmitter probes are taken up selectively by appropriate regions. As the unstable isotope decays, the extra proton breaks down into a neutron and a positron. The emitted positrons travel several millimeters, on average, until they collide with an electron. The collision of a positron with an electron destroys both particles, emitting two gamma rays from the site of the collision in directions that are exactly 180° apart. Gamma ray detectors placed around the head are therefore arranged to register a “hit” only when two detectors 180° apart react simultaneously. By reconstructing the sites of the positron-electron collisions, the location of active regions can be imaged. The mean free path of the positrons in brain tissue limits the resolution of PET scanning to about 4 mm. Nonetheless, PET images can be superimposed on MRI images from the same subject (see Box B), providing detailed information about specific brain areas involved in a wide variety of functions. The elegance and power of this technique are evident in Figures 24.6 and 25.6 in Unit V of this book.
SPECT imaging is an outgrowth of older techniques for measuring regional cerebral blood flow. A radiolabeled compound with a relatively short half-life (for example, 133Xe) is inhaled or injected into the circulation (in the latter case, 123I-labeled iodoamphetamine is used); the probes bind to red blood cells and are carried throughout the body. As the label undergoes radioactive decay, it emits high-energy photons. The rate of clearance of the probes was initially detected using an array of sodium iodide photon detectors placed around the head. More recent approaches have used a gamma camera that can be rapidly moved around the head to collect photons from many different angles, thus permitting a more accurate three-dimensional image. The information gathered using SPECT can also be combined with structural information from other imaging techniques, such as CT scans and MRI scans, to provide better localization of the active areas. A limitation of SPECT scanning is its relatively low resolution (about 8 mm). Although this level is not sufficient to resolve the finer features of the brain, it reveals the major areas involved in normal processing or disease. SPECT imaging is neither as flexible nor as accurate as PET imaging, but it is much simpler, primarily because the radiolabeled probes are commercially available and do not require an on-site cyclotron (as does the synthesis of PET probes).
A variant of MRI, called functional MRI (fMRI), now offers the best approach to analyzing the brain at work (see figure). fMRI is based on the fact that oxyhemoglobin (the oxygen-carrying form of hemoglobin) has a different magnetic resonance signal than deoxyhemoglobin (the oxygen-depleted form of hemoglobin) or the surrounding brain tissue. Brain areas activated by a specific task (e.g., the occipital cortex during visual behavior; see figure) utilize more oxygen. Initially, this activity decreases the levels of oxyhemoglobin and increases levels of deoxyhemoglobin. Within seconds, the brain microvasculature responds to this local oxygen depletion by increasing the flow of oxygen-rich blood to the active area. These changes in the concentration of oxyhemoglobin lead to localized blood oxygenation level dependent (BOLD) changes in the magnetic resonance signal, which form the basis for the fMRI signal. Thus, unlike PET or SPECT, fMRI uses signals intrinsic to the brain rather than signals originating from exogenous, radioactive probes; consequently, repeated observations can be made on the same individual, providing a major advantage over other imaging methods. fMRI also offers superior spatial localization (currently a few millimeters), as well as good temporal resolution (on the order of seconds or less under optimal circumstances, compared to minutes for other functional imaging techniques). As a result of these advantages, fMRI has emerged as the technology of choice for probing both the normal and abnormal functional architecture of the human brain.

Example of functional magnetic resonance imaging. Regional changes in cerebral blood flow were measured during visual stimulation; the area of activated visual cortex (color) was then mapped onto the brain, a section of which is shown at the appropriate level in the head. (From Belliveau et al., 1991.)
References
- Belliveau J. W. , 7 others Functional mapping of the human visual cortex by magnetic resonance imaging. Science. (1991);254:716–719. [PubMed: 1948051]
- Cohen M. S. , Bookheimer S. Y. Localization of brain function using magnetic resonance imaging. Trends Neurosci. (1994);17:268–277. [PubMed: 7524210]
- Kwong K. K. , 9 Others Dynamic magnetic resonance imaging of human brain activity during primary sensory stimulation. Proc. Natl. Acad. Sci. USA. (1992);89:5675–5679. [PMC free article: PMC49355] [PubMed: 1608978]
- Ogawa S. , 6 Others Intrinsic signal changes accompanying sensory stimulation: Functional brain mapping with magnetic resonance imaging. Proc. Natl. Acad. Sci. USA. (1992);89:5951–5955. [PMC free article: PMC402116] [PubMed: 1631079]
- Petersen S. E. , Fox P. T. , Snyder A. Z. , Raichle M. E. Activation of extrastriate and frontal cortical areas by visual words and word-like stimuli. Science. (1990);249:1041–1044. [PubMed: 2396097]
- Raichle M. E. Images of the mind: Studies with modern imaging techniques. Ann. Rev. Psychol. (1994);45:333–356. [PubMed: 8135505]
- Raichle, M. E. and M. I. Posner (1994) Images of Mind. New York: Scientific American Library.
- PubMedLinks to PubMed
- Box C, Functional Brain Imaging: PET, SPECT, and fMRI - NeuroscienceBox C, Functional Brain Imaging: PET, SPECT, and fMRI - Neuroscience
- Brevundimonas diminuta strain ATCC 19146 16S ribosomal RNA gene, partial sequenc...Brevundimonas diminuta strain ATCC 19146 16S ribosomal RNA gene, partial sequencegi|109809960|gb|DQ650706.1|Nucleotide
- Fusarium oxysporum strain ATCC MYA-4833 28S ribosomal RNA gene, complete sequenc...Fusarium oxysporum strain ATCC MYA-4833 28S ribosomal RNA gene, complete sequencegi|378925986|gb|JQ070149.1|Nucleotide
- Ochrobactrum anthropi partial 16S rRNA gene, isolate GH 1568Ochrobactrum anthropi partial 16S rRNA gene, isolate GH 1568gi|7242709|emb|AJ276036.1|Nucleotide
- Hypocrea virens strain ATCC MYA-4894 internal transcribed spacer 1, partial sequ...Hypocrea virens strain ATCC MYA-4894 internal transcribed spacer 1, partial sequence; 5.8S ribosomal RNA gene and internal transcribed spacer 2, complete sequence; and 28S ribosomal RNA gene, partial sequencegi|407971638|gb|JX174053.1|Nucleotide
Your browsing activity is empty.
Activity recording is turned off.
See more...